Highlights
- •
We combine biomechanical tests, μCT and histomorphometry to study a minipig model.
- •
We investigate osseointegration of titanium implants with innovative scaffolds.
- •
We measure means of IT values, RFA, BIC, BMD and stereological values of bone.
- •
We provide quantitative results on bone structure and mechanical properties.
Abstract
Objectives
To present a practical approach that combines biomechanical tests, microcomputed tomography (μCT) and histomorphometry, providing quantitative results on bone structure and mechanical properties in a minipig model, in order to investigate the specific response to an innovative dental biomaterial.
Methods
Titanium implants with innovative three-dimensional scaffolds were inserted in the tibias of 4 minipigs. Primary stability and osseointegration were investigated by means of insertion torque (IT) values, resonance frequency analysis (RFA), bone-to-implant contact (BIC), bone mineral density (BMD) and stereological measures of trabecular bone.
Results
A significant positive correlation was found between IT and RFA ( r = 0.980, p = 0.0001). BMD at the implant sites was 18% less than the reference values ( p = 0.0156). Peri-implant Tb.Th was 50% higher, while Tb.N was 50% lower than the reference zone ( p < 0.003) and they were negatively correlated ( r = −0.897, p = 0.006).
Significance
μCT increases evaluation throughput and offers the possibility for qualitative three-dimensional recording of the bone–implant system as well as for non-destructive evaluation of bone architecture and mineral density, in combination with conventional analysis methods. The proposed multimodal approach allows to improve accuracy and reproducibility for peri-implant bone measurements and could support future investigations.
1
Introduction
Improvements in bone–implant integration are crucial in modern dental surgery . The processes of osseointegration involve an initial mechanical interlocking between alveolar bone and the implant body (primary implant stability) and, later on, a biological fixation through continuous bone apposition (contact osteogenesis) and remodeling toward the implant (secondary implant stability). .
Biofidelity is a raising topic in modern medicine; such a concept derives from the study of the biomechanic of tissues and materials and of the biomimetic approach nowadays common in tissue engineering to imitate the properties of natural tissues with bioinspired materials. The bone tissue is as a natural nanostructured biomaterial containing a collagen matrix and hydroxyapatite filler . Tissue engineering using synthetic biomaterials is nowadays used in regenerative therapies and implantology, since they may act as bioactive scaffolds promoting wound healing and bone formation . The physico-chemical and structural characteristics of the scaffold materials have been observed to significantly influence their in vivo activity; 3D scaffolds may then represent new clinical applications in bone reconstructive surgery and implantology . In particular, it has been shown that scaffolds made up of porous hydroxyapatite may be colonized by osteoblasts favoring implant stability improvement .
Different methods were developed to objectively evaluate implant primary stability. In particular, peak insertion torque (IT) and resonance frequency analysis (RFA) are the parameters that are most globally used and a correlation between primary stability and implant insertion torque has been often suggested in dental literature . Insertion torque values were correlated to histological bone-to-implant contact and radiological bone density . RFA is another effective and reliable method to assess implant stability, which positively correlates with IT method .
The use of patients care newly developed implant materials often requires the use of preclinical models to test their biocompatibility, mechanical stability and safety. Before clinical trials in humans, minipigs are a recommended as animal model for translational research of new dental materials, since their bone structure is similar to that of humans. This species is considered to be closely representative of human bone tissue with regard to morphology , bone composition , microstructure and remodeling characteristics . Moreover, the bone of minipig shows similarities in mineral density and concentration with that of humans . However, minipigs bones have a denser trabecular network: mean cavity path lengths values in the adult man are typically 1200 μm and 350 μm in the minipig , while mean trabecular path lengths in child are typically 190 μm and 280 μm in the minipig . Bone regeneration rate in minipigs is comparable to that of humans (namely, 1.2–1.5 mm per day in minipigs and 1.0–1.5 mm per day in humans) , and cortical bone mineralization rate is similar to man , although mesenchymal stem cells in minipig showed a significantly lower ability than human ones to form differentiated and functional osteoblasts . Based on the above considerations, minipig has been selected in this study as large animal model for the pivotal preclinical testing of our innovative biomaterials and implants. This model would benefit from a non-destructive imaging modality so that mechanical and morphological endpoints can more readily be examined in the same specimens. Presently, microcomputed tomography (μCT) may represent a promising candidate tool for bone characterization when paired to biomechanical testing and standard histology. The implant stability which is critically dependent on structural characteristics and bone quality in a relatively broad area around the implant, may be correlated to bone properties assessed by μCT . This technique is fast, precise and, unlike conventional microscopic methods, does not need long time for specimen preparation and it is not impaired by the assessment of a limited number of sections. High-resolution μCT allows measurements of trabecular and cortical bone and a three-dimensional (3D) representation of bone formation at the peri-implant region . In accordance with the principles of the “refinement” and “reduction” declared by Russell and Burch in 1954, μCT allows less use of samples necessary for both biomechanical and morphometric studies. In the present investigation, an innovative hybrid nanocomposite working as a biomimetic scaffold was used to evaluate the performance of the novel material in improving implant primary stability in post-surgical sites.
Our innovative biomaterial appears as a nanoporous transparent glassy polymer ( Fig. 1 B ) able to progressively swell into a hydrogel form ( Fig. 1 C) when in contact with water solutions (picking up to 30–50% in weight of water), with a kinetic of swelling at 37 °C of about 0.1 mm per hour . The geometrical configuration of the modified implant ( Fig. 1 A) confines the polymeric scaffold in a niche that allows swelling deformation only in the radial implant direction. This radial guided swelling could be used to stabilize the implant in the socket while creating a biomechanically active interface for bone growth . Moreover, the nanoporosity can be modulated to host cells and growth factors. In previous investigations, the material was characterized for physical, chemical and mechanical properties . It showed a bioactive surface with improved osteoblastic adhesion and proliferation and it could be speculated that this feature could promote more effective and rapid osseointegration. Furthermore, the finite element analysis (FEA) was used to evaluate the scaffold interactions between the swollen interface and the bone . The elastic modulus of the scaffolds in the swollen state was reported to range between 2 and 10 MPa, which is comparable to that of the periodontal ligament (2–50 MPa) . The scaffold had to play two biomechanical functions: a structural one, as part of the fixture, and a bioactive one, as bone growth stimulus. Physiologically, the stresses and deformations induced by the stretching of the periodontal ligament acts as a biomechanical stimulus that favors new bone apposition in the tooth socket . Since the elastic modulus of the swollen scaffold was comparable to that of the periodontal ligament, the FEA confirmed that the swelling of the nanocomposite could act as a biomechanical bone growth input . According to Frost’s law regarding the strain dependent adaptive properties of bone , the strain values recorded at the bone-scaffold interface fell within the adapted window and were compatible with an organized bone growth. The purpose of this pilot study was to examine the utility of μCT for evaluating bone–implant integration in a minipig model, as an adjunct to conventional histomorphometry and biomechanical testing. The presented approach can serve as a model for future investigations to study implants of different composition or shape with complementary μCT analysis. The null hypothesis stated that there was no association between the biomechanical, histomorphometric and μCT study variables of control and experimental implant sites.

2
Materials and methods
2.1
Surgical procedures
All experimental procedures have complied with the Italian D.L. no 116 of 1992 and associated guidelines in the European Communities Council Directive of the 24th of November 1986 (86/609/ECC). Titanium implants (Winsix Implant System, Biosafin, Ancona, Italy) modified with 3D scaffolds were used.
The proposed biomaterial was a hydrophilic hybrid composite made up of nanoinclusions of hydroxyapatite dipped in a ceramo-polymeric matrix composed by poly-hydroxylethylmethacrylate and fumed nanosilica.
The scaffolds were designed using a reverse engineering approach and were retained by modified implant prototypes. Standard titanium implants with a diameter of 5.2 mm, a length of 13 mm and a hexagonal internal connection were used. An implant was imported in a solid modeler and meshed to discretize its geometry. Then, a finite element modeling was used to modify the fixture and create the experimental prototype, whose shape was suitable to incorporate the scaffold ( Fig. 1 A). The coronal part of the screw thread was removed so as to incorporate the scaffold, that had a length of 5 mm and the shape of a truncated cone with the major base apically (major base 0.6 mm, minor base 0.4 mm). The titanium implant prototypes had a micro-rough surface that was acid etched ( Fig. 1 A).
A steel cylinder was used as tray for the fabrication of the modified implants. A double impression technique was performed using putty and light silicon impression materials. First, the shape of a standard unmodified implant was impressed in the silicon to obtain the external profile shape mold. Subsequently, the modified titanium implant prototypes were inserted in the silicon molds by means of an implant holder and the unpolymerized novel material liquid composite resin was injected in the empty space between the external shape mold and the modified implant. The material was let to self-polymerize at room temperature for 15 min. Then, the implant threads were thoroughly polished by means of silicon rubbers. Although, the new biomaterial was designed as self-setting at room temperature, an additional thermal treatement at 80 °C was added to the fabrication procedures in order to favor complete polymerization and stabilization of the scaffolds in their highly rigid glassy state (with an elastic modulus comparable to that of the bone) before the surgical insertion ( Fig. 1 B). The scaffolded implants were then sterilized by means of gamma-rays. The scaffolded implants were introduced unicortically into the left and right proximal tibial metaphysis of 4 male Yucatan minipigs (>18-month old, average body weight 65 kg) with an inter-implant distance of 5–9 mm. The implants were inserted flush with the cortical bone, so as the scaffolds could interface with both the cortical and the spongy bone ( Fig. 2 ). This was made to evaluate if the different elasticity of the bony tissues could affect the swelling of the scaffolds. The animals were sedated by an intramuscular injection (10 mg/kg) of ketamine (Inoketam 1000 ® , Virbac S.r.l., Milan, Italy) and 0.5 mg/kg midazolam PHG (Hospira, Lake Forest, IL, USA). Anesthesia was induced by mask and, after endotracheal intubation, the minipigs were maintained in spontaneously breathing by inhalation of 3% isoflurane and oxigen. The surgical fields were shaved and disinfected with benzoxonium chloride (Citrosil ® , Manetty & Roberts, Milan, Italy). The tibias were exposed by skin incisions and fascial-periosteal flaps. The implant sites were prepared using drills with increasing diameter and a torque-controlled handpiece implant unit at a rotation speed of 25–30 RPM, with continuous external sterile saline irrigation to minimize bone damage caused by overheating. Thereafter, 4 implants were placed in each tibia, according to local anatomy and bone quality. A total of 28 scaffolded fixtures was inserted in 7 tibias; 1 tibia was not used because of unfavorable local anatomy. The insertion torque values were recorded using an electronic torque-control implant handpiece. The implant position was checked by means of radiographs and intensification of brilliance, in order to verify that the scaffolds were in the transition area between the cortical and the spongy bone. The skin and the fascia-periosteum were closed in separate layers with synthetic monofilament non absorbable polypropylene suture (Prolene ® 2-0, Ethicon, Somerville, NJ, USA) and a single absorbable suture (Vicryl ® 3-0, Ethicon, Somerville, NJ, USA), respectively. Perioperatively, the animals received enrofloxacin 2.5 mg/kg/12 h (Baytril ® , Bayer, Barmen, Germany) as antibiotic for 6 days and ketorolac 1 mg/kg/24 h as anti-inflammatory medication for 3 days. The animals were inspected after the first few postoperative days for signs of wound dehiscence or infection and, thereafter, weekly to assess general health. The animals did not show signs of physical impairments and were in stable condition throughout the study. After 8 weeks, animals were sacrificed and specimens containing the implants were fixed in 10% pH 7.0-buffered formalin.
2.2
Biomechanical analysis
Insertion torque values (N cm) were recorded during implant placement by means of a torque-controlled implant handpiece. Moreover, once implant placement was completed, a RFA was performed using a dedicated electronic device (Osstell ISQ, Osstell AB, Göteborg, Sweden). The values of resonance frequency were recorded as an implant stability quotient (ISQ) in order to allow a comparison of primary stability between different fixtures .
2.3
μCT and 3D reconstruction
The bone surrounding the implant scaffolds was examined using μCT (eXplore Locus, GE Healthcare, London, Canada). The detector of such μCT system was made up of 2 components, a scintillator and a CCD camera, which were connected with optical fibers. The scintillator is a thin screen of cesium iodide, that luminesces when exposed to X-rays. The camera behind it records the image that is projected onto the scintillator. The projection is saved in digital format. A total of 466 μCT slices, with a pixel size of 45 μm, were imaged at an X-ray energy level of 80 kVp and a current of 450 μA. Exposure time was 100 ms with a total scanning time of 11 min. Images were reconstructed using a modified Feldkamp algorithm and scaled into Hounsfield units (HU). A calibration standard, containing a compartment with 1073 mg/cm 3 SB3 (an epoxy based bone-mimicking material), was used for bone mineral density (BMD) calibration. All data were evaluated in MicroView, 2.1.2 (GE Healthcare, London, Canada). Datasets were resampled to reorient implants perpendicular to the cross-section plane. Before segmentation, threshold levels for bone, implant and scaffold were determined, based on visual inspection of the complete slices and on the gray-scale histogram. The upper and lower threshold levels for bone, implants and scaffold were determined in all 7 samples and did not overlap, allowing to make a clear distinction. An automatic segmentation technique, that utilizes the Otsu method, was used for calculate the value to be used as the threshold to extract the bone from the surrounding structures. The means were calculated and used for each sample. The inter- and intra-examiner variabilities within 1 standard deviation were calculated. Then, a cylindrical, volumetric region of interest (VOI) was placed in correspondence of the scaffold element of the implant circumferentially expanded 2.5 mm around the scaffold, including both cortical and cancellous bone ( Fig. 2 C), which was defined as the test zone ( Fig. 3 A ). Subsequently, bone, implant and scaffold in the ROI were differentiated based on their threshold levels. Outcome variables were: BMD (mg/cm 3 ) and bone mineral concentration (BMC, mg); bone volume fraction (BVF), being the % of the ROI which includes bone; bone volume (BV), being the mm 3 of bone that is present in the region of interest; the ratio of the volume of bone present (BV) to the total volume (TV) of interest (%); the ratio of the surface (BS) to the volume (BV) of bone (mm −1 ); the average trabecular thickness (Tb.Th, mm); the mean trabecular number (Tb.N, mm −1 ); the mean diameter to the marrow spaces (Tb.Sp, mm). These values were also measured in VOI at an equivalent distance between 2 median implants, as reference zone ( Fig. 3 B). 3-D volume rendering images were performed on 27 μm μCT dataset using Osirix Imaging Software 5.8.5 (Pixmeo, Bermex, Switzerland) ( Fig. 2 A, B, E, F).
2.4
Histology and histomorphometry
The formalin-fixed specimens were transferred to 70% ethanol solution, dehydrated in ascending concentration of ethanol up to 100%, and then infiltrated and embedded in a hydrophilic acrylic resin (LR White, London Resin Company, Berkshire, England). After polymerization, the specimens were sectioned along the longitudinal axis with a high-precision diamond disk at about 100 μm, provided with a custom-built sawing and a grinding apparatus (TT System, TMA2, Grottammare, Italy). A total of 3 sections was obtained for each implant (1 longitudinal central section and 2 sections at a cutting distance of 600 μm). The sections were ground down to about 60 ± 10 μm and stained with acid fuchsine and toluidine blue. The investigation was conducted in a transmitted brightfield and circularly polarized Light Microscope Axiolab (Zeiss Oberchen, Germany) connected to a high-resolution digital camera (FinePix S2 Pro, Fuji Photo Film Co. Ltd, Minato-Ku, Japan). A histomorphometric software package with image capturing capabilities (Image-Pro Plus 6.0, Media Cybernetics Inc, Bethesda, MD, USA) was used. To ensure accuracy, the software was calibrated for each experimental image using a feature named “Calibration Wizard”, which creates a linear remapping of the pixel numbers. The unit of measurement was the pixel. The analyzed parameter was the bone-to-implant contact (BIC, %), defined as the ratio between the length of the scaffold material section in contact with bone and the perimeter of the scaffold area, at 25× magnification. At each measurement, the software was calibrated using the length of the implant as reference. Measurements were performed by a single experienced and calibrated operator. Intra-examiner variability was controlled by carrying out 2 measurements; if the difference between the 2 values was greater than 5%, the measuring was repeated.
2.5
Statistical analyses
The study variables were divided into 4 groups as follows: biomechanical (IT and RFA), histomorphometric (BIC), μCT of implant sites (BMD, BMC, BVF, BV, BV/TV, BS/BV, TbTh, TbN, TbSp) and μCT of control sites (cBMD, cBMC, cBVF, cBV, cBV/TV, cBS/BV, cTb/Th, cTbN, cTbSp). Mean, standard deviation, median, minimum and maximum values were calculated for all the variables. Normality was tested using Shapiro–Wilk test and variance homogeneity using Bartlett or Levene test. When data were normally distributed and variance homogeneity was met, the variables were compared using one-way analysis of variance (ANOVA). In case of violation of normality or variance homogeneity, Kruskall–Wallis or Mann–Whitney test were performed. The mean values of 21 variables for 7 tibial samples were used for the statistical analysis. The Pearson correlation was performed for all possible pairs of variables, for a total of 210 combinations. The linear regression coefficients were calculated for the 12 pairs of variables found to be correlated. Moreover, IT, RFA, BIC, BMD, BMC, TbTh, TbN and TbSp were analyzed for comparison among implant sites. Sample size required to reach a 5% significance with a 80% power was estimated for Mann–Whitney, Shapiro–Wilk, Anova and Pearson correlation tests. Statistical analysis was performed using R statistical software (version 3.1.2, R Foundation for Statistical Computing, Vienna, Austria) and the level of significance was set at p < 0.05 for all tests.
2
Materials and methods
2.1
Surgical procedures
All experimental procedures have complied with the Italian D.L. no 116 of 1992 and associated guidelines in the European Communities Council Directive of the 24th of November 1986 (86/609/ECC). Titanium implants (Winsix Implant System, Biosafin, Ancona, Italy) modified with 3D scaffolds were used.
The proposed biomaterial was a hydrophilic hybrid composite made up of nanoinclusions of hydroxyapatite dipped in a ceramo-polymeric matrix composed by poly-hydroxylethylmethacrylate and fumed nanosilica.
The scaffolds were designed using a reverse engineering approach and were retained by modified implant prototypes. Standard titanium implants with a diameter of 5.2 mm, a length of 13 mm and a hexagonal internal connection were used. An implant was imported in a solid modeler and meshed to discretize its geometry. Then, a finite element modeling was used to modify the fixture and create the experimental prototype, whose shape was suitable to incorporate the scaffold ( Fig. 1 A). The coronal part of the screw thread was removed so as to incorporate the scaffold, that had a length of 5 mm and the shape of a truncated cone with the major base apically (major base 0.6 mm, minor base 0.4 mm). The titanium implant prototypes had a micro-rough surface that was acid etched ( Fig. 1 A).
A steel cylinder was used as tray for the fabrication of the modified implants. A double impression technique was performed using putty and light silicon impression materials. First, the shape of a standard unmodified implant was impressed in the silicon to obtain the external profile shape mold. Subsequently, the modified titanium implant prototypes were inserted in the silicon molds by means of an implant holder and the unpolymerized novel material liquid composite resin was injected in the empty space between the external shape mold and the modified implant. The material was let to self-polymerize at room temperature for 15 min. Then, the implant threads were thoroughly polished by means of silicon rubbers. Although, the new biomaterial was designed as self-setting at room temperature, an additional thermal treatement at 80 °C was added to the fabrication procedures in order to favor complete polymerization and stabilization of the scaffolds in their highly rigid glassy state (with an elastic modulus comparable to that of the bone) before the surgical insertion ( Fig. 1 B). The scaffolded implants were then sterilized by means of gamma-rays. The scaffolded implants were introduced unicortically into the left and right proximal tibial metaphysis of 4 male Yucatan minipigs (>18-month old, average body weight 65 kg) with an inter-implant distance of 5–9 mm. The implants were inserted flush with the cortical bone, so as the scaffolds could interface with both the cortical and the spongy bone ( Fig. 2 ). This was made to evaluate if the different elasticity of the bony tissues could affect the swelling of the scaffolds. The animals were sedated by an intramuscular injection (10 mg/kg) of ketamine (Inoketam 1000 ® , Virbac S.r.l., Milan, Italy) and 0.5 mg/kg midazolam PHG (Hospira, Lake Forest, IL, USA). Anesthesia was induced by mask and, after endotracheal intubation, the minipigs were maintained in spontaneously breathing by inhalation of 3% isoflurane and oxigen. The surgical fields were shaved and disinfected with benzoxonium chloride (Citrosil ® , Manetty & Roberts, Milan, Italy). The tibias were exposed by skin incisions and fascial-periosteal flaps. The implant sites were prepared using drills with increasing diameter and a torque-controlled handpiece implant unit at a rotation speed of 25–30 RPM, with continuous external sterile saline irrigation to minimize bone damage caused by overheating. Thereafter, 4 implants were placed in each tibia, according to local anatomy and bone quality. A total of 28 scaffolded fixtures was inserted in 7 tibias; 1 tibia was not used because of unfavorable local anatomy. The insertion torque values were recorded using an electronic torque-control implant handpiece. The implant position was checked by means of radiographs and intensification of brilliance, in order to verify that the scaffolds were in the transition area between the cortical and the spongy bone. The skin and the fascia-periosteum were closed in separate layers with synthetic monofilament non absorbable polypropylene suture (Prolene ® 2-0, Ethicon, Somerville, NJ, USA) and a single absorbable suture (Vicryl ® 3-0, Ethicon, Somerville, NJ, USA), respectively. Perioperatively, the animals received enrofloxacin 2.5 mg/kg/12 h (Baytril ® , Bayer, Barmen, Germany) as antibiotic for 6 days and ketorolac 1 mg/kg/24 h as anti-inflammatory medication for 3 days. The animals were inspected after the first few postoperative days for signs of wound dehiscence or infection and, thereafter, weekly to assess general health. The animals did not show signs of physical impairments and were in stable condition throughout the study. After 8 weeks, animals were sacrificed and specimens containing the implants were fixed in 10% pH 7.0-buffered formalin.
2.2
Biomechanical analysis
Insertion torque values (N cm) were recorded during implant placement by means of a torque-controlled implant handpiece. Moreover, once implant placement was completed, a RFA was performed using a dedicated electronic device (Osstell ISQ, Osstell AB, Göteborg, Sweden). The values of resonance frequency were recorded as an implant stability quotient (ISQ) in order to allow a comparison of primary stability between different fixtures .
2.3
μCT and 3D reconstruction
The bone surrounding the implant scaffolds was examined using μCT (eXplore Locus, GE Healthcare, London, Canada). The detector of such μCT system was made up of 2 components, a scintillator and a CCD camera, which were connected with optical fibers. The scintillator is a thin screen of cesium iodide, that luminesces when exposed to X-rays. The camera behind it records the image that is projected onto the scintillator. The projection is saved in digital format. A total of 466 μCT slices, with a pixel size of 45 μm, were imaged at an X-ray energy level of 80 kVp and a current of 450 μA. Exposure time was 100 ms with a total scanning time of 11 min. Images were reconstructed using a modified Feldkamp algorithm and scaled into Hounsfield units (HU). A calibration standard, containing a compartment with 1073 mg/cm 3 SB3 (an epoxy based bone-mimicking material), was used for bone mineral density (BMD) calibration. All data were evaluated in MicroView, 2.1.2 (GE Healthcare, London, Canada). Datasets were resampled to reorient implants perpendicular to the cross-section plane. Before segmentation, threshold levels for bone, implant and scaffold were determined, based on visual inspection of the complete slices and on the gray-scale histogram. The upper and lower threshold levels for bone, implants and scaffold were determined in all 7 samples and did not overlap, allowing to make a clear distinction. An automatic segmentation technique, that utilizes the Otsu method, was used for calculate the value to be used as the threshold to extract the bone from the surrounding structures. The means were calculated and used for each sample. The inter- and intra-examiner variabilities within 1 standard deviation were calculated. Then, a cylindrical, volumetric region of interest (VOI) was placed in correspondence of the scaffold element of the implant circumferentially expanded 2.5 mm around the scaffold, including both cortical and cancellous bone ( Fig. 2 C), which was defined as the test zone ( Fig. 3 A ). Subsequently, bone, implant and scaffold in the ROI were differentiated based on their threshold levels. Outcome variables were: BMD (mg/cm 3 ) and bone mineral concentration (BMC, mg); bone volume fraction (BVF), being the % of the ROI which includes bone; bone volume (BV), being the mm 3 of bone that is present in the region of interest; the ratio of the volume of bone present (BV) to the total volume (TV) of interest (%); the ratio of the surface (BS) to the volume (BV) of bone (mm −1 ); the average trabecular thickness (Tb.Th, mm); the mean trabecular number (Tb.N, mm −1 ); the mean diameter to the marrow spaces (Tb.Sp, mm). These values were also measured in VOI at an equivalent distance between 2 median implants, as reference zone ( Fig. 3 B). 3-D volume rendering images were performed on 27 μm μCT dataset using Osirix Imaging Software 5.8.5 (Pixmeo, Bermex, Switzerland) ( Fig. 2 A, B, E, F).
2.4
Histology and histomorphometry
The formalin-fixed specimens were transferred to 70% ethanol solution, dehydrated in ascending concentration of ethanol up to 100%, and then infiltrated and embedded in a hydrophilic acrylic resin (LR White, London Resin Company, Berkshire, England). After polymerization, the specimens were sectioned along the longitudinal axis with a high-precision diamond disk at about 100 μm, provided with a custom-built sawing and a grinding apparatus (TT System, TMA2, Grottammare, Italy). A total of 3 sections was obtained for each implant (1 longitudinal central section and 2 sections at a cutting distance of 600 μm). The sections were ground down to about 60 ± 10 μm and stained with acid fuchsine and toluidine blue. The investigation was conducted in a transmitted brightfield and circularly polarized Light Microscope Axiolab (Zeiss Oberchen, Germany) connected to a high-resolution digital camera (FinePix S2 Pro, Fuji Photo Film Co. Ltd, Minato-Ku, Japan). A histomorphometric software package with image capturing capabilities (Image-Pro Plus 6.0, Media Cybernetics Inc, Bethesda, MD, USA) was used. To ensure accuracy, the software was calibrated for each experimental image using a feature named “Calibration Wizard”, which creates a linear remapping of the pixel numbers. The unit of measurement was the pixel. The analyzed parameter was the bone-to-implant contact (BIC, %), defined as the ratio between the length of the scaffold material section in contact with bone and the perimeter of the scaffold area, at 25× magnification. At each measurement, the software was calibrated using the length of the implant as reference. Measurements were performed by a single experienced and calibrated operator. Intra-examiner variability was controlled by carrying out 2 measurements; if the difference between the 2 values was greater than 5%, the measuring was repeated.
2.5
Statistical analyses
The study variables were divided into 4 groups as follows: biomechanical (IT and RFA), histomorphometric (BIC), μCT of implant sites (BMD, BMC, BVF, BV, BV/TV, BS/BV, TbTh, TbN, TbSp) and μCT of control sites (cBMD, cBMC, cBVF, cBV, cBV/TV, cBS/BV, cTb/Th, cTbN, cTbSp). Mean, standard deviation, median, minimum and maximum values were calculated for all the variables. Normality was tested using Shapiro–Wilk test and variance homogeneity using Bartlett or Levene test. When data were normally distributed and variance homogeneity was met, the variables were compared using one-way analysis of variance (ANOVA). In case of violation of normality or variance homogeneity, Kruskall–Wallis or Mann–Whitney test were performed. The mean values of 21 variables for 7 tibial samples were used for the statistical analysis. The Pearson correlation was performed for all possible pairs of variables, for a total of 210 combinations. The linear regression coefficients were calculated for the 12 pairs of variables found to be correlated. Moreover, IT, RFA, BIC, BMD, BMC, TbTh, TbN and TbSp were analyzed for comparison among implant sites. Sample size required to reach a 5% significance with a 80% power was estimated for Mann–Whitney, Shapiro–Wilk, Anova and Pearson correlation tests. Statistical analysis was performed using R statistical software (version 3.1.2, R Foundation for Statistical Computing, Vienna, Austria) and the level of significance was set at p < 0.05 for all tests.
3
Results
The minipigs showed good generale health after surgery and signs of infection were not found at clinical and μCT examination. No effects from X-ray scattering or ring artifacts were found in the μCT images in the peri-implant zone used for measurements ( Fig. 4 ). All the implants were osseointegrated and the peri-implant bone structure consisted of lamellar bone architecture ( Fig. 5 ). The IT and RFA values were reported in Table 1 .
Minipig | Tibia | Measurement | Implant | |||
---|---|---|---|---|---|---|
#1 | #2 | #3 | #4 | |||
#1 | Left | IT (N cm) | 44.2 | 46.2 | 47.3 | 44.5 |
RFA (ISQ) | 71 | 73 | 74 | 71 | ||
Right | IT (N cm) | 48.1 | 44.6 | 45.1 | 44.4 | |
RFA (ISQ) | 75 | 71 | 72 | 71 | ||
#2 | Left | IT (N cm) | 43.4 | 44.7 | 42.9 | 42.5 |
RFA (ISQ) | 70 | 71 | 70 | 69 | ||
Right | IT (N cm) | 51.1 | 45.2 | 45.8 | 44.7 | |
RFA (ISQ) | 79 | 72 | 72 | 71 | ||
#3 | Left | IT (N cm) | Excluded because of unfavorable local anatomy | |||
RFA (ISQ) | ||||||
Right | IT (N cm) | 42.0 | 45.6 | 42.9 | 43.1 | |
RFA (ISQ) | 69 | 72 | 70 | 69 | ||
#4 | Left | IT (N cm) | 46.2 | 43.7 | 45.2 | 43.8 |
RFA (ISQ) | 73 | 70 | 72 | 70 | ||
Right | IT (N cm) | 42.6 | 39.9 | 43.2 | 42.2 | |
RFA (ISQ) | 69 | 66 | 70 | 69 |
According to the results of the present investigation, the null hypothesis was rejected.
Descriptive statistics for biomechanical, μCT and histologic variables were summarized in Table 2 . The mean and standard deviation of lower and upper threshold gray-levels for titanium implants, for ceramo-polymeric scaffolds and for bone made by 2 independent and calibrated operators were summarized in Table 3 . The first box of Table 2 shows the values of the 3 series of repeated measures for each bone sample; the second box those of all samples for each series of measurements; the third one the global threshold value for implant, scaffold and bone. The inter- and intra-examiner variabilities were equivalent within 1 standard deviation. ANOVA did not reveal differences among the implant sites for BIC ( F = 1.76, p = 0.112) and IT ( F = 2.580, p = 0.058), unlike RFA (Kruskall–Wallis, p = 0.0156). For μCT parameters, significant differences were found among the implant sites for BMC ( F = 5.201, p = 0.003) and BMD (Kruskall–Wallis, p = 0.037), unlike for TbTh ( F = 2.61, p = 0.056), TbN (Kruskall–Wallis, p = 0.037) and TbSp ( F = 1.764, p = 0.167). For comparisons between implant and control sites, ANOVA did not reveal differences between the μCT pairs of variables cBMC–BMC ( F = 1.26, p = 0.2831) and cTbSp–TbSp ( F = 8.68, p = 0.0514), whereas cBMD-BMD and cBV–BV were significantly different (Mann–Whitney test: p < 0.05). Fifty Pearson correlations were observed with p < 0.05, both between variables of the same group that between variables of different groups. The correlation matrix of all correlation coefficients between the set of variables were reported in Table 4 .
Variable | Unit | Mean | SD | Min | Median | Max |
---|---|---|---|---|---|---|
IT | N cm | 44.39 | 1.63 | 41.90 | 43.83 | 46.70 |
RFA | ISQ | 71.11 | 1.71 | 68.50 | 71.25 | 73.50 |
BMD | mg/cm 3 | 623.7 | 42.1 | 533.2 | 631.9 | 665.0 |
BMC | mg | 46.62 | 5.71 | 36.18 | 47.47 | 54.34 |
BVF | % | 0.7809 | 0.0639 | 0.6705 | 0.7925 | 0.8579 |
BV | mm 3 | 74.04 | 4.42 | 67.47 | 73.98 | 79.39 |
BV/TV | % | 0.8394 | 0.1118 | 0.7332 | 0.8164 | 1.0743 |
BS/BV | mm −1 | 1.641 | 0.182 | 1.451 | 1.634 | 1.891 |
TbTh | mm | 1.244 | 0.140 | 1.058 | 1.228 | 1.405 |
TbN | mm −1 | 0.6392 | 0.0335 | 0.5846 | 0.6316 | 0.6866 |
TbSp | mm | 0.3311 | 0.0744 | 0.2246 | 0.3165 | 0.4351 |
cBMD | mg/cm 3 | 764.0 | 31.5 | 719.9 | 765.5 | 808.9 |
cBMC | mg | 52.40 | 12.36 | 33.62 | 54.13 | 73.82 |
cBVF | % | 0.6955 | 0.0983 | 0.4933 | 0.7322 | 0.7799 |
cBV | mm 3 | 63.70 | 12.47 | 44.50 | 69.52 | 73.82 |
cBV/TV | % | 0.7075 | 0.1009 | 0.5043 | 0.7467 | 0.7913 |
cBS/BV | mm −1 | 3.722 | 1.307 | 1.923 | 4.117 | 5.517 |
cTbTh | mm | 0.6152 | 0.2675 | 0.3624 | 0.4857 | 1.0399 |
cTbN | mm −1 | 1.274 | 0.355 | 0.761 | 1.391 | 1.617 |
cTbSp | mm | 0.2407 | 0.0817 | 0.1507 | 0.2474 | 0.3561 |
BIC | % | 76.91 | 4.17 | 69.86 | 77.44 | 82.34 |
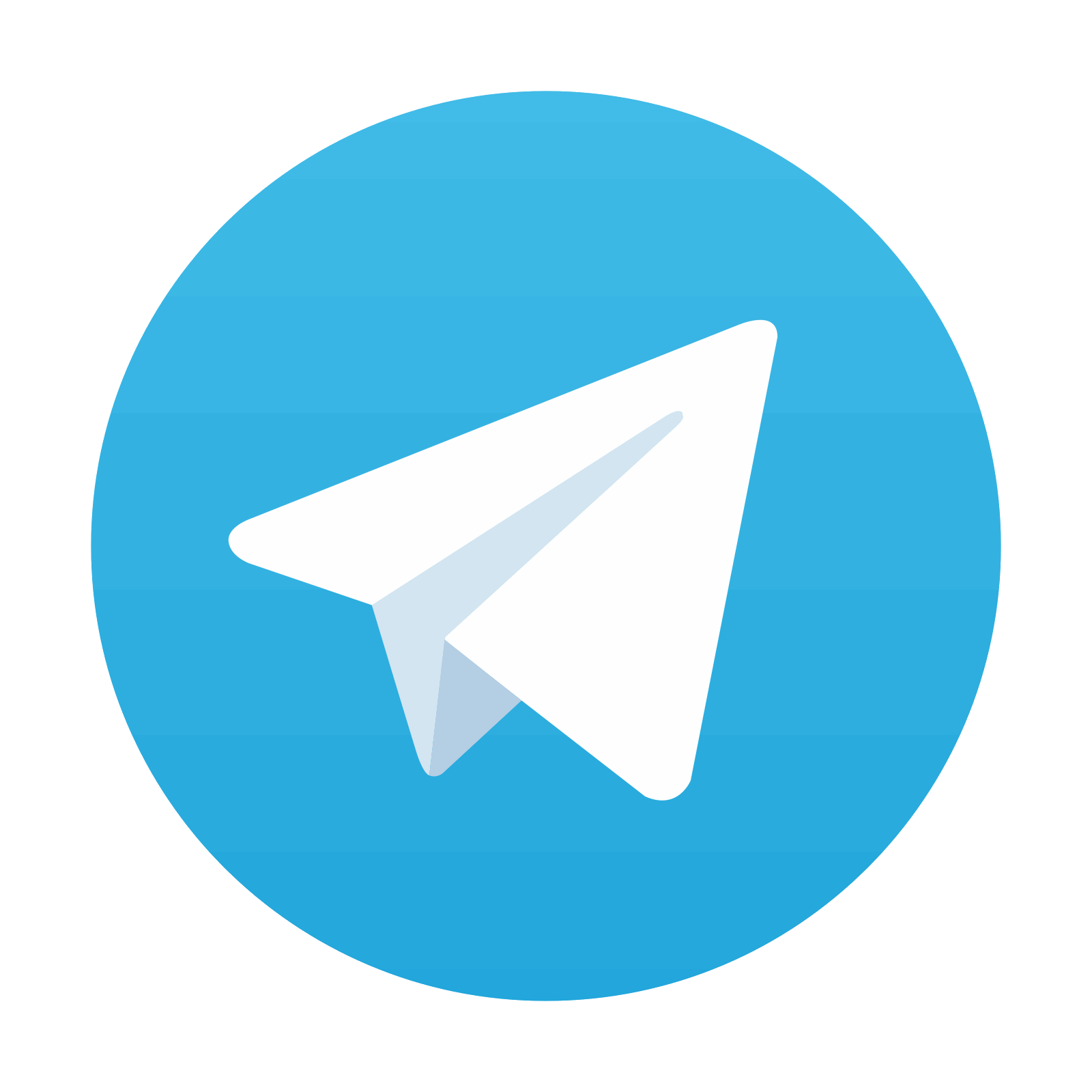
Stay updated, free dental videos. Join our Telegram channel

VIDEdental - Online dental courses
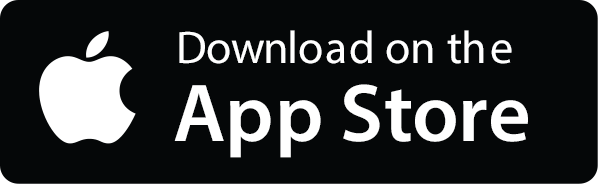
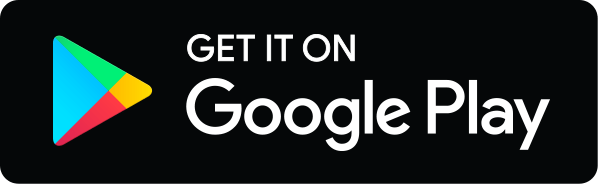
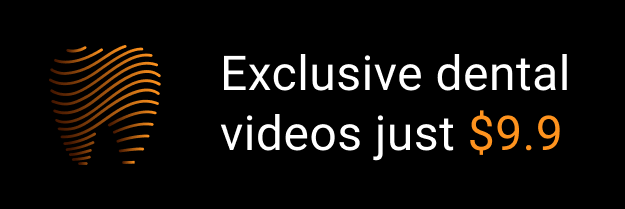