Clinical endodontics encompasses a number of treatments, but they have in common the goal of preventing and treating microbial contamination of pulps and root canal systems. Treatment of traumatic dental injuries and prophylactic treatment of vital pulps are fundamentally different from pulpectomies and root canal instrumentation of teeth with infected pulps (see Chapter 1 for more details on diagnosis).
Endodontic therapy is directed toward one specific set of aims: to cure or prevent periradicular periodontitis. The ultimate aim is for patients to retain their natural teeth in function and aesthetics.
To date, many treatment modalities, including the use of nickel-titanium rotary instruments, have not consistently provided a statistically relevant impact on treatment outcomes. This poses a problem in the age of evidence-based therapy, because new therapeutic techniques should deliver improved clinical results over standard procedures. However, the few pertinent clinical trials and numerous in vitro studies do suggest that certain practices in canal preparation and disinfection are more appropriate than others. This chapter will summarize relevant information.
Orthograde root canal treatment is a predictable and usually highly successful procedure, both in relatively straightforward ( Fig. 6-1 ) and more complex cases ( Fig. 6-2 ). Studies and reviews report favorable outcome rates of up to 95% for the treatment of teeth diagnosed with irreversible pulpitis and positive outcome rates of up to 85% for infected, necrotic teeth.


Microorganisms can breach dental hard-tissue barriers through several avenues, the most common being dental caries ( Fig. 6-3 ). Shaping and cleaning procedures ( Box 6-1 ) as part of root canal treatment are directed against microbial challenges to the root canal system. However, disinfection per se does not guarantee long-term retention of root canal-treated teeth; there is good evidence that this outcome is closely related to placement of an adequate coronal restoration. Moreover, the impact of preservation of radicular structural strength should not be underestimated.

The primary objectives in cleaning and shaping the root canal system are to do the following:
- ◆
Remove infected soft and hard tissue
- ◆
Give disinfecting irrigants access to the apical canal space
- ◆
Create space for the delivery of medicaments and subsequent obturation
- ◆
Retain the integrity of radicular structures
Principles of Cleaning and Shaping
Endodontists agree that a major biologic aim of root canal therapy is to address apical periodontitis by disinfection and subsequent sealing of root canal systems. However, considerable disagreement exists over how this goal should be achieved. Although the terms cleaning and shaping are often used to describe root canal treatment procedures, reversing the order to shaping and cleaning more correctly reflects the fact that enlarged canals direct and facilitate the cleaning action of irrigants and the removal of infected dentin.
Planktonic microorganisms in the pulp cavity and coronal root canal may be readily killed by irrigants early in a procedure, but bacteria in less accessible canal areas or in biofilms still can elicit or maintain apical periodontitis. In everyday practice, these bacteria can be targeted only after mechanical root canal preparation.
Mechanical Objective
An ideal mechanical objective of root canal instrumentation is complete and centered incorporation of the original canals into the prepared shape, meaning that all root canal surfaces are mechanically prepared ( green areas in Fig. 6-4 , A and B ). This goal is unlikely to be met with current techniques.

Preparation errors such as deviations, zipping, and perforations should be absent. Although these negative effects of canal shaping and other procedural mishaps (discussed later) per se may not affect the probability of a favorable outcome, they leave parts of the root canal system inaccessible for disinfection and are undesirable for that reason alone.
Another important mechanical objective is to retain as much cervical and radicular dentin as possible so as not to weaken the root structure, thereby preventing root fractures. Before root canal shaping, dentin wall thickness dimensions of 1 mm and below have been demonstrated in anatomic studies. Straightening of canal paths can lead to thinning of curved root walls ( Fig. 6-5 ). Although no definitive minimal radicular wall thickness has been established, 0.3 mm is considered critical by some authors. To avoid overpreparation and outright perforations, adequate access cavity preparation and optimal enlargement of the coronal third of the root canal has to be ascertained (discussed later).

Biologic Objective
Schilder suggested that canals should be prepared to a uniform and continuous taper ; however, this guideline was aimed at facilitating obturation rather than targeting antimicrobial efficacy. For optimal disinfection, the preparation shape and antimicrobial efficacy are intimately related through the removal of infected pulp and dentin ( Fig. 6-6 ) and creation of space for delivery of irrigants.

Traditionally, fluids have been dispensed passively into root canals by syringe and needle ( Fig. 6-7 ). When delivered with passive needle irrigation, solutions have been shown to progress only 1 mm farther than the tip of the needle. Enlarged apical canals and finer needles are likely to allow increasingly deeper needle placement, and this improves debridement and disinfection of canals. However, thorough cleaning of the most apical part of any preparation remains difficult, especially in narrow and curved canals.

Technical Objective
Although a continuous taper that encompasses the original shape and curvature of a given root canal is an accepted goal, final apical preparation size remains a much-disputed entity in root canal therapy, as does final taper of the preparation. Arguments were made for better disinfection with larger sizes (i.e., #50 or greater) in combination with smaller tapers of .02 to .05. Others found no difference whether the selected final size was small or large. A self-adjusting file was introduced, which does not prepare canals to a specific normed size; its debridement effect is thought to result from a greater radicular wall contact, notably in buccolingually wide canals.
Clinical Issues
A wide spectrum of possible strategies exists for attaining the goal of removing the canal contents and eliminating infection. Lussi and colleagues introduced an approach to removing canal contents and accomplishing disinfection that did not involve the use of a file: the noninstrumentation technique. This system consisted of a pump, a hose, and a special valve that was cemented into the access cavity ( Fig. 6-8, A ) to provide oscillation of irrigation solutions (1% to 3% sodium hypochlorite) at a reduced pressure. Although several in vitro studies suggested that canals can be cleaned and subsequently filled using this noninvasive system (see Fig. 6-8, B and C ), preliminary clinical results have not been as convincing (see Fig. 6-8, D ).

At the opposite end of the spectrum is a treatment technique that essentially removes all intraradicular infection through extraction of the tooth in question (see Fig. 6-8, E and F ). Almost invariably, periradicular lesions heal after extraction of the involved tooth.
Clinical endodontic therapy takes place somewhere along this spectrum of treatment strategies. This is reflected in some of the controversies that surround the cleaning and shaping process, such as how large the apical preparation should be and what are the correct diameter, length, and taper. Once the decision has been made to initiate endodontic treatment, the clinician must integrate his or her knowledge of dental anatomy, immunology, and bioengineering science with clinical information.
Endodontic therapy has been compared to a chain of events, wherein the chain is only as strong as each individual link. For the purposes of this chapter, shaping and cleaning of the root canal system is considered a decisive link, because shaping determines the efficacy of subsequent procedures. It includes mechanical debridement, the creation of space for the delivery of medicaments, and optimized canal geometries for adequate obturation. These tasks are attempted within a complex anatomic framework, as recognized in the early 20th century by Walter Hess ( Fig. 6-9 ; see also Chapter 5 for a complete description of root canal anatomy).

A clinician must choose appropriate strategies, instruments, and devices to overcome challenges and accomplish precise preparation in shape, length, and width. This allows endodontic therapy to address various forms of the disease processes described previously ( Fig. 6-10 ). Recall radiographs taken at appropriate intervals predictably demonstrate longevity and favorable outcomes (see Figs. 6-1, 6-2, and 6-11 ) if a systematic approach to root canal shaping is adhered to (see Box 6-1 ).


Endodontic files traditionally have been manufactured according to empiric designs, and most instruments still are conceived based on individual clinicians’ philosophies rather than developed through an evidence-based approach. Similar to the development of composite resins in restorative dentistry, the development of new files is a fast and market-driven process. With new instruments becoming available, the clinician may find it difficult to pick the file and technique most suitable for an individual case. Clinicians must always bear in mind that all file systems have benefits and weaknesses. Ultimately, clinical experience, handling properties, usage safety, and case outcomes, rather than marketing or the inventor’s name, should decide the fate of a particular design. The following section describes typical instruments used in root canal shaping.
Endodontic Instruments
General Characteristics
Design Elements
Root canal preparation instruments such as K-files and nickel-titanium rotary instruments follow certain design principles that relate to drills and reamers used for work in wood and metal, respectively, whereas other instruments such as broaches and Hedström files do not find a direct technologic correlate. Design elements such as the tip, flutes, and cross sections are considered relevant for files and reamers used in rotary motion. These pertinent aspects are briefly described next; for a more detailed review, the reader is referred to the literature.
Tip Design
In root canal preparation, an instrument tip has two main functions: to guide the file through the canal and to aid the file in penetrating deeper into the canal. A clinician unfamiliar with the tip design, in particular of a rotary instrument, may do either of the following: (1) transport the canal (if the tip is capable of enlarging and is used too long in one position in a curved canal) or (2) encounter excessive torsion and break the file (if a noncutting tip is forced into a canal with a smaller diameter than the tip).
The angle and radius of its leading edge and the proximity of the flute to its actual tip end determines the cutting ability of a file tip. Cutting ability and file rigidity determine the propensity to transport the canal. The clinician must keep in mind that as long as a flexible file with a noncutting tip is engaged, 360-degree canal transportation is unlikely to occur.
Studies have indeed shown that tip design affects file control, efficiency, and outcome in the shaping of root canal systems. The tip of the original K-file resembled a pyramid; instrument tips have been described as cutting, noncutting, and partially cutting, although no clear distinction exists among the three types ( Fig. 6-12 ).

Noncutting tips, also called Batt tips, are created by grinding and smoothing the apical end of the instrument (see Fig. 6-12, A ). A tip modification was introduced with the Flex-R file, which was manufactured fully by grinding so that the transitional angles were smoothed laterally between the tip and the instrument’s working parts. Similar techniques are required to manufacture NiTi K-files.
For NiTi rotary files, typically rounded noncuttings tips are used (see Fig. 6-12, B ), which effectively prevent preparation errors that were found with earlier so-called safe cutting tips. One exception to this rule is the type of rotary specifically designed as a retreatment instrument; cutting tips in that case facilitate removal of the existing root canal filling material and are sufficiently safe.
Longitudinal and Cross-Sectional Design
The flute of the file is the groove in the working surface used to collect soft tissue and dentin chips from the wall of the canal. The effectiveness of a flute depends on its depth, width, configuration, and surface finish. The surface with the greatest diameter that follows the groove (where the flute and land intersect) as it rotates forms the leading (cutting) edge, or the blade of the file. The cutting edge forms and deflects chips along the wall of the canal and severs or snags soft tissue. Its effectiveness depends on its angle of incidence and sharpness.
Some instruments have a feature between trailing and cutting edge that forms a larger contact area with the radicular wall; this surface is called a radial land ( Fig. 6-13 ). Such a land is thought to reduce the tendency of the file to thread into the canal. It also supports the cutting edge and limits the depth of cut; its position relative to the opposing cutting edge and its width determine its effectiveness. On the other hand, landed files are typically less cutting efficient compared to triangular cross sections.

To reduce frictional resistance, some of the surface area of the land that rotates against the canal wall may be reduced to form the relief . The angle the cutting edge forms with the long axis of the file is called the helical angle (see Fig. 6-13 ).
If a file is sectioned perpendicular to its long axis, the rake angle is the angle formed by the leading edge and the radius of the file through the point of contact with the radicular wall. If the angle formed by the leading edge and the surface to be cut is 90 degrees, the rake angle is said to be neutral. The rake angle may be negative or scraping ( Fig. 6-14, A ) or positive or cutting ( Fig. 6-14, C ).

The cutting angle is considered a better indication of a file’s cutting ability and is determined by measuring the angle formed by the leading edge of the file and a tangent to the radicular wall in the point of contact. The clearance angle corresponds to the cutting angle at the trailing edge of the file and, in case of reciprocating action, becomes the cutting angle. The sum of cutting angle and rake angle is 90 degrees.
The pitch of the file is the distance between a point on the leading edge and the corresponding point on the adjacent leading edge (the distance from one “spiral twist” to the next) (see Fig. 6-13 ). The smaller the pitch or the shorter the distance between corresponding points, the more spirals the file has and the greater the helix angle. Although K-files have a constant pitch typically in the range of 1 mm, many NiTi rotaries have a variable pitch, one that changes along the working surface. When variable pitch is used, usually tighter spirals are located close to the tip of the file and more space between the flutes is located toward the coronal part of the file. A longitudinal section through an instrument reveals the core (see Fig. 6-13 ). The outer diameter of a tapered instrument increases from the file tip toward the handle; depending on core dimensions, the flute may become proportionately deeper, resulting in a core taper that may be different from the external taper .
The cutting angles, helix angles, and external and core tapers may vary along the working surface of the file, and the ratios of these quantities can vary among instruments of the same series. A change in any of these features may influence the file’s effectiveness or its propensity for breakage as it progresses into the canal space.
Taper
The taper usually is expressed as the amount the file diameter increases each millimeter along its working surface from the tip toward the file handle. For example, a size #25 file with a .02 taper would have a 0.27-mm diameter 1 mm from the tip, a 0.29-mm diameter 2 mm from the tip, a 0.31 mm diameter 3 mm from the tip, and so forth. Instruments can have constant or variable taper: Some manufacturers express the taper in terms of percentage (e.g., a .02 instrument has been said to have a 2% taper; Fig. 6-15 ). Current instrument developments include variations in helical angle, pitch, and taper along the cutting portion, which along with variations in alloy and rotational speed (rpm) all affect cutting behavior. The ability to determine cross-sectional diameter at a given point on a file can help the clinician determine the file size in the point of curvature and the relative stress being placed on the instrument. Instruments with greater tapers are designed so that the tip of the instrument functions as a guide and the middle and coronal part of the instrument’s working part is the one engaging the canals walls.

ISO Norms
Standardized specifications have been established to improve endodontic instrument quality. For example, the International Standards Organization (ISO) has worked with the Fédération Dentaire Internationale (FDI) to define specifications. These standards are designated with an ISO number. The American Dental Association (ADA) also has been involved in this effort, as has the American National Standards Institute (ANSI); these standards are designated with an ANSI number. However, new instrument designs have resulted in a need for reconsideration of the standards.
Two ISO standards pertain to endodontic instruments. ISO No. 3630-1 deals with K-type files as does ANSI No. 28), Hedström files (ANSI No. 58), and barbed broaches and rasps (ANSI No. 63). ISO No. 3630-3 deals with condensers, pluggers, and spreaders (ANSI No. 71); however, the term ISO-normed instruments is often used as a synonym for K-files (see Fig. 6-15 ).
One important feature of ISO-normed hand instruments is a defined increase in tip diameter of 0.05 or 0.1 mm, depending on the instrument size ( Fig. 6-16 ). ISO-normed K- and Hedström files ( Fig. 6-17 ) are available in different lengths (21, 25, and 31 mm), but all have a 16-mm-long section of cutting flutes (see Figs. 6-12 and 6-15 ). The cross-sectional diameter at the first rake angle of any file is labeled D0. The point 1 mm coronal to D0 is D1, the point 2 mm coronal to D0 is D2, and so on up to D16. The D16 point is the largest diameter of an ISO-normed instrument. Each file derives its numeric name from the diameter at D0 and is assigned a specific color code (see Fig. 6-15 ).


Another aspect of ISO files is the standard taper of 0.32 mm over 16 mm of cutting blades, or 0.02 mm increase in diameter per millimeter of flute length (.02 taper). Thus, a size #10 instrument has a diameter of 0.1 mm at D0 and a corresponding diameter of 0.42 mm at D16 [0.1 mm + (16 * 0.02 mm)]. For a size #50 instrument, the diameters are 0.5 mm at D0 and 0.82 mm at D16.
The ISO-normed design is a simplification that has specific disadvantages, and it may explain the clinical observation that enlarging a root canal from size #10 to #15 is more difficult than the step from size #55 to #60. The introduction of K-type files with tip sizes between the ISO-stipulated diameters seemed to solve the problem. However, the use of such files is not universally recommended, perhaps because the approved machining tolerance of ±0.02 mm could negate any intended advantages. Moreover, although ±0.02 mm tolerance is stipulated by the ISO norm, most manufacturers do not adhere to it.
Another suggested modification relates to tips with a constant 29% percentage of diameter increments. This sizing pattern creates smaller instruments that carry less of a workload. However, the intended advantage is offset by larger diameters, because the 29% increase between successive files is actually greater than the percentage change found in the ISO file series.
Further changes to the numbering system for files with different sizes have been implemented by several manufacturers. One system has introduced “half” sizes in the range of #15 through #60, resulting in instruments in sizes #15, #17.5, #20, #22.5, and so on.
Alloys
There are currently two principally different types of alloys used for endodontic instruments, stainless steel and nickel-titanium. Most manually operated endodontic instruments are fabricated from stainless steel and have considerable resistance to fracture. A clinician who is careful in applying force and adheres to a strict program of discarding instruments after use should have few instrument fractures. Stainless steel files are comparably inexpensive so that adequate cleaning and sterilization for reuse of files in sizes up to #60 may not be cost effective. If this is the case, files in the range up to #60 may be considered disposable instruments.
Several burs and instruments designed for slow-speed handpiece operation such as Gates Glidden drills, Peeso burs, and pilot drills for intraradicular posts are also manufactured from stainless steel. Instruments designed for rotary root canal instrumentation, however, are typically made of nickel-titanium. This alloy offers unique properties, specifically flexibility and corrosion resistance.
Physical and Chemical Properties of Steel and Nickel Titanium Alloys
Basic engineering terms relate to metals and their behavior when used to manufacture endodontic instruments. Stress-strain diagrams describe the response of metal wires under loading depending on their crystal configuration ( Fig. 6-18 ).

During the development of the equiatomic nitinol [this acronym is derived from ni ckel- ti tanium investigated at the N aval O rdinance L aboratory] alloy (55% [by weight] nickel and 45% [by weight] titanium), several effects were noted that relate to its specific crystal arrangement with two stable main phases, austenite and martensite ( Fig. 6-19 ): a shape memory effect as temperature- and strain-dependent pseudoelasticity, all attributable to specific thermodynamic properties of the new alloy.

Walia and colleagues thought that the pseudoelastic properties of 55-nitinol might prove advantageous in endodontics and initially tested hand instruments. They found that size #15 NiTi instruments were two to three times more flexible than stainless steel instruments; moreover, the instruments showed superior resistance to angular deflection.
Furthermore, hardly any plastic deformation of cutting flutes was recorded when an instrument was bent up to 90 degrees, and forces required to bend endodontic files to 45 degrees were reduced by 50% with NiTi. Serene and colleagues speculated that heat, probably during sterilization cycles, could even restore the molecular structure of used NiTi files, resulting in an increased resistance to fracture. Such a behavior is claimed to occur for current martensitic instruments.
These unusual properties are the result of a molecular crystalline phase transformation in specific crystal structures of the austenitic and martensitic phases of the alloy. External stresses transform the austenitic crystalline form of NiTi into a martensitic crystalline structure that can accommodate greater stress without increasing the strain. As a result, a NiTi file has transformational elasticity, also known as pseudoelasticity, or the ability to return to its original shape after being deformed (see Fig. 6-19, B ). This property dictates that typically NiTi instruments are manufactured by milling rather than twisted; twisting incorporates plastic deformation and is used, for example, to produce stainless steel K-files.
Similar to the application of deforming forces, heat can also result in phase transformation (see Fig. 6-19, A ) from austenite to martensite and vice versa. Moreover, thermal conditions during the production of the raw wire can be used to modify its properties, most important of which is its flexibility. For austenitic endodontic instruments, a recoverable elastic response of up to 7% is expected ( Fig. 6-20 ). However, more martensitic instruments will have less of an elastic range and are more likely to plastically deform during use.

Experiments designed to test fracture resistance demonstrate physical properties of endodontic instruments; following the pertinent ISO norm, 3630-1 graphs such as those shown in Fig. 6-20 are generated and compared among different designs.
Attempts to improve the NiTi alloy continue, and reports indicate that new NiTi alloys may be five times more flexible than currently used alloys. NiTi instruments may have imperfections such as milling marks, metal flash, or rollover. Some researchers have speculated that fractures in NiTi instruments originate at such surface imperfections.
Surface irregularities may provide reservoirs of corrosive substances, most notably sodium hypochlorite (NaOCl). Chloride corrosion may lead to micropitting and possibly subsequent fracture in NiTi instruments. Immersion in various disinfecting solutions for extended periods (e.g., overnight soaking) produced corrosion of NiTi instruments and subsequent decreased torsional resistance. For ProTaper, RaCe, and ProFile385 instruments, 2-hour immersion damaged the integrity of the alloy. Other authors did not find a corrosion-related effect on K3-files or ProFile instruments.
Regular reprocessing procedures do not seem to significantly affect NiTi rotary instruments. In one study, only limited material loss occurred when NiTi LightSpeed instruments were immersed in 1% and 5% NaOCl for 30 to 60 minutes. Corrosion of NiTi instruments used in the clinical setting, therefore, might not significantly contribute to fracture except when the instruments are immersed in warmed NaOCl for longer than 60 minutes. In the majority of studies, sterilization procedures do not appear to negatively impact torsional strength or fatigue resistance of most NiTi instruments: austenitic and martensitic alloy behaved grossly similarly in this aspect.
There is an ongoing discussion over the impact of other aspects of clinical usage on the mechanical properties of NiTi rotaries. Most likely, clinical usage leads to some changes in the alloy, potentially through work hardening.
Another strategy to improve surface characteristics is electropolishing; also surface coatings and ion implantation have been tried. Electropolishing is a process that removes surface irregularities such as flash and bur marks. It is thought to improve material properties, specifically fatigue and corrosion resistance; however, the evidence for both these claims is mixed. One study found an extension of fatigue life for electropolished instruments, whereas others found no improvement of fatigue resistance of electropolished instruments. Boessler and colleagues suggested a change in cutting behavior with an increase of torsional load after electropolishing.
Corrosion resistance of electropolished NiTi rotaries is also controversial. One study found superior corrosion resistance for electropolished RaCe instruments, whereas another study demonstrated similar corrosion susceptibility for RaCe and nonelectropolished ProFile instruments. Attempts have been made to improve surface quality by coating it with titanium nitride. The latter process also seems to have a beneficial effect on cutting efficiency.
Perhaps more relevant than surface treatment are modification of the base alloy that significantly alter material properties within the atomic ratio. The first commercialized alloy was M-Wire (SportsWire, Langley, OK), which was shown to have higher fatigue resistance with similar torsional strength. More recently, instrument are produced through production process that include annealing and cooling steps to create instruments that, after cold working during the production process, are more martensitic during dental treatment, as defined by their M s temperature (see Fig. 6-19, A ). Under clinical conditions these alloys are more flexible and present higher fatigue resistance. Examples are the newly introduced gold and blue alloys types (Dentsply Tulsa Dental Specialties, Tulsa, OK) or so-called controlled memory alloy used in Hyflex instruments (Coltene Endo, Cuyahoga Falls, OH).
Manually Operated Instruments
Endodontic trays contain many items familiar from general dentistry but certain hand instruments are designed specifically for endodontic procedures. This includes instruments employed for procedures inside the pulp space, for example hand and engine-driven instruments for root canal preparation, and energized instruments for root canal shaping. Special instruments and devices for root canal obturation are selected for filling prepared canal spaces.
K-Type Instruments
Manually operated instruments are generically called files. Defined by function, files are instruments that enlarge canals with apico-coronal insertion and withdrawal motions.
Historically, root canal instruments were manufactured from carbon steel. Subsequently, the use of stainless steel greatly improved the quality of instruments. More recently, K-type files manufactured from NiTi were introduced (NiTiFlex, Dentsply Maillefer, Ballaigues, Switzerland).
Files were first mass produced by the Kerr Manufacturing Co. of Romulus, Michigan, in the early 1900s, hence the name “K-type” file (or K-file) and K-type reamer (K-reamer). K-files and K-reamers were manufactured by the same process—that is, by twisting square or triangular metal blanks along their long axis, producing partly horizontal cutting blades (see Fig. 6-17, A ). Three or four equilateral, flat surfaces were ground at increasing depths on the sides of a piece of wire, producing a tapered pyramidal shape. The wire then was stabilized on one end, and the distal end was rotated to form the spiral instrument. The number of sides and the number of spirals determine whether the instrument is best suited for filing or reaming. Generally, a three-sided configuration with fewer spirals (e.g., 16 per 16-mm working portion) is used for reaming (i.e., cutting and enlarging canals with rotational motions). A file has more flutes per length unit (e.g., 20) than a reamer, whereas a three- sided or triangular configuration is generally more flexible than a four-sided one.
K-type instruments are useful for penetrating and enlarging root canals. Generally, a reaming motion (i.e., constant file rotation) causes less transportation than a filing motion (translational or “in and out” motion). ( Transportation is defined here as the excessive loss of dentin from the outer wall of a curved canal in the apical segment, as described in more detail later.)
Stainless steel K-files may be precurved by overbending. This procedure subjects the file to substantial strain and should therefore be done carefully. Permanent deformation occurs when the flutes become wound more tightly or opened more widely ( Fig. 6-21 ). When such deformation occurs, the instrument should no longer be used; file fracture is likely to occur during clockwise motion after plastic deformation.

Interestingly, although the force required for failure is the same in both directions of rotation, failure occurs in the counterclockwise direction at half the number of rotations required for failure in the clockwise direction. Therefore, K-type instruments should be operated more carefully when pressure is applied in a counterclockwise direction.
Cross-sectional analysis of a K-file reveals why this design allows careful application of clockwise and counterclockwise rotational and translational working strokes: the cross section is symmetrical with negative rake angles, allowing dentin to be adequately cut in both clockwise and counter-clockwise direction.
Reamers are instruments that are similar to K-files in overall design, but they have fewer cutting flutes per mm of the working surface. They are more appropriate for twisting motion and are less frequently used today.
H-Type Instruments
H-type instruments, also known as Hedström files (see Fig. 6-17, B ), are milled from round, stainless steel blanks. These files are very efficient for translational strokes, because of a positive rake angle and a blade with a cutting rather than a scraping angle. Rotational working movements are strongly discouraged because of the possibility of fracture. Hedström files up to size #25 can be efficiently used to relocate canal orifices and, with adequate filing strokes, to remove overhangs. Similarly, wide oval canals can be instrumented with Hedström files as well as with rotary instruments. On the other hand, overzealous filing can lead to considerable thinning of the radicular wall and strip perforations ( Fig. 6-22 ). As with stainless steel K-files, Hedström files have been described as disposable instruments.

Bending Hedström files results in points of greater stress concentration than in K-type instruments. These prestressed areas may lead to the propagation of cracks and ultimately fatigue failure. Note that clinically, fatigue fractures may occur without visible signs of deformation.
Hedström files are produced by grinding a single continuous flute into a tapered blank. Computer-assisted machining technology has allowed the development of H-type instruments with complex forms. This process, called multiaxis grinding, allows adjustment of the rake angle, helix angle, multiple flutes, and tapers and is also used to fabricate the majority of NiTi instruments. Because the H-file generally has sharper edges than the K-file, it has a tendency to thread into the canal during rotation, particularly if the instrument’s blades are nearly parallel. Awareness of threading-in forces is important to avoid instrument failure.
Effectiveness and Wear of Instruments
The ability of an endodontic hand instrument to cut and machine dentin is essential; however, no standards exist for either the cutting or the machining effectiveness of endodontic files, nor have clear requirements been established for resistance to wear. In any study of the effectiveness of an instrument, two factors must be investigated: (1) effectiveness in cutting or breaking loose dentin and (2) effectiveness in machining dentin.
Attempts have been made to evaluate the effectiveness of an instrument when used with a linear movement. Collectively, these studies showed that instruments might differ significantly, not only when comparing brands and types but also within one brand and type. For K-files, effectiveness varies 2 to 12 times between files of the same brand. This variation for Hedström files is greater, ranging from 2.5 to more than 50 times. The greater variation among Hedström files is easy to understand because the H-file is the result of more individual grinding during manufacture than the conventional K-file, which is difficult to alter much during the manufacturing process. For example, during the grinding of a Hedström file, the rake angle can be modified to neutral or even slightly positive; this is impossible to achieve with a K-file.
During canal preparation, a file’s rake edge shaves off dentin that accumulates in the grooves between the rake edges. The deeper and larger this space, the longer the stroke can be before the instrument is riding on its own debris, making it ineffective.
These design variations and the rake angle of the edges determine the effectiveness of a Hedström file. Of the hybrid files, the K-Flex (SybronEndo, Orange, CA) file has properties similar to those of K-files. The Flex-R file (Integra Miltex, York, PA), which is a ground instrument with a triangular cross section similar to a K-file, more closely resembles a Hedström file in its variations in cutting behavior. It also is more effective at substrate removal than the K-files but cannot measure up to the H-files’ ability to machine radicular dentin.
Barbed Broaches
Barbed broaches ( Fig. 6-23 ) are produced in a variety of sizes and color codes. They are manufactured by cutting sharp, coronally angulated barbs into metal wire blanks. Broaches are intended to remove vital pulp from root canals, and in cases of mild inflammation, they work well for severing pulp at the constriction level in toto . The use of broaches has declined since the advent of NiTi rotary instruments, but broaching occasionally may be useful for expediting emergency procedures (see Chapter 18 ) and removing materials (e.g., cotton pellets or absorbent points) from root canals.

Low-Speed Engine-Driven Instruments
Burs
Specialized burs are available for endodontic access cavities. These burs are used in both high-speed and slow-speed handpieces and are manufactured from stainless steel. Access cavity preparation and used materials are described in detail in Chapter 5 ).
Gates-Glidden Drills
Gates-Glidden (GG) drills ( Fig. 6-24 ) have been used for more than 100 years without noteworthy design changes. Gates Glidden drills are typically used to enlarge coronal canal areas. When misused, GG drills can dramatically reduce radicular wall thickness.

GG drills are sized from 1 to 6 (with corresponding diameters of 0.5 to 1.5 mm); the number of rings or color-coding on the shank identifies the specific drill size. GG instruments are available in various lengths and by several manufacturers. Each instrument has a long, thin shaft with parallel walls and a short, oval cutting head with safety tips ( Fig. 6-24, B ); these drills are produced in stainless steel and NiTi varieties. Because of their design and physical properties, GG drills are side-cutting instruments; they can be used to cut dentin as they are withdrawn from the canal (i.e., on the outstroke). Used this way, their cutting action can deliberately be directed away from external root concavities in single-rooted and furcated teeth. GG drills should be used only in the straight portions of a canal.
Two procedural sequences have been proposed: with the step-down technique, the clinician starts with a large drill and progresses to smaller ones; conversely, with the step-back technique, the clinician starts with a small drill and progresses to larger ones. With the step-down approach, the clinician must select a GG instrument with a diameter that allows introduction into the respective orifice and progression for about 1 mm. The subsequent smaller instruments progress deeper into the canal until the coronal third has been preenlarged. This technique efficiently opens root canal orifices and works best when canals exit the access cavity without severe angulations. Opened orifices simplify subsequent cleaning and shaping procedures and help establish a smooth glide path from the access cavity into the root canal system.
With the step-back approach, a small GG instrument is introduced into the canal, and dentin is removed on the outstroke. This process is repeated with the next larger GG instrument, which is again worked shorter than the preceding smaller one. In this way, the coronal third of the root canal is enlarged and dentin overhangs are removed.
When used adequately, GG instruments are inexpensive, safe, and clinically beneficial tools. Gates-Glidden drills may be used safely and to their fullest potential at 750 to 1500 rpm. High revolutions per minute (rpm), excessive pressure, an incorrect angle of insertion, and the use of GG instruments to aggressively drill into canals have resulted in mishaps such as strip perforation. The preferred mode of action for GG drills is against the outer canal wall, away from the canal curvature. Also, cyclic fatigue may cause GG instruments to fracture when used in curved canal areas, and the short cutting heads may fracture with high torsional loads. As with nickel-titanium rotary instruments, GG drills work best when used in electric gear reduction handpieces rather than with air motors.
Peeso Drills (Reamers)
Peeso drills are typically used in root canal preparation either for coronal flaring or during post preparation. These drill are at this point manufactured mostly from stainless steel by milling similar to the Gates Gliddens. Peeso drills are also used in the electric slow-speed handpiece; the rotational speed is the range of 800 to 1200 rpm; the cutting flutes are more parallel and longer compared to GG drills but shorter than the 16 mm prescribed for ISO-normed hand files. Peeso drills are classified as type P and type B-l, as defined by ISO norm 3630-2. The sizing for these drills is also numbers 1 to 6, similar to GGs. Peeso drills are available with cutting and noncutting tips and should be used with caution to avoid excessive preparation and thinning of radicular dentin walls.
Engine-Driven Instruments for Canal Preparation
Instrument Types
Engine-driven instruments for root canal preparation made of stainless steel have been in use for more than half a century—for the first decades, mainly in handpieces that permitted reciprocation (alternating clockwise-counterclockwise motion). The major two problems with this type of instrument were canal transportation (discussed later) and file fracture. This changed with the advent of NiTi rotaries from about the early 1990s; the much more flexible alloy allowed continuous rotation and reduced both canal preparation errors and instrument fracture compared to earlier engine driven techniques.
Currently, more than 50 types of rotary instrument systems have been described and more continue to be developed. The instruments vary greatly in terms of design, alloy used, and recommended cutting movement ( Table 6-1 ). Various built-in features may help prevent procedural errors, increase efficiency, and improve the quality of canal shaping.
Group | Enlargement Potential | Preparation Errors | Fracture Resistance | Clinical Performance |
---|---|---|---|---|
I ProFile , ProSystem GT, GTX , Quantec , Pow-R , Guidance , K3 LightSpeed var. |
+, Depending in sizes, often time consuming | ++ Low incidence, usually <150 µm canal transportation | +/− Fatigue + Torsional load, depending on system |
++ Good, depending on treatment conditions; no difference between instruments shown so far, except for inexperienced clinicians, who perform better with landed instruments |
II ProTaper var. , RaCe , Hero 642 , FlexMaster , Mtwo , Sequence , Alpha … ProFile Vortex Twisted File |
+/−, Good with use of hybrid techniques | +/−, Overall more demanding in clinicians’ ability | + Fatigue +/− Torsional load, depending on taper, handling |
|
III, EndoEZE AET , Liberator … WaveOne , Reciproc OneShape SAF |
Limited | Varies, Liberator— EndoEZE AET— WaveOne, Reciproc+ |
Varies + with WaveOne, Reciproc | Varies |
For example, a longer pilot tip may guide the instrument and help to stay centered in the canal long axis. Alternatively, a file can be given an asymmetric cross section to help maintain the central axis of the canal.
Another direction of instrument development is the prevention of instrument fractures ( Fig. 6-25 ). There are several ways to modify an instrument to make it less likely to fracture; for example, increasing the core diameter will increase torsional resistance. Another approach is to use a torque-limiting motor (discussed later). Alternatively, a zero taper or nearly parallel and fluted working portion of the file can be provided for curved canals so that the apical portion of the canal can be enlarged without undue file stress and compression of debris. More recently a reciprocation motion was reapplied to NiTi rotary instruments to prevent threading-in and instrument fractures in general.

Yet another, more recent, direction of instrument development is to improve shaping as it relates to circumferential root canal wall contact. One example for this strategy is a file manufactured from an expandable and flexible hollow NiTi tube, the so-called Self-adjusting File (ReDent-Nova, Ra’anana, Israel, Israel) ( Fig. 6-26 ). More recently, a substantial S-shape was superimposed onto a flexible NiTi rotary that provides a larger envelope of motion while maintaining a limited maximum flute diameter (TRUShape, Dentsply Tulsa Dental Specialties).

Marketed files vary greatly in terms of their specific design characteristics, such as tip sizing, taper, cross section, helix angle, and pitch (see Fig. 6-13 ). Some of the early systems have been removed from the market or relegated to minor roles; others, such as ProFile (Dentsply Tulsa Dental Specialties), are still successfully used. More recently added instruments vary in cross-sectional and longitudinal design ( Fig. 6-27 ). However, the extent to which clinical outcome changes (if any) will depend on design characteristics is difficult to forecast.

Most instruments described in the following section are manufactured by a grinding process, although some are produced by laser etching and yet others by plastic deformation under specific heating and cooling processes. Surface quality has been considered an important detail because cracks that arise from superficial defects may play a role in instrument fracture. Moreover, surface defects such as metal flash and rollover are common in unused NiTi instruments.
Many variables and physical properties influence the clinical performance of NiTi rotaries. Clinical practice has generated much of what is known about NiTi instruments, including reasons for instrument fracture and instrument sequences. These instruments have substantially reduced the incidence of relevant canal shaping errors, but they are also thought to fracture somewhat more easily than hand instruments.
Table 6-1 and the following sections describe the instrument groups most widely used for root canal preparation at this point in time. Most basic strategies apply to all NiTi rotary instruments, regardless of the specific design or brand. However, three design groups need to be analyzed separately: group I, instruments designed for passive preparation; group II, rotary instruments designed for active cutting; and group III, unique designs that do not fit in either group I or group II.
Group I: Passive Preparation; Presence of Radial Lands
The first commercially successful rotary instruments were ProFile (Dentsply Tulsa Dental Specialties), LightSpeed (marketed in its current form by SybronEndo), and GT rotaries (Dentsply Tulsa Dental Specialties) and have in common a cross section with so-called radial lands. These are created by three round excavations, also known as the U-shape. The design of the instrument tip and also the lateral file surface (radial land) guide the file as it progresses apically. This makes rotaries listed in group I fairly safe regarding preparation errors. On the other hand, it results in a reaming action rather than cutting of dentin and this makes them inefficient. Moreover, the smear layer produced with radial-landed rotaries is different in consistency and amount compared to the debris and smear created by cutting files.
LightSpeed
The LightSpeed file, developed by Drs. Steve Senia and William Wildey in the early 1990s and now also known as LS1, was introduced as an instrument different from all others because of its long, thin, noncutting shaft and short anterior cutting head. The same design principles apply to the currently available LSX instrument (SybronEndo) that is manufactured not by milling but by a stamping process. A full set consists of 25 LightSpeed LS1 instruments in sizes #20 to #100, including half sizes (e.g., 22.5, 27.5); LSX does not have half sizes, and a set includes sizes #20 to #80.
The original LightSpeed is a widely researched NiTi rotary instrument, and most reports have found that the system has a low incidence of overall and specific preparation errors. One report found similar shaping abilities for LSX and LightSpeed LS1 assessed with a double-exposure technique.
ProFile
The ProFile system (Dentsply Tulsa Dental Specialties) was introduced by Dr. Ben Johnson in 1994. ProFile instruments have increased tapers compared with conventional hand instruments. The ProFile system was first sold as the “Series 29” hand instruments in .02 taper, but it soon became available in .04 and .06 tapers. The tips of the ProFile Series 29 rotary instruments had a constant proportion of diameter increments (29%). Later, a ProFile series with ISO-sized tips (Dentsply Maillefer) was developed and marketed in Europe.
Cross sections of a ProFile instrument show a U-shape design with radial lands ( Fig. 6-28 ) and a parallel central core. Lateral views show a 20-degree helix angle, a constant pitch, and bullet-shaped noncutting tips (see Fig. 6-12 ). Together with a slightly negative rake angle, this configuration facilitates a reaming action on dentin rather than cutting. Also, debris is transported coronally and is effectively removed from the root canals.

ProFile instruments shaped canals without major preparation errors in a number of in vitro investigations. A slight improvement in canal shape was noted when .04 and .06 tapered instruments were used in an alternating fashion. Loss of working length did not exceed 0.5 mm and was not affected by the use of .06 tapered instruments. Comparative assessments in vitro suggested that ProFile prepared mesial canals in mandibular molars with less transportation than K3 and RaCe.
A more recent addition to the ProFile family of instruments was Vortex (Dentsply Tulsa Dental Specialties). The major change lies in the nonlanded cross section, whereas tip sizes and tapers are similar to existing ProFiles, hence these files are placed in group 2 (discussed later).
GT and GTX Files
The Greater Taper, or GT file, was introduced by Dr. Steve Buchanan in 1994. This instrument incorporates a radial landed cross-sectional design and was marketed as ProFile GT (Dentsply Tulsa Dental Specialties). The system was first produced as a set of four hand-operated files and later as engine-driven files. The instruments came in four tapers (.06, .08, .10, and .12), and the maximum diameter of the working part was 1 mm. The design limited the maximum shank diameter and decreased the length of the cutting flutes with increasing taper. The instruments had a variable pitch and a growing number of flutes in progression to the tip; the apical instrument diameter was 0.2 mm. Instrument tips were noncutting and rounded; these design principles are mostly still present in the current incarnation, the GTX instrument. The main differences are the M-Wire alloy type used and minor design and handling changes.
Studies on GT files found that the prepared shape stayed centered and was achieved with few procedural errors. A shaping assessment using micro-computed tomography [µCT; see Fig. 6-4 as an example)] showed that GT files machined statistically similar canal wall areas compared with ProFile and LightSpeed preparations. These walls were homogeneously planed and smooth. The more recently introduced GTX variant is manufactured from M-Wire and does not appear to have significantly improved physical properties or shaping capacity. GT instruments were available for small (tip size #20), medium (tip size #30), and large canal diameters (tip size #40).
K3
In a sequence of design developments by their inventor, Dr. McSpadden, the Quantec 2000 files were followed by the Quantec SC, the Quantec LX, and the current K3 system (all by SybronEndo). The overall design of the K3 is similar to that of the ProFile in that it includes instruments with .02, .04, and .06 tapers. The most obvious difference between the Quantec and K3 models is the K3’s unique cross-sectional design: a slightly positive rake angle for greater cutting efficiency, wide radial lands, and a peripheral blade relief for reduced friction. Unlike the Quantec, a two-flute file, the K3 features a third radial land to help prevent threading-in.
In the lateral aspect, the K3 has a variable pitch and variable core diameter, which provide apical strength. This intricate design is relatively difficult to manufacture, resulting in some metal flash.
Like most other current instruments, the K3 features a round safety tip, but the file is about 4 mm shorter than other files (although it has the same length of cutting flutes) because of the so-called Axxess handle. The instruments are coded by ring color and number.
Tested in vitro, K3’s shaping ability seems to be similar to that of the ProTaper and superior to that achieved with hand instruments. More recently, when curved canals in lower molars were shaped to a size #30 .06, K3 files had less canal transportation in a modified Bramante model than RaCe but more than ProFile.
Summary
Radial landed rotary instruments are considered very safe, even when accidentally taken beyond the confines of the root canal. Fracture resistance to torsional loading and cyclic varies depending on specific instrument design. The limited cutting efficacy of these files was perceived as a downside and is a reason that the market share has diminished. However, their excellent track record in clinical applications and research continues to support the use of rotaries listed in group I.
Group II: Active Cutting; Triangular Cross Section
Rotaries in group II all have a more active cutting flute design in common. Radial lands are absent (see Fig. 6-13 ), and this fact results in a higher cutting efficacy. This translates to a higher potential for preparation errors, in particular when the instrument is taken through the apical foramen, thus eliminating the guide derived from the noncutting tip.
ProTaper Universal
The ProTaper system originally comprised six instruments: three shaping files and three finishing files. This set is now complemented by two larger finishing files and a separate set of three rotaries tailored to retreatment procedures. The instruments were designed by Drs. Cliff Ruddle, John West, and Pierre Machtou. In cross sections, ProTaper shows a convex triangle with sharp cutting edges and no radial lands. The cross section of finishing files F3, F4, and F5 is slightly relieved for increased flexibility. The three shaping files have tapers that increase coronally, and the reverse pattern is seen in the five finishing files.
Shaping files nos. 1 and 2 have tip diameters of 0.185 mm and 0.2 mm, respectively, 14-mm-long cutting blades, and partially active tips. The finishing files (F1-F5) have tip diameters of 0.2, 0.25, 0.3, 0.4, and 0.5 mm, respectively, between D0 and D3, and the apical tapers are .07, .08, .09, .05, and .04, respectively. The finishing files have rounded noncutting tips.
Two aspects of handling have been emphasized for ProTaper. The first is the preparation of a glide path, either manually or with special rotary instruments. An enlargement to a size approaching the subsequent rotaries’ tips, at least larger than the file’s core diameter, prevents breakage and allows assessment of the canal size. This means that the glide path should correspond to a size #15 or #20. The second specific recommendation is the use of a more laterally directed “brushing” working stroke. Such a stroke allows the clinician to coronally direct larger files away from danger zones and counteract any “threading-in” effect. Both usage elements should be considered good practice for other instruments, particularly for more actively cutting ones.
In a study using plastic blocks, ProTaper created acceptable shapes more quickly than GT rotary, ProFile, and Quantec instruments, but it also created somewhat more aberrations. This was recently corroborated comparing preparations of mesial root canals in mandibular molars ex vivo with ProTaper Universal to Alpha (Gebr. Brasseler GmbH & Co. KG-Komet, Lemgo, Germany). In a comparison of ProTaper and K3 instruments (SybronEndo), Bergmans and colleagues found few differences, with the exception of some transportation by the ProTaper into the furcation region. A study using µCT showed that the ProTaper created consistent shapes in constricted canals, without obvious preparation errors, although wide canals may be insufficiently prepared with this system. It has been recommended that ProTaper be combined with less tapered, more flexible rotaries to reduce apical transportation.
A newer version of this system, called ProTaper Next was introduced in 2013. Current research suggests that mechanical properties of these instruments, manufactured from M-Wire, are better than ProTaper Universal.
No data are currently available on shaping outcomes or clinical results.
HERO 642, HERO Shaper
Several systems in group II (see Table 6-1 ) were designed with positive rake angles, which provide greater cutting efficiency. HERO instruments (MicroMega, Besançon, France) are an example. The original version was known as HERO 642 (the acronym HERO stands for h igh e lasticity in ro tation), and the name has now changed into HERO Shaper, with little apparent differences in the instrument design.
Cross sections of HERO instruments show geometries similar to those of an H-file without radial lands. Tapers of .02, .04, and .06 are available in sizes ranging from #20 to #45. The instruments are relatively flexible but maintain an even distribution of force into the cutting areas. HERO instruments have a progressive flute pitch and a noncutting passive tip, similar to other NiTi rotary systems. The instruments are coded by handle color.
Research with HERO files indicates a shaping potential similar to that of the FlexMaster (VDW, Munich, Germany) and ProFile, although in one study HERO files induced more changes in cross-sectional anatomy. HERO instruments also caused some aberrations when used in simulated canals with acute curves, but they were safer than Quantec SC instruments (SybronEndo). More recently, HERO Shapers had a better centering ability compared to RaCe instruments in resin blocks. Using a modified Bramante technique in vitro, earlier HERO 642 and current HERO Shaper rotaries showed no differences in canal cross sections before and after shaping.
FlexMaster
The FlexMaster file system is currently unavailable in the United States but popular in Europe. It features .02, .04, and .06 tapers. The cross sections have a triangular shape, with sharp cutting edges and no radial lands. This makes for a relatively solid instrument core and active cutting ability. The overall manufacturing quality seems high, with minimal metal flash and rollover.
FlexMaster files have rounded, passive tips; the tip diameters range from 0.15 to 0.7 mm for .02 instruments and 0.15 to 0.4 mm for.04 and .06-tapered files. In addition to the standard set, the Intro file is available, which has a .11 taper and a 9-mm cutting part. The instruments are marked with milled rings on the instrument shaft, and the manufacturer provides a system box that indicates sequences for narrow, medium-size, and wide canals.
Several studies indicate that the FlexMaster allows centered preparations in both constricted and wider canals and that it performed on par with other systems. Clinical studies confirmed that the FlexMaster showed superior shaping characteristics compared with K-files. Also, novice dental students were able to shape plastic blocks successfully with the FlexMaster after a short training period. Tested in a well-described model of simulated canals, FlexMaster instruments led to few aberrations but took longer than preparations with RaCe files. Moreover, FlexMaster appeared to be less effective than RaCe in removing dye from the walls of simulated canals prepared to size #30 but were more effective than ProFile.
RaCe, BioRaCe, BT Race
The RaCe file has been manufactured since 1999 by FKG and was later distributed in the United States by Brasseler (Savannah, GA). The name, which stands for r eamer with a lternating c utting e dges, describes just one design feature of this instrument. Light microscopic imaging of the file shows flutes and reverse flutes alternating with straight areas; this design is aimed at reducing the tendency of files to thread into a root canal. Cross sections are triangular or square for .02 instruments with size #15 and #20 tips. The lengths of cutting parts vary from 9 to 16 mm.
The surface quality of RaCe instruments has been modified by electropolishing, and the two largest files (size #35, .08 taper and size #40, .10 taper) are also available in stainless steel. The tips are round and noncutting, and the instruments handles are color-coded and marked by and milled rings. RaCe instruments have been marketed in various packages to address small and large canals; recently they are sold as BioRaCe, purportedly to allow larger preparation sizes, with an emphasis on the use of .02 tapered instruments.
Few results of in vitro experiments comparing RaCe to other contemporary rotary systems are available, Canals in plastic blocks and in extracted teeth were prepared by the RaCe system with less transportation than with ProTaper files. In another study, ProTaper and RaCe performed similarly when canals were prepared to an apical size #30. When preparing to a size #40, RaCe files prepared canals rapidly and with few aberrations or instrument deformities. The newer BioRaCe instrument sequence utilizes .02 tapered instruments to promote larger apical sizes. As with any rotary system, this is also possible in a hybrid hand-rotary technique. BioRace instruments prepared S-shaped canals in plastic blocks (to size #40) similarly to ProTaper and MTwo but were superior when combined with S-Apex. In a clinical study, Rocas and colleagues found no significant differences between NiTi hand file preparation and BioRace regarding the reduction of bacterial load. A new variant, BT RaCe, has been introduced and incorporates different tip designs, as well as different sequences.
EndoSequence
The EndoSequence rotary instrument is produced by FKG in Switzerland and marketed in the United States by Brasseler. This instrument adheres to the conventional length of the cutting flutes, 16 mm, and to larger tapers, .04 and .06, to be used in a crown-down approach. The overall design, including the available tapers and cross sections, is thus similar to many other files; however, the manufacturer claims that a unique longitudinal design (called alternating wall contact points [ACP]) reduces torque requirements and keeps the file centered in the canal. It also has varying, comparatively small helical angles. Another feature of the EndoSequence design is an electrochemical treatment after manufacturing, similar to that of RaCe files, resulting in a smooth, polished surface. This is thought to promote better fatigue resistance, hence a rotational speed of 600 rpm is recommended for EndoSequence. Most in vitro results, however, suggest that EndoSequence is not superior to other files in cyclic fatigue resistance.
Twisted File
In 2008, SybronEndo presented the first fluted NiTi file manufactured by plastic deformation, a process similar to the twisting process that is used to produce stainless steel K-files: the Twisted File (TF). According to the manufacturer, a thermal process allows twisting during a phase transformation into the so-called R-phase of nickel-titanium. The instrument is currently available with a size tip from #25 to #50 and in tapers from .04 to .12.
The unique production process is thought to result in superior physical properties; indeed, early studies suggested significantly better fatigue resistance when size #25 .06 taper Twisted Files were compared to K3 instruments of the same size GTX instrument. Moreover, as determined by bending tests according to the ISO norm for hand instruments, 3630-1, Twisted Files size #25 .06 taper were more flexible than ProFiles of the same size. Others found similar levels for fatigue resistance for TF and Profile of similar size.
A more recent development for TF is the use of an electric motor that allows different file movement, both continuous rotation and reciprocation, depending on the clinical situation (TF Adaptive, SybronEndo).
ProFile Vortex
Profile Vortex files are manufactured from NiTi. Two versions are on the market, one made from M-Wire and another from so-called blue wire (Vortex Blue, which showed greater resistance to cyclic fatigue and increased torque resistance), and they have varying helical angles to counteract the tendency of nonlanded files to thread into the root canal. Vortex instruments are recommended to be used at 500 rpm; higher rotational speed results in less torque generated. Canal preparation with ProFile Vortex in vitro was similar to other rotary instruments. Vortex instruments are available in sizes #15 to #50 and in .04 and .06 tapers.
MTwo
This instrument, originally sold in Italy by Sweden e Martina, was put onto the European market in 2004. The instrument has a two-fluted S-shaped cross section. The original strategy allowed for three distinct shaping approaches after the use of a basic sequence with tip sizes from #10 to #25 and tapers ranging from .04 to .06. Subsequent enlargement was meant to create apical sizes up to #40 .04 or, alternatively, to #25 .07 or to larger apical sizes with so-called apical files. MTwo is a well-researched and cutting-efficient instrument; clinically it is an example for the so-called single length technique. Canal shapes with MTwo were similar to other contemporary root canal instruments, either in rotation or reciprocation.
Summary
The market share for rotary files without radial lands continues to expand because of perceived higher efficacy. The overall incidence of clinically relevant preparation errors (details are discussed later) appears to be low, in spite of more aggressive cutting by files without radial lands. Instrument fractures remain a concern, as does the tendency of continuously rotating instruments to thread or pull into the canal, specifically as working length is approached.
Group III, Special Cases
WaveOne, Reciproc
A way to mitigate problems with continuous rotation (e.g., taper lock, fatigue fracture, threading-in) is to return to reciprocation, which had been used decades ago (e.g., in the Giromatic handpiece). A case report described this approach using ProTaper F2 in reciprocation. Based on experiments evaluating the maximal rotational angle before plastic deformation for the selected instrument, a forward angle of 144 degrees followed by a reverse rotation of 72 degrees was recommended. This cycle continues at 400 rpm until working length is reached.
Subsequently two instruments specifically designed for reciprocation were brought to the market: WaveOne (Tulsa Dentsply Dental Specialties) and Reciproc (VDW, currently not available in the United States). WaveOne instruments are available in three tip sizes, #21, #25, and #40, with tapers of .06 and .08, respectively. Corresponding sizes for Reciproc are tips of #25, #40, and #50 with tapers of .08, .06, and .05, respectively. Both instruments feature variable tapers that are largest toward the tips. The main WaveOne cross section is triangular, similar to ProTaper, whereas Reciproc is a two-fluted file with a design similar to MTwo.
Special motors are used for both systems to provide reciprocation action with alternating counterclockwise and clockwise rotations of about 150 to 170 and 30 to 50 degrees, respectively.
Both files are machined with left-leaning flutes; therefore, the cutting direction for both is clockwise. One problem that may occur with this design is the transportation of dentin debris into the apical area, rather than moving debris coronally. There is mixed evidence for this phenomenon in vitro. Clinically, frequent careful cleaning of the cutting blades with a moist gauze is recommended.
The shaping ability of these systems, according to current in vitro data, seems similar to that of established systems with continuous rotation.
Self-Adjusting File
The self-adjusting file (SAF; ReDent-Nova) represents a different approach, both in file design and mode of operation. The file is really a cylindrical, hollow device, designed as a thin-walled NiTi lattice with a lightly abrasive surface (see Fig. 6-26 ). An initial glide path is established up to a #20 K-file to allow the insertion of the SAF file. The file is proposed to be compressed from its 1.5 mm diameter into dimensions equivalent to those of a #25 K-file. It is operated with a handpiece that generates in-and-out vibrations (4000 per minute) and 0.4 mm amplitudes. As noted, the file is hollow, which allows for continuous irrigation through the file while operated in the root canal.
In vitro data for this system suggest that indeed more wall contact is made compared to rotary files, resulting in better debridement and antimicrobial efficacy. Shaping quality is also on par with rotary instruments.
Endo-Eze
The Giromatic handpiece (MicroMega), a rotary instrument system in use since 1969, delivers 3000 quarter-turn reciprocating movements per minute. Special rasps and barbed broaches made from stainless steel were most often used in Giromatic handpieces, but K-type and H-type instruments may also be used. The preparation quality with this system was insufficient in curved canals, and the technique fell into disregard.
The Endo-Eze file system (Ultradent, South Jordan, UT) is a more recently introduced addition using a similar motion, provided by special equipment or an original Giromatic handpiece. The set has four engine-driven instruments that are designed to clean the middle third of the canal. The sizes and tapers are #10 and #13, with tapers ranging from 0.02 to 0.04. In this system, the use of stainless steel hand instruments is suggested for the apical third of the canal.
Preparation quality in curved canals appeared to be inferior to NiTi rotaries. In straight canals, Endo-EZE performed similar to FlexMaster.
Sonic and Ultrasonic Instruments
An alternative way of instrumenting root canals was introduced when clinicians became able to activate files by electromagnetic ultrasonic energy. Piezoelectric ultrasonic units are also available for this purpose. These units activate an oscillating sinusoidal wave in the file with a frequency of about 30 kHz.
Two types of units, ultrasonic and sonic, are marketed. Ultrasonic devices, which operate at 25 to 30 kHz, include the magnetostrictive Cavi-Endo (Dentsply Caulk, Milford, DE), the piezoelectric ENAC (Osada, Tokyo), the EMS Piezon Master 400 (Electro Medical Systems, Vallée de Joux, Switzerland), and the P5 Neutron (Acteon Satelec, Merignac Cedex, France) ( Fig. 6-29 ). Sonic devices, which operate at 2 to 3 kHz, include the Sonic Air MM 1500 (MicroMega), the Megasonic 1400 (Megasonic Corp, House Springs, MO), and the Endostar (Syntex Dental Products, Valley Forge, PA).

Ultrasonic device holders can fit regular types of instrument blanks (e.g., K-files), whereas sonic devices use special inserts known as Rispi-Sonic, Shaper-Sonic, Trio-Sonic, or Heli-Sonic files.
Although similar in function, piezoelectric units have some advantages over the magnetostrictive systems. For example, piezoelectric devices generate little heat, so no cooling is needed for the handpiece. Magnetostrictive systems, however, generate considerable heat, and a special cooling system is needed in addition to the cooling effect provided by root canal irrigation. Working without water-cooling becomes essential when using an operating microscope, because the water spray may obstruct visualization.
The piezoelectric transducer transfers more energy to the file than does the magnetostrictive system, making it more cutting efficient. The file in an ultrasonic device vibrates in a sinus wave–like fashion. A standing wave has areas with maximal displacement (i.e., antinodes) and areas with no displacement (i.e., nodes). The tip of the instrument exhibits an antinode. If powered too high, especially with no contact with the canal wall, the instrument may break because of the intense vibration. Therefore, files must be used only for a short time, must remain passive within the canal, and the power must be controlled carefully. The frequency of breakage in files used for longer than 10 minutes may be as high as 10%, and the breakage normally occurs at the nodes of vibrations. Ultrasonic devices have been linked to a higher incidence of preparation errors and to reduced radicular wall thickness.
Summary
An abundance of NiTi systems is currently on the market. Table 6-1 lists these instruments systematically and illustrates some of the most relevant properties. Most systems included in Table 6-1 have files with tapers greater than the .02 stipulated by the ISO norm. Differences exist in tip designs, cross sections, and manufacturing processes. In vitro tests have continued to identify the effect of specific designs on shaping capabilities and fracture resistance. A desirable goal is the instrumentation of more canal surface, in an effort to make biofilm more susceptible to subsequent chemical disinfection. At the same time, instrumentation paradigms that conserve more dentin appear desirable to promote long-term function. Meaningful differences in clinical outcomes in regard to various specific design variations have yet to be ascertained.
Motors
Motors for rotary instruments have become more sophisticated since the simple electric motors of the first generation in the early 1990s ( Fig. 6-30, A ). Electric motors with gear reduction are most suitable for rotary NiTi systems, because they ensure a constant rpm level and constant torque. They can also be programmed to provide alternative rotation patterns, for example, reciprocation with freely selectable angles of rotation. Electric motors often have presets for rpm and torque and are capable of delivering torques much higher than those required to break tips. Some authors think that torque-controlled motors (see Fig. 6-30, B to D ) increase operational safety. Others have suggested that such motors may mainly benefit inexperienced clinicians. These motors probably do not reduce the risk of fracture caused by cyclic fatigue, and even if the torque is below the fracture load at D3, a fracture at a smaller diameter (D2) is still possible.

To complicate matters further, a differential exists between torque at failure at D3 and the working torque needed to operate an instrument effectively ( Fig. 6-31 and Box 6-2 ). In many cases the working torque is greater than the torque required to fracture the instrument’s tip.

For rotary instrument tips, susceptibility to breakage is governed by the quotient of torque needed to fracture divided by working torque. Simply put, the larger the value, the safer the file.
This differential between working torque and tip fracture load is especially large, with files having a taper equal to or greater than .06; therefore, these files are rather ineffective in most torque-controlled motors. Future motors will likely offer more microprocessor controlled features—for example, information about the specific instrument being used, such as the presets and the usage history.
Certain motors have built-in apex locators (see Fig. 6-30, D ). This type of motor may be programmed to stop a rotary instrument when working length is reached or go into reverse gear. Similar changes in movement can occur in an adaptive fashion, depending on the torsional load that the instrument experiences in the root canal. The TF Adaptive motor (SybronEndo) is an example for this technology.
Some of the factors, besides the motor itself, that may influence the incidence of fracture in engine-driven NiTi rotary instruments are lubrication, specific instrument motion, and speed of rotation. It cannot be overemphasized that NiTi rotary instruments should be used only in canals that have been flooded with irrigant. Although lubricants such as RC-Prep (Premier Dental Products Co., Plymouth Meeting, PA) and Glyde (Dentsply Maillefer) have been recommended in the past, their benefit may be restricted to plastic blocks and less relevant when rotary instruments engage dentin surfaces. Moreover, experimental data for dentin suggest that the use of lubricants fails to reduce torque values during simulated canal preparation. Finally, because of chemical interactions between NaOCl and ethylenediamine tetra-acetic acid (EDTA), alternating irrigants and using lubricants that contain EDTA may even be counterproductive. Details in irrigation solution interactions are described later in this chapter.
For instrument motion, some manufacturers recommend a pecking, up-and-down motion. This not only prevents threading in of the file, it is also thought to distribute stresses away from the instrument’s point of maximum flexure, where fatigue failure would likely occur. However, such in-and-out movements did not significantly enhance the life span of ProFile .04 taper or GT rotary instruments rotated around a 5-mm radius cylinder with a 90-degree curve. Furthermore, large variations were noted in the lengths of the fractured segments. This suggests that ductile fractures may originate at points of surface imperfections. Files made of more martensitic alloy tend to work better with longer sweeping or brushing strokes. The notion of “brushing” is not directly related to a paintbrush motion, as this would predispose a file to bending and subsequent fatigue. It rather refers to stroking against the wall away from the danger zone, the inner curvature of a curved root.
Rotational speed may influence instrument deformation and fracture. Some studies indicated that ProFile instruments with ISO-norm tip diameters failed more often at higher rotational speed, whereas other studies did not find speed to be a factor. For Vortex, 300 rpm reduced the generated torque and force; this higher rpm preset did not result in an increase of instrument fractures in vitro.
Clinicians must fully understand the factors that control the forces exerted on continuously rotating NiTi instruments ( Box 6-3 ). To minimize the risk of fracture and prevent taper lock, motor-driven rotary instruments should not be forced in an apical direction. Similarly, acute apical curves limit the use of instruments with higher tapers because of the risk of cyclic fatigue. The incidence of instrument fracture can be reduced to an absolute minimum if clinicians use data from well-designed torque and stress studies. Adequate procedural strategies, such as an adequate glide path, a detailed knowledge of anatomic structures, with avoidance of extreme canal configurations, and specific instrumentation sequences may also improve shaping results.
- ◆
Clinician’s handling (most important)
- ◆
Combination of torsional load, bending, and axial fatigue
- ◆
Root canal anatomy
- ◆
Manufacturing process and quality
Certain procedures have evolved for removing fractured instruments from root canals (see Fig. 6-25 ); these are discussed in detail elsewhere in this book (see Chapters 8 and 19 ). Most of those methods require the use of additional equipment, such as a dental operating microscope and ultrasonic units. However, the best way to deal with instrument fracture is prevention. An understanding of the anatomy of the root canal system, together with a clear plan for selecting, sequencing, and using shaping instruments, can certainly help prevent procedural mishaps.
Steps of Cleaning and Shaping
Access: Principles
Access cavity preparation is essential for root canal treatment and is described in detail elsewhere in this book ( Chapter 5 ). It should be emphasized at this point that mishaps during access (e.g., perforation) significantly affect the long-term outcome of a root canal treated tooth. Overenlargement or gouging during access significantly reduces structural strength and may lead to root fracture and nonrestorable conditions.
Rationally, the use of a cylindrical diamond bur, followed by a tapered fluted bur with noncutting tip and perhaps a small round bur, is recommended ( Fig. 6-32 ). One experiment suggested that more conservative access cavities, in particular in premolars, may increase fracture resistance and permit similar shaping outcomes. The use of ultrasonically powered tips under magnification is aimed at achieving ideal access cavities ( Fig. 6-33 ), including discovery and perhaps relocation of the canal orifices.


Coronal Preflaring
The extension of an access cavity into the coronal-most portion of the root canal is called coronal flaring. If a canal is constricted, mineralized, or difficult to access, flaring the coronal portion prior to any deep entry into the root canal is beneficial. This canal modification should be preceded by a scouting step, in which a small (e.g., size #10) K-file is passively placed several millimeters into the root canal. Tools for preflaring include Gates Gliddens ( Fig. 6-34 ) and dedicated NiTi instruments ( Fig. 6-35 ). Both step-back and step-down sequences have been recommended for Gates Gliddens, whereas NiTi rotaries are typically used in a crown-down sequence. More recently, specific orifice shaping rotaries have been developed that are used as a single instrument, rather than a sequence.


The use of laterally cutting NiTi rotaries allows the clinician to modify the root canal orifice to form a receptacle for subsequent instrumentation. This is related to removal of coronal mineralization and to relocation of the canal pathway by removal of dentin overhangs. For example, a second mesiobuccal canal typically exits the pulp chamber toward the mesial under a dentin shelf and then curves toward the distal. It is this curve in a small canal that often leads to formation of a ledge, which in turn prevents shaping to length.
Preenlargement of the coronal half to two thirds of a root canal allows files unimpeded access to the apical one third and gives the clinician better tactile control in directing small, adequately precurved negotiating files ( Fig. 6-36 ).

One of the purported benefits of early coronal flaring is access of disinfecting irrigation solutions, but this has not been confirmed in experiments. On the other hand, a documented benefit of coronal flaring is mitigation of working length changes during canal preparation.
Comparatively little is known about the efficacy of individual flaring instruments. One study compared the cutting efficacy of radial-landed and triangular cross sections. The latter were shown to be more cutting efficient compared to radial-landed design; somewhat surprisingly, flexible martensitic alloy was cutting more rapidly compared to conventional NiTi alloys.
Patency File
A patency file is a small K-file (usually a size #10 or #15) that is passively extended slightly beyond the apical foramen. The use of a patency file has been suggested for most rotary techniques. This step is thought to remove accumulated debris, to help maintain working length, and to translate into greater clinical success. One concern with the patency file was that instead of having a cleaning effect, the file would push contaminated debris through the foramen. However, an in vitro study suggested that the risk of inoculation was minimal when canals were filled with NaOCl. Maintaining patency throughout an endodontic procedure does not lead to an increase in posttreatment symptoms. Only initial clinical evidence exists favoring the use of a patency file; however, experience suggests that this technique involves relatively little risk and provides some benefit as long as small files are used carefully.
Working Length Determination
Devices
Radiographs, tactile sensation, the presence of moisture on paper points, and knowledge of root morphology have been used to determine the length of root canal systems. Following earlier experiments by Custer, Sunada developed the first commercialized electronic apex locator, suggesting that the apical foramen could be localized using a direct electric current. Currently, the electronic apex locator is considered an accurate tool for determining working length. One study reported that the use of electronic apex locators in a dental student clinic resulted in a higher quality of obturation length control and an overall reduction in the number of radiographs taken. However, these devices must not be considered flawless, because several variables are known to affect their accuracy. For example, immature roots can present problems. Once roots mature (i.e., form a narrow apical foramen) and instruments are able to contact the canal walls, an electronic apex locator’s accuracy greatly improves. Some investigators have found no statistical difference between roots with vital and necrotic tissue. Because apical root resorption is prevalent in necrotic cases with longstanding apical lesions, it may be concluded that apical resorption does not have a significant effect on the accuracy of electronic apex locators.
Some clinicians have advocated the use of the electronically determined working length in lieu of working length estimations using the placement of a file in the canal and a radiograph. However, combined use of both of these techniques has been shown to result in greater accuracy. Furthermore, radiographs may add essential anatomic information that could be missed if electronic apex locators are used exclusively.
The first two generations of electronic apex locators were sensitive to the contents of the canal and irrigants used during treatment. The development of an algorithm called the ratio measurement method distinguished the third generation of apex locators. To arrive at this method, the impedance of the canal was measured with two current sources of different frequencies, and a quotient was determined using the electrical potentials proportional to each impedance. This study found that electrolytes did not have a significant effect on the accuracy of the unit. This means that clinically canal contents need not be dried, but fluids in contact with crowns or coronal metallic restoration materials could conduct currents and lead to false results.
Some third-generation apex locators are the Endex Plus, or Apit (Osada, Los Angeles, CA), the Root ZX (J. Morita, Kyoto, Japan), and the Neosono Ultima EZ (Acteon Satelec). The Endex Plus device uses 1 and 5 kHz and provides apex location based on subtraction. The Root ZX emits currents at frequencies of 8 and 0.4 kHz and provides apex location based on the resulting quotient. In addition to latest model apex locators, manufacturers have developed smaller models ( Fig. 6-37, B ).

Apex locators are generally safe to use; however, manufacturers’ instructions state that they should not be used on patients with pacemakers without consulting the patient’s cardiologist. However, when connected directly to cardiac pacemakers in vitro, electronic apex locators did not interfere with the function of the pacemaker, and they did not interfere with the functioning of any of the cardiac devices tested in a clinical study under electrocardiogram monitoring.
Strategies
Anatomic studies and clinical experience suggest that typically teeth are 19 to 25 mm long. Most clinical crowns are approximately 10 mm long, and most roots range from 9 to 15 mm in length. Roots, therefore, can be divided into thirds that are 3 to 5 mm long. An important issue in root canal treatment is the apical end point of the prepared shape in relation to the apical anatomy. Traditional treatment has held that canal preparation and subsequent obturation should terminate at the apical constriction , the narrowest diameter of the canal ( Fig. 6-38 ). This point is thought to coincide with the cementodentinal junction (CDJ) (see Chapter 12 ) and is based on histologic sections and ground specimens. However, the position and anatomy of the CDJ vary considerably from tooth to tooth, from root to root, and from wall to wall in each canal. Moreover, the CDJ cannot be located precisely on radiographs. For this reason, some have advocated terminating the preparation in necrotic cases at 0.5 to 1 mm short of the radiographic apex and 1 to 2 mm short in cases involving irreversible pulpitis. Although there is no definitive validation for this strategy at present, well-controlled follow-up studies seem to support it.

Working to shorter lengths may lead to the accumulation and retention of debris, which in turn may result in apical blockage ( Fig. 6-39 ). If the path to the apex is blocked, working to short lengths may contribute to procedural errors such as apical perforations and fractured instruments. Such obstacles (which consist of collagen fibers, dentin mud, and, most important, residual microbes) in apical canal areas are a major cause of persistent or recurrent apical periodontitis, or post-treatment disease (also see Chapters 14 and 15 ).

Using an electronic apex locator has helped clinicians identify the position of apical foramina more accurately and allow safe canal shaping as close as 0.5 mm to the canal terminus.
Canal Enlargement/Preparation
Rationale
Like the position of the apical constriction, apical diameters are difficult to assess clinically. Some have recommended gauging canal diameters by passing a series of fine files apically until one fits snugly. However, such an approach is likely to result in underestimation of the diameter. This is a crucial point because the initial canal size is a major determinant for the desired final apical diameter.
An ongoing debate exists between those who prefer smaller apical preparations combined with tapered shapes and those who favor larger apical preparations for better removal of infected dentin and to allow irrigation fluids access to the apical areas ( Table 6-2 ). Both sides stress the importance of maintaining the original path of the canal during preparation; otherwise, bacteria in the apical one-third of the root canal may not be reached by sufficient amounts of an antimicrobial agent. Investigators demonstrated a higher percentage of bacterial elimination in single-root canal systems by using a combination of significant enlargement of the apical third and NaOCl irrigation. Preparation errors (e.g., zips, canal transportation) may occur in larger preparations, with both stainless steel and NiTi instruments ( Fig. 6-40 ).
Root Canal Preparation | Benefits | Drawbacks |
---|---|---|
Narrow apical size | Minimal risk of canal transportation and extrusion of irrigants or filling material Can be combined with tapered preparation to counteract some drawbacks Less compaction of hard tissue debris in canal spaces |
Little removal of infected dentin Questionable rinsing effect in apical areas during irrigation Possibly compromised disinfection during interappointment medication Not ideal for lateral compaction |
Wide apical size | Removal of infected dentin Access of irrigants and medications to apical third of root canal |
Risk of preparation errors and extrusion of irrigants and filling material Not ideal for thermoplastic obturation |

Thorough disinfection of the apical part of a root canal is essential, because this area is most likely to harbor residual intraradicular bacteria. Wider apical preparations remove potentially infected dentin, allowing the delivering needle and subsequently the antimicrobial irrigant to penetrate the root canal more deeply.
A study investigating rotary NiTi files of three tapers (.06, .08, and .10) with file tips in sizes #20, #30, and #40 showed that size #20 instruments left significantly more debris in the apical third compared with size #40 instruments. On the other hand, a study in which half the samples were prepared to a size #25 file and the other half to a size #40 file found no statistically significant difference in bacterial growth after instrumentation, with no growth observed after 1 week of treatment with a Ca(OH) 2 dressing. Step-down sequences with additional apical enlargement to ISO size #35 and a serial step-back technique with no apical enlargement were compared, using NaOCl and EDTA as irrigants. Here, no significant difference was detected in colony-forming units with or without apical enlargement. These researchers concluded that dentin removal in the apical third might be unnecessary if a suitable coronal taper is achieved.
Despite the disagreement over the appropriate width of a preparation (see Table 6-2 ), these studies suggest that root canal preparations should be confined to the canal space, should be sufficiently wide, and should incorporate the original root canal cross sections (see Fig. 6-4 ).
Traditional cleaning and shaping strategies (e.g., the step-back technique) focused immediate preparation of the apical third of the root canal system, followed by various flaring techniques to facilitate obturation. In an attempt to reach the canal terminus, the clinician first selected a small file, placed an appropriate curve on the instrument, and then tried to work the file to full length. If the terminus could not be reached, the file was removed and, after irrigation, either the same file or a smaller one was inserted. However, not infrequently, full length was not obtained, either because of blockage or because of coronal binding.
Coronal binding is caused by overhangs at the orifice level and when the canal is less tapered than an instrument, making it bind coronally. Moreover, a straight root often contains a curved canal, such as buccal and lingual curvatures that cannot be seen on radiographs. In addition, passing a precurved negotiating file through a coronally tight canal will straighten the instrument.
Various instrumentation sequences have been developed for hand and rotary instruments; these are discussed later in this chapter. However, the shape of the access cavity is the prerequisite that must be optimized before any canal preparation can take place (see Chapter 5 ).
As stated previously, preparation of an adequate access cavity (see Fig. 6-32 ) may involve the use of a cylindrical diamond or fissure bur, a safety-ended drill for additional enlargement, and round burs to remove overhangs on outward strokes. The access cavity shape must allow instruments unimpeded access to the middle third of the root canal system. Ultrasonically powered instruments used under an operating microscope greatly facilitate removal of mesial dentin shelves in mandibular molars (see Fig. 6-33, A and B ) and other teeth. Preexisting restorations allow for ideal access cavities that serve as reservoirs of irrigants (see Fig. 6-33, C ).
Basic cleaning and shaping strategies for root canal preparation can be categorized as crown-down, step-back, apical widening, and hybrid techniques. In a crown-down approach, the clinician passively inserts a large instrument into the canal up to a depth that allows easy progress. The next smaller instrument is then used to progress deeper into the canal; the third instrument follows deeper again, and this process continues until the terminus is reached. Both hand and rotary instruments may be used in a crown-down manner. However, instrument sets with various tip diameters and tapers allow the use of either decreasing tapers or decreasing diameters for apical progress. Debate continues as to which of those strategies is superior for avoiding taper lock; currently, no compelling evidence favors either of them.
In the step-back approach, working lengths decrease in a stepwise manner with increasing instrument size. This prevents less flexible instruments from creating ledges in apical curves while producing a taper for ease of obturation.
As discussed previously, the aim of apical widening is to fully prepare apical canal areas for optimal irrigation efficacy and overall antimicrobial activity. Apical enlargement has been broken down into three phases: preenlargement, apical enlargement, and apical finishing.
Most rotary techniques require a crown-down approach to minimize torsional loads, and they reduce the risk of instrument fracture. Used sequentially, the crown-down technique can help enlarge canals further. All basic techniques described so far may be combined into a hybrid technique to eliminate or reduce the shortcomings of individual instruments.
Root canal preparation can be broken down into a series of steps that parallel the insertion depths of individual instruments. Anatomic studies and clinical experience suggest that most teeth are 19 to 25 mm long. Most clinical crowns are approximately 10 mm long, and most roots range from 9 to 15 mm in length. Roots, therefore, can be divided into thirds that are 3 to 5 mm long.
Provided adequate tools are used and the access cavity design is appropriate, excessive thinning of radicular structures can be avoided (see Fig. 6-5 ). Vertical root fractures and perforations are possible outcomes of excessive removal of radicular dentin in zones that have been termed danger zones . Overenthusiastic filing, for example, may lead to more procedural errors (see Figs. 6-22 and 6-40 ). On the other hand, ideal preparation forms without any preparation errors and with circular incorporation of the original canal cross sections may be achieved with suitable techniques (see Fig. 6-4 ).
Dedicated NiTi instruments have been introduced or suggested for coronal preenlargement, such as the ProFile orifice shapers, GT accessory files, the ProTaper SX, the FlexMaster Intro file, and the size #40, .10 taper or size #35, .08 taper RaCe files. These instruments are better suited than GGs and safer for more difficult cases (see Figs. 6-34 and 6-35 ).
Techniques
Standardized Technique
The standardized technique adopts the same working length definition for all instruments introduced into a root canal. It therefore relies on the inherent shape of the instruments to impart the final shape to the canal. Negotiation of fine canals is initiated with lubricated fine files in a so-called watch-winding movement. These files are advanced to working length and worked either in the same hand movement or with “quarter-turn-and-pull” until a next larger instrument may be used. Conceptually, the final shape should be predicted by the last instrument used ( Fig. 6-41 ). A single matching gutta-percha point may then be used for root canal filling. In reality, this concept is often violated: curved canals shaped with the standardized technique will be wider than the last used instrument, exacerbated by the pulling portion of the hand movement. Moreover, adequate compaction of gutta-percha in such small a taper (~.02) is difficult or impossible (see Chapter 7 ).

The standardized technique was hampered by the very standardization of the instruments used to perform the technique. Specifically, the similar size increment by 0.05 mm up to size 55 was clinically more difficult to achieve moving from size #10 to size #15, compared to the step from size #40 to #45. In very small files (sizes #6 to #10), the problem is partly resolved by several key points: (1) apical dimensions are such that a size #6 file does not significantly remove dentin other than in severely calcified cases; (2) a size #8 file taken 0.5 to 1 mm long to establish patency (discussed later in the chapter) contacts the desired end point of the preparation with a diameter approaching the tip size of a #10 file; (3) similarly, placing a size #10 file just minutely through the foramen eases the way for passive insertion of the subsequent #15 file to full length.
Step-Back Technique
Realizing the importance of a shape larger than that produced with the standardized approach, one investigator suggested the step-back technique, incorporating a stepwise reduction of the working length for larger files, typically in 1-mm or 0.5-mm steps, resulting in flared shapes with 0.05 and 0.10 taper, respectively ( Fig. 6-42 ). Incrementally reducing the working length when using larger and stiffer instruments also reduced the incidence of preparation errors, in particular in curved canals. This concept appeared to be clinically very effective.

Although the step-back technique was primarily designed to avoid preparation errors in curved canals, it applies to the preparation of apparently straight canals as well. Several modifications of the step-back technique have been described over the years. Another investigator advocated the insertion of progressively larger hand instruments as deep as they would passively go in order to explore and provide some enlargement prior to reaching the working length.
Step-Down Technique
Other investigators described a different approach. They advocated shaping the coronal aspect of a root canal first, before apical instrumentation commenced. This technique is intended to minimize or eliminate the amount of necrotic debris that could be extruded through the apical foramen during instrumentation. Moreover, by first flaring the coronal two thirds of the canal, apical instruments are unimpeded through most of their length. This in turn may facilitate greater control and less chance of zipping near the apical constriction.
Crown-Down Technique
Numerous modifications of the original step-down technique have been introduced, including the description of the crown-down technique. The more typical step-down technique includes the use of a stainless steel K-file exploring the apical constriction and establishing working length. In contrast, a crown-down technique relies more on coronal flaring and then determination of the working length later in the procedure.
To ensure penetration during step-down, one may have to enlarge the coronal third of the canal with progressively smaller GG drills or with other rotary instruments. Irrigation should follow the use of each instrument and recapitulation after every other instrument. To properly enlarge the apical third and to round out ovoid shape and lateral canal orifices, a reverse order of instruments may be used starting with a size #20 (for example) and enlarging this region to a size #40 or #50 (for example). The tapered shape can be improved by stepping back up the canal with larger instruments, bearing in mind all the time the importance of irrigation and recapitulation.
The more typical crown-down, or double-flare, technique consisted of an exploratory action with a small file, a crown-down portion with K-files of descending sizes, and an apical enlargement to size #40 or similar. The original technique included stepping back in 1-mm increments with ascending file sizes and frequent recapitulations with a small K-file and copious irrigation. It is further emphasized that significant wall contact should be avoided in the crown-down phase to reduce hydrostatic pressure and the possibility of blockage. Several studies demonstrated more centered preparations in teeth with curved root canals shaped with a modified double-flare technique and Flex-R files compared to shapes prepared with K-files and step-back technique. A double-flare technique was also suggested for ProFile rotary instruments.
Balanced Force Technique
Regarding hand movements, a general agreement exists that the so-called balanced force technique creates the least canal aberrations with K-files. This technique has been described as a series of rotational movements for Flex-R files, but it can also be used for K-files and other hand instruments such as GT hand files. Many explanations have been offered for the obvious and undisputed efficacy of the balanced force approach, but general agreement exists that it provides excellent canal-centering ability, superior to other techniques with hand instruments.
The balanced force technique involves three principle steps. The first step (after passive insertion of an instrument into the canal) is a clockwise rotation of about 90 degrees to engage dentin ( Fig. 6-43 ). In the second step, the instrument is held in the canal with adequate axial force and rotated counterclockwise to break loose the engaged dentin chips from the canal wall; this produces a characteristic clicking sound. Classically, in the third step, the file is removed with a clockwise rotation to be cleaned; however, because files used with the balanced force technique are not precurved, every linear outward stroke essentially is a filing stroke and may lead to some straightening of the canal path. Therefore, in many cases, the clinician may advance farther apically rather than withdrawing the file, depending on the grade of difficulty.

Rotary Instrumentation
NiTi rotary instruments are an invaluable adjunct in the preparation of root canals, although hand instruments may be able to enlarge some canals just as efficiently when used in appropriate sequences. Hand instruments should be used only after coronal preenlargement (e.g., with GG drills). After preenlargement, the access cavity and canals are flooded with irrigant, and a precurved scouting file is advanced into the canal. A lubricant when using hand instruments can help prevent apical blockage in this early stage. Once the working length has been established (aided by an electronic apex locator and radiographically verified), apical preparation to begins to facilitate a glide path for subsequent rotary instrumentation ( Fig. 6-44 ).

The term glide path has been used in endodontics since the early 2000s and relates to securing an open pathway to the canal terminus that subsequent engine-driven instruments can follow. The typical minimum glide path is a size #15 to #20 K-file and should be confirmed with a straight, not precurved file. Various small engine-driven instruments were introduced to simplify this process, such as Pathfiles (Dentsply Maillefer), Scout RaCe (FKG Dentaire SA, La Chaux‑de‑Fonds Switzerland), and G-file (MicroMega). Copious irrigation and frequent recapitulations with a smaller file to working length may be required, and in some instances, clinicians must devise creative strategies using small crown-down or step-back sequences.
Fig. 6-45 illustrates the development of two different shapes in the mesial root canals of a mandibular molar, clearly showing that substantial areas of the root canal surface in either case are not instrumented, even when apical size #50 or 0.09 taper are reached (see the red areas in Fig. 6-45, G and I ).

Specific NiTi Instrumentation Techniques
Crown Down
This was the dominant approach for many years and is still being used, for example, for ProFile and several others (ProFile Vortex, HERO 642, K3, and FlexMaster). It must be noted that the manufacturers’ instructions for these systems vary somewhat, and the instructions for GT rotary, RaCe, and the Twisted File vary even more. Clinicians should always read the manufacturers’ instructions for details on working with those instruments.
Working length is determined after any coronal preenlargement , and an open glide path is secured with K-files up to size #15 or #20, depending on the canal anatomy. If canal size permits, canal preparation begins with .06 taper instruments in descending tip diameters. In more difficult small canals, .06 tapers are followed by .04-tapered instruments, also with descending tip diameters. Apical preparation is performed either with multiple shaping waves, as suggested for GT rotary files, or in a step-back manner. Recapitulation with a small hand file is recommended throughout the preparation.
Single Length
The approach for ProTaper Universal and ProTaper Next instruments differs from that for many other NiTi rotary files (except MTwo, WaveOne and Reciproc) in that no traditional crown-down procedure is performed (see Fig. 6-44 ).
Size #10 and #15 hand files are passively inserted into the coronal two thirds of a root canal as path-finding files, which confirm the presence of a smooth, reproducible glide path. This step is essential for ProTaper shaping instruments, because they are mostly side cutters and have fine, fragile tips.
Shaping files S1 and S2 are then passively inserted into the scouted canal spaces, which have been filled with irrigant (preferably NaOCl). If necessary, the SX file can be used at this stage to relocate orifices or remove obstructing dentin. After each shaping file is used, the canals are reirrigated, and a size #10 file is used to recapitulate to break up debris and move it into solution. This process is repeated until the depth of the path-finding #10 or #15 file is reached.
After irrigation, the apical third is fully negotiated and enlarged to at least a size #15 K-file, and the working length is confirmed (see Fig. 6-44 ). Depending on the canal anatomy, the rest of the apical preparation can be done with engine-driven ProTaper shaping and finishing hand files. As an alternative, handles can be placed on these instruments so that they can be used for the balanced force technique.
ProTapers S1 and S2 are then carried to the full working length, still in a floating, brushing motion. The working length should be confirmed after irrigation and recapitulation with a K-file, aided by an electronic apex locator or radiographs. Because of the progressive taper and more actively cutting flutes higher up in the ProTaper design, interferences in the middle and coronal thirds are removed at this stage.
The preparation is finished with one or more of the ProTaper finishing files, used in a nonbrushing manner; because of their decreasing taper, these files will reach the working length passively. Recapitulation and irrigation conclude the procedures (see Fig. 6-44 ).
LightSpeed Technique
Since the introduction of LightSpeed instruments, the manufacturer’s guidelines have changed. This section presents a version used for the original LightSpeed rather than the LS variant ( Fig. 6-46 ).

After access and coronal preenlargement with the instrument of choice, working lengths are obtained, and apical enlargement is done with at least a loose-fitting size #15 K-file. LSX instruments are then slowly advanced to working length while registering tactile feedback. The first instrument that experiences resistance 4 mm short of working length is the final apical size; it is then advanced to working length like the smaller instruments before. The next larger instrument is placed to 4 mm short of working length. This prepares the apical 5 mm for a matching SimpliFill obturator (SybronEndo). Midroot shaping is then accomplished with sequentially larger LSX instruments. Finally, the so-called master apical rotary, or MAR, is used to recapitulate to the working length.
All LightSpeed instruments are used in the following way: a slow, continuous apical movement is used until the blade binds; after a momentary pause, the blade is advanced further with intermittent (“pecking”) motions.
Technique with the Self-Adjusting File
For this unusual file, a coronal flaring step—for example, with a G—precedes exploration with small K-files, including verification of patency and determining of working length. A glide path to a size #20 is considered appropriate; then the selected self-adjusting file is introduced into the canal with continuous irrigation through the center of the hollow file at a rate of approximately 5 ml/min. This maintains a continuous flow of active irrigant that carries with its outflow tissue debris and the dentin mud generated by the file. One file is used throughout the procedure. It is initially compressed into the root canal and gradually enlarges while cleaning and shaping the canal.
The file can rotate passively around its long axis and is guided by the operator to working length. The file is initially compressed into the root canal and gradually enlarges while cleaning and shaping the canal. The suggested time frame of instrumentation per canal dictates the final size and is approximately 3 to 4 minutes. Obturation may commence with various techniques that allow plastifying gutta-percha (see Chapter 7 ).
Hybrid Techniques
For some time, combining various NiTi preparation systems have been suggested to address certain shortcomings of current instruments ( Box 6-4 ). Although many combinations are possible, the most popular and useful ones involve coronal preenlargement followed by different additional apical preparation sequences. However, clinicians must keep in mind that anatomic variations in each canal must be addressed individually with specific instrument sequences. Most important, oval canals extend deep into the apical area, and apical foramina may in fact be oval in most cases. Naturally, a rotating file can produce a round canal at best ( Fig. 6-47 ); therefore, a strategy must be devised for adequately shaping oval canals without overly weakening radicular structure (compare Figs. 6-4 and 6-5 ). One hybrid approach completely prepared 95% or more of all such canals and resulted in extremely wide apical sizes that may be difficult to achieve with most instrument systems.
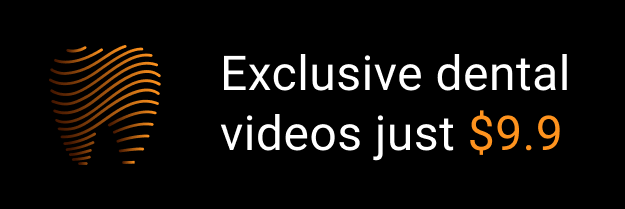