Abstract
Objectives
This study evaluated the role of endogenous dentin MMPs in auto-degradation of collagen fibrils within adhesive-bonded interfaces. The null hypotheses tested were that adhesive blends or chlorhexidine digluconate (CHX) application does not modify dentin MMPs activity and that CHX used as therapeutic primer does not improve the stability of adhesive interfaces over time.
Methods
Zymograms of protein extracts from human dentin powder incubated with Adper Scotchbond 1XT (SB1XT) on untreated or 0.2–2% CHX-treated dentin were obtained to assay dentin MMPs activity. Microtensile bond strength and interfacial nanoleakage expression of SB1XT bonded interfaces (with or without CHX pre-treatment for 30 s on the etched surface) were analyzed immediately and after 2 years of storage in artificial saliva at 37 °C.
Results
Zymograms showed that application of SB1XT to human dentin powder increases MMP-2 activity, while CHX pre-treatment inhibited all dentin gelatinolytic activity, irrespective from the tested concentration. CHX significantly lowered the loss of bond strength and nanoleakage seen in acid-etched resin-bonded dentin artificially aged for 2 years.
Significance
The study demonstrates the active role of SB1XT in dentin MMP-2 activation and the efficacy of CHX inhibition of MMPs even if used at low concentration (0.2%).
1
Introduction
Despite successful immediate bonding, the longevity of the adhesive interface remains questionable due to physical (occlusal forces, expansion and contraction stresses related to temperature changes) and chemical factors challenging the adhesive interface . The hybrid layer created by two-step etch-and-rinse adhesives containing high percentages of hydrophilic monomers, results in the formation of a porous bonded interface that behaves as a permeable membrane allowing elution of unreacted monomers, water sorption, polymer swelling and resin hydrolysis . In addition, enzymatic activity can degrade the exposed type I collagen fibrils located at the bottom of the hybrid layer due to the activation of endogenous collagenolytic factors previously identified within the dentin organic matrix .
These enzymes belong to the family of matrix metalloproteinases (MMPs) and are involved in connective tissue turn-over, degrading almost all extracellular matrix components . Recent findings indicate that MMP-2, -3, -8, -9 and -20 are present within the human dentin matrix .
Although these enzymes are involved in dentinogenesis and in caries progression mechanisms , the role of dentin MMPs in mature dentin is still unclear. Human dentin matrices exhibit variable collagenolytic and gelatinolytic activities when mixed with dentin/enamel bonding agents with different pHs . Thus, the simple application of adhesive systems on acid-etched dentin substrate can activate dentinal MMPs, initiating autolytic phenomena that will eventually affect the hybrid layer.
Chlorhexidine digluconate (CHX) has the ability to inactivate MMP-2, -8 and -9 . Despite the use of CHX as a therapeutic primer to stabilize the adhesive interface over time , the relationship between the collagenolytic activity of dentin, MMPs role in hybrid layer degradation, and the mechanism of CHX inhibition of MMPs needs to be clarified. Aqueous 2% CHX prevented much of the decline in bond strength and increased nanoleakage seen both in vitro and in vivo in deciduous and permanent teeth . Additionally, a recent in vitro study confirmed the protective role of 0.2% CHX after 1 year of storage of bonded specimens in artificial saliva, suggesting that lower concentration of CHX can be equally effective compared to 2% concentrations .
The purpose of this study was to evaluate the effect of 0.2 and 2% CHX on the adhesive–dentin interfaces created by a two-step etch-and-rinse adhesive system. The null hypotheses tested were that (1) dentin MMPs activity is not affected by adhesive or CHX application and (2) the use of CHX as a therapeutic primer does not affect bond strength and interfacial nanoleakage expression after 2 years.
2
Material and methods
2.1
Zymographic analysis
Reagents were purchased from Sigma Chemical (St Louis, MO, USA) unless otherwise specified.
Ten freshly extracted human molars were selected after patient’s informed consent was obtained under a protocol approved by the University of Trieste. Enamel, roots and remnant pulp tissue were removed and dentin powder was obtained by pulverizing liquid nitrogen-frozen coronal dentin with a steel mortar/pestle (Reimiller, Reggio Emilia, Italy). Five aliquots of 1 g each of dentin powder were obtained and treated as follow. Group 1: untreated mineralized dentin powder; Group 2: dentin powder demineralized in 1% aqueous H 3 PO 4 for 10 min; Group 3: mineralized dentin powder treated with 3 mL of Adper Scotchbond 1XT (SB1XT, 3M ESPE, St Paul, MN, USA) for 24 h at 4 °C in dark condition; Groups 4 and 5: mineralized dentin powder was treated either with 0.2 or 2% CHX water solution (Groups 4 and 5, respectively) for 30 min at 4 °C, rinsed with 1 mL of distilled water (five times), then incubated with 3 mL of SB1XT for 24 h at 4 °C in dark condition. All specimens were thoroughly rinsed with 4 mL of acetone and then centrifuged for 10 min (14,000 rpm) at 4 °C. Specimens were then re-suspended in 4 mL extraction buffer (50 mM Tris–HCl pH 6, containing 5 mM CaCl 2 , 100 mM NaCl, 0.1% Triton X-100), 0.1% non-ionic detergent P-40 (0.1 mM ZnCl 2 , 0.02% NaN 3 ) and EDTA-free protease inhibitor cocktail (Roche Diagnostics GmbH, Germany) for 24 h. Specimens were then centrifuged, supernatants were collected and protein content was precipitated with 25 wt% trichloroacetic acid (TCA) at 4 °C. TCA precipitates were re-solubilized in loading buffer (25% Trizma–HCl 1 M pH 6.8, 8% (w/v) sodium dodecyl sulfate, 40% glycerol and distilled water).
Total protein concentration of the extracts of mineralized and partially demineralized dentin powder was determined using the Bradford assay. Proteins were electrophorized under non-reducing conditions on 7.5% SDS-polyacrylamide gels copolymerized with 2 g/L gelatin (porcine skin). Activation of gelatinase proforms was achieved with 2 mM p-aminophenylmercuric acetate (APMA) for 1 h at 37 °C and then incubated for 24 h at 37 °C in zymography buffer (CaCl 2 , NaCl and Tris–HCl, pH 8.0). Gels were stained in 0.2% Coomassie Brilliant Blue R-250 and destained in destaining buffer (50% methanol, 10% acetic acid, 40% water).
Control zymograms were incubated in the presence of 5 mM EDTA and 2 mM 1,10-phenanthroline to inhibit gelatinases.
2.2
Specimens preparation for microtensile bond strength test
An additional 48 non-carious extracted human molars were selected and flat surfaces of middle/deep dentin were exposed with a slow speed diamond saw (Micromet, Bologna, Italy). Smear layer-covered dentin surfaces were etched with 35% phosphoric acid for 15 s (etching gel, 3M ESPE), rinsed and surfaces were blot dried according to the wet bonding technique. Specimens were randomly assigned to the following treatments ( N = 16 teeth in each group). Groups 1 and 2: acid-etched dentin surfaces were treated with aqueous solutions of either 0.2 or 2% CHX, respectively, for 30 s, blot dried and bonded with SB1XT; Group 3: received no pre-treatment before SB1XT application (control). SB1XT was applied in accordance with manufacturers’ instructions and light-cured. Resin composite build-ups were created with Filtek Z250 (3M ESPE, St Paul, MN, USA).
2.3
Microtensile bond strength evaluation
Resin–dentin sticks with cross-sectional area of approximately 0.9 mm 2 were obtained in accordance with the non-trimming technique . Each stick was measured and recorded for bond strength calculation. Sticks were divided in two equal groups and either stored for 24 h ( T 0 ) or for 2 years ( T 2 years ) in artificial saliva (prepared in accordance with the protocol of Pashley et al. , but without protease inhibitors) at 37 °C. Sticks were stressed until failure with a simplified universal testing machine at a crosshead speed of 1 mm/min (Bisco Inc., Schaumburg, IL, USA). Failure modes were evaluated as described by Breschi et al. .
2.4
Statistical analysis
As values were normally distributed (Kolmogorov–Smirnof test), data were analyzed with a two-way ANOVA (tested variables were: CHX concentration, time of storage) and Tukey’s post hoc test. To analyze the effect of chlorhexidine on fracture modes, mixed and dentin cohesive failures were combined. Wilcoxon Signed Ranks Test was used to analyze the differences in failure modes between T 0 and T 2 years for each group, and Kruskal–Wallis test was used to compare the fracture modes between the groups within each time points. Statistical significance was set at p < 0.05.
2.5
Nanoleakage evaluation
Twelve additional teeth ( N = 4/group) were prepared and bonded as previously described. Specimens were then vertically cut into 1-mm thick slabs to expose the bonded surfaces and submitted to the two storage times in artificial saliva at 37 °C: T 0 and T 2 years . Specimens were then submerged in 50 wt% ammoniacal silver nitrate for 24 h, rinsed, photodeveloped and processed for nanoleakage analysis under TEM and examined under TEM (Philips CM-10) operating at 70 kV .
2
Material and methods
2.1
Zymographic analysis
Reagents were purchased from Sigma Chemical (St Louis, MO, USA) unless otherwise specified.
Ten freshly extracted human molars were selected after patient’s informed consent was obtained under a protocol approved by the University of Trieste. Enamel, roots and remnant pulp tissue were removed and dentin powder was obtained by pulverizing liquid nitrogen-frozen coronal dentin with a steel mortar/pestle (Reimiller, Reggio Emilia, Italy). Five aliquots of 1 g each of dentin powder were obtained and treated as follow. Group 1: untreated mineralized dentin powder; Group 2: dentin powder demineralized in 1% aqueous H 3 PO 4 for 10 min; Group 3: mineralized dentin powder treated with 3 mL of Adper Scotchbond 1XT (SB1XT, 3M ESPE, St Paul, MN, USA) for 24 h at 4 °C in dark condition; Groups 4 and 5: mineralized dentin powder was treated either with 0.2 or 2% CHX water solution (Groups 4 and 5, respectively) for 30 min at 4 °C, rinsed with 1 mL of distilled water (five times), then incubated with 3 mL of SB1XT for 24 h at 4 °C in dark condition. All specimens were thoroughly rinsed with 4 mL of acetone and then centrifuged for 10 min (14,000 rpm) at 4 °C. Specimens were then re-suspended in 4 mL extraction buffer (50 mM Tris–HCl pH 6, containing 5 mM CaCl 2 , 100 mM NaCl, 0.1% Triton X-100), 0.1% non-ionic detergent P-40 (0.1 mM ZnCl 2 , 0.02% NaN 3 ) and EDTA-free protease inhibitor cocktail (Roche Diagnostics GmbH, Germany) for 24 h. Specimens were then centrifuged, supernatants were collected and protein content was precipitated with 25 wt% trichloroacetic acid (TCA) at 4 °C. TCA precipitates were re-solubilized in loading buffer (25% Trizma–HCl 1 M pH 6.8, 8% (w/v) sodium dodecyl sulfate, 40% glycerol and distilled water).
Total protein concentration of the extracts of mineralized and partially demineralized dentin powder was determined using the Bradford assay. Proteins were electrophorized under non-reducing conditions on 7.5% SDS-polyacrylamide gels copolymerized with 2 g/L gelatin (porcine skin). Activation of gelatinase proforms was achieved with 2 mM p-aminophenylmercuric acetate (APMA) for 1 h at 37 °C and then incubated for 24 h at 37 °C in zymography buffer (CaCl 2 , NaCl and Tris–HCl, pH 8.0). Gels were stained in 0.2% Coomassie Brilliant Blue R-250 and destained in destaining buffer (50% methanol, 10% acetic acid, 40% water).
Control zymograms were incubated in the presence of 5 mM EDTA and 2 mM 1,10-phenanthroline to inhibit gelatinases.
2.2
Specimens preparation for microtensile bond strength test
An additional 48 non-carious extracted human molars were selected and flat surfaces of middle/deep dentin were exposed with a slow speed diamond saw (Micromet, Bologna, Italy). Smear layer-covered dentin surfaces were etched with 35% phosphoric acid for 15 s (etching gel, 3M ESPE), rinsed and surfaces were blot dried according to the wet bonding technique. Specimens were randomly assigned to the following treatments ( N = 16 teeth in each group). Groups 1 and 2: acid-etched dentin surfaces were treated with aqueous solutions of either 0.2 or 2% CHX, respectively, for 30 s, blot dried and bonded with SB1XT; Group 3: received no pre-treatment before SB1XT application (control). SB1XT was applied in accordance with manufacturers’ instructions and light-cured. Resin composite build-ups were created with Filtek Z250 (3M ESPE, St Paul, MN, USA).
2.3
Microtensile bond strength evaluation
Resin–dentin sticks with cross-sectional area of approximately 0.9 mm 2 were obtained in accordance with the non-trimming technique . Each stick was measured and recorded for bond strength calculation. Sticks were divided in two equal groups and either stored for 24 h ( T 0 ) or for 2 years ( T 2 years ) in artificial saliva (prepared in accordance with the protocol of Pashley et al. , but without protease inhibitors) at 37 °C. Sticks were stressed until failure with a simplified universal testing machine at a crosshead speed of 1 mm/min (Bisco Inc., Schaumburg, IL, USA). Failure modes were evaluated as described by Breschi et al. .
2.4
Statistical analysis
As values were normally distributed (Kolmogorov–Smirnof test), data were analyzed with a two-way ANOVA (tested variables were: CHX concentration, time of storage) and Tukey’s post hoc test. To analyze the effect of chlorhexidine on fracture modes, mixed and dentin cohesive failures were combined. Wilcoxon Signed Ranks Test was used to analyze the differences in failure modes between T 0 and T 2 years for each group, and Kruskal–Wallis test was used to compare the fracture modes between the groups within each time points. Statistical significance was set at p < 0.05.
2.5
Nanoleakage evaluation
Twelve additional teeth ( N = 4/group) were prepared and bonded as previously described. Specimens were then vertically cut into 1-mm thick slabs to expose the bonded surfaces and submitted to the two storage times in artificial saliva at 37 °C: T 0 and T 2 years . Specimens were then submerged in 50 wt% ammoniacal silver nitrate for 24 h, rinsed, photodeveloped and processed for nanoleakage analysis under TEM and examined under TEM (Philips CM-10) operating at 70 kV .
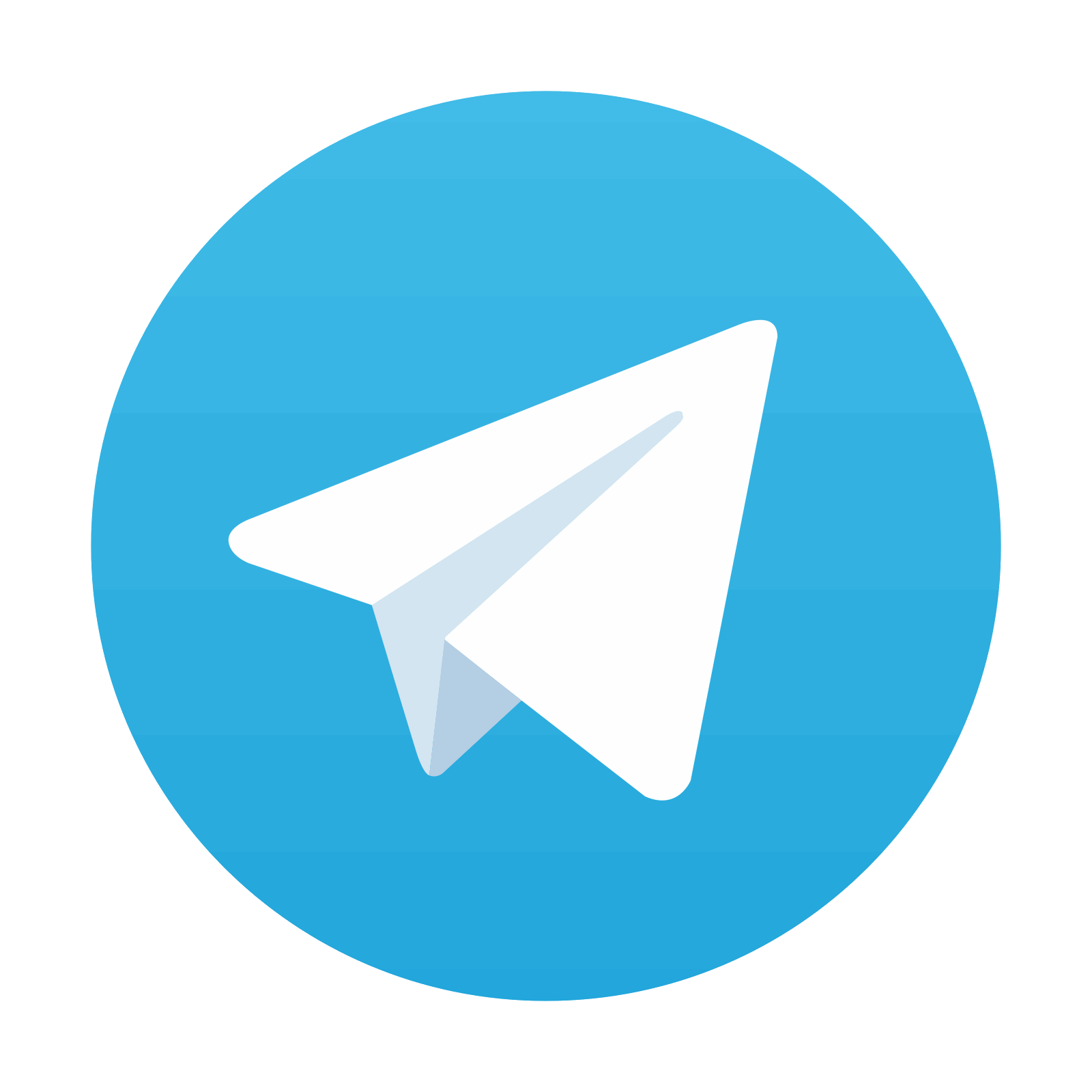
Stay updated, free dental videos. Join our Telegram channel

VIDEdental - Online dental courses
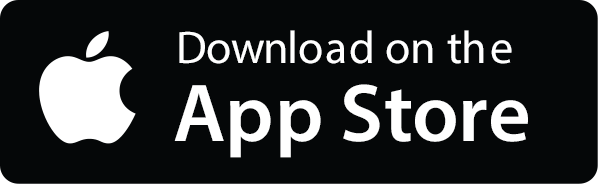
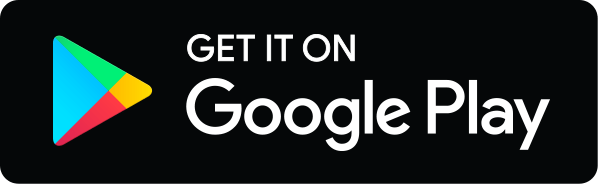