Abstract
Objective
The edge chipping test was used to measure the fracture resistance of CAD/CAM dental restoration ceramics and resin composites.
Methods
An edge chipping machine was used to evaluate six materials including one feldspathic porcelain, two glass ceramics, a filled resin-composite, a yttria-stabilized zirconia, and a new ceramic-resin composite material. Force versus edge distance data were collected over a broad range of forces and distances. Data were analyzed by several approaches and several chipping resistance parameters were evaluated. The effects of using different indenter types were explored.
Results
The force versus distance trends were usually nonlinear with good fits to a power law equation with exponents usually ranging from 1.2 to 1.9. The order of chipping resistance (from least to greatest) was: feldspathic porcelain and a leucite glass ceramic (which were similar), followed by the lithium disilicate glass ceramic and the two resin composites (which were similar), and finally the zirconia which had the greatest resistance to chipping. Chipping with a Vickers indenter required 28–45% more force than with the sharp conical 120° indenter. The two indenters rank materials approximately the same way. The power law exponents were very similar for the two indenters for a particular material, but the exponents varied with material. The Rockwell C indenter gives different power law trends and rankings.
Significance
Despite the variations in the trends and indenters, simple comparisons between materials can be made by chipping with sharp conical 120° or Vickers indenters at 0.50 mm. Broad distance ranges are recommended for trend evaluation.
1
Introduction
The edge chipping test is used to evaluate the resistance of brittle materials to flaking near an edge as shown in Fig. 1 . Chips are formed by advancing an indenter into a material near an edge. The force required for chip formation, F , increases with distance from the edge, d . The shape of the chip is usually independent of the material tested.

This method was originally developed in the late 1980s to study hard metal cutting tools at the National Physical Laboratory in London . Later, J. Quinn pioneered the application of this method to dental restorative materials . Other groups are now using it and it has even been used to evaluate human dentin and enamel . A short review paper on edge chipping as applied to dental materials was recently prepared . This method has also been used for many applications other than dental materials . A draft European prestandard was written in 2009 .
Chipping is a common failure mode in many dental restorations made of brittle materials. Although the laboratory scale edge chip tests use specific indenters on test blocks with carefully prepared edges, the chips physically resemble some types of in-vivo failures and shown in Supplemental Fig. 1 in the on-line version of this paper. In addition, edge chipping arising from CAD/CAM machining of dental restoration materials is a major concern . In that study it was shown that the microstructure of the material played a very strong role in determining chip sizes in 90° edges prepared by CAD/CAM milling with a 4 cm grinding disk having 64 μm average grain size diamonds .
Unfortunately, divergences in technique, analysis, and reporting of results from laboratory edge chip tests have emerged and the divergences hamper data comparisons. Indenters include the original Rockwell C diamond indenters, Knoop and Vickers pyramidal indenters, and the sharp-tip diamond 120° conical indenter that we favor. The latter is nearly identical to a 120° conical Rockwell C indenter, but the Rockwell C indenter has a rounded tip with a 200 μm radius. In this paper, we will explore some of the differences in testing procedures and the way the results are reported. We will also determine if the edge chipping resistance ranking of materials varies with indenter type.
One criticism of the common lab scale edge chip tests is that they use sharp diamond indenters in very controlled conditions with specimens having nicely defined 90° edges. People usually do not chew on sharp diamond indenters and teeth do not have flat surfaces with 90° corners. Our objective with the edge chip tests is to conduct chipping resistance experiments under controlled conditions with well-defined indenters and reference configurations. One could apply the same criticism to classic fracture toughness ( K Ic ) tests using compact tension specimens or single-edged precracked bend bars. These configurations are not representative of actual components, but the classical tests do measure a fundamental fracture resistance property under controlled conditions. That is our objective with the edge chip test: measure chipping resistance under controlled conditions.
Edge chipping is a multistep process that entails:
- a.
formation of a small indentation
- b.
formation of short, stable radial cracks (unless the indenter is blunt, in which case cone cracks form in some materials).
- c.
propagation of some of the radial cracks downward and parallel to the side surface.
- d.
unstable crack propagation towards the side surface causing a flake to pop off.
Early work emphasized the relationship between edge toughness and traditional fracture parameters such as K Ic , the fracture toughness; G Ic , the critical strain energy release rate; or γ f , the fracture surface energy. Most investigators graph the edge chip data as force versus distance and use linear repression to fit the data. The slope is interpreted as the “edge toughness, M , or T e ”. It has units of force/distance which is dimensionally analogous to (force × distance)/area or energy/area. Thus, it should not be surprising that the edge toughness sometimes correlates better with G Ic or γ f than with K Ic .
The objectives of this paper are to show typical edge chip results for six commercial CAD/CAM restoration materials, compare results from different indenters, and report outcomes using alternative resistance indices. It is hoped that this work will contribute to refinements to the CEN draft standard for technical ceramics so that it will be useful to the dental community. The new data in this paper may also be useful for modeling of the edge chip process. Results are analyzed with the two force versus distance functions (linear and power law) that have been customarily applied to edge chipping data. Part 2 includes a more comprehensive analysis of the results including correlations with traditional fracture toughness parameters and presentation of a new force–distance function based on a phenomenological model that overcomes some of the limitations of the power law fit used in this paper.
Two hypotheses are set forth in this paper: indenter type does not affect test results, and force versus distance data follow a linear trend.
2
Materials
The six commercial materials that were tested are listed in Table 1 . 1
1 Commercial products and equipment are identified only to specify adequately the experimental procedures and does not imply endorsement by the authors, institutions or organizations supporting this work, nor does it imply that they are necessarily the best for the purpose.
Test specimens for the various materials varied in size and shape from 3 mm by 4 mm cross section sized bend bar fragments to larger square blocks of 10–20 mm length per side. The two feldspathic porcelains, one glass ceramic, and a new ceramic-filled composite were available in the form of four to five wear test type rectangular blocks cut from CAD/CAM blanks. Spare bend bars or broken bend bar fragments, typically 3 mm × 4 mm × 15 mm to 20 mm were used for a yttria-stabilized zirconia and the filled resin composite. Care was taken to grind and the surfaces to make well-defined, reproducible edges. Specimen preparation procedures varied with the materials, but top and side surfaces were progressively hand ground with a rotary polishing wheel using 1200, 2400, and 4000 grit wet SiC papers. For the bend bar specimens, coarse-grit initial grinding with 600, 800, and 1000 grit papers was used to remove the ∼0.15 mm sized chamfers that were on the long edges.
Material | H b (GPa) | E c (GPa) | ρ (g/cm 3 | K Ic d (MN/m 1.5 ) | G Ic e (J/m 2 ) | B f (μm −1 ) | Ref. |
---|---|---|---|---|---|---|---|
Zirconia, 3Y-TZP (3M-ESPE Lava) | HV, 12.3 | 210 | 6.1 | (∼10) | – | ||
HK2, 11.2 (0.2) | 216 res | – | 4.8 (0.6) fract | 109.7 | 122.0 | Unpubl. | |
Lithium disilicate glass ceramic (Ivoclar, IPS e.max CAD) | HV, 5.7 (0.2) | 95 (5) | 2.5 (0.1) | 2.0–2.5 SEVNB | 42–66 | 87–135 | |
Nanoceramic filled composite (3M-ESPE Lava Ultimate) | HV1, 1.05 (0.01) | 14.8 | 1.94 | 2.02 (0.15) SEVNB | 276 | 3.8 (0.1) | |
Filled resin composite (3M-ESPE Paradigm, MZ100) | HK, 0.99 (0.02) | 12.2 defl | 2.13 | 1.1 (0.2) fract. | 99 | 9.98 | |
HV1, 1.31 (0.02) | 12 defl | – | 1.3 (0.1) SBCN | ||||
– | – | 1.21 (0.07) CT g | |||||
– | 1.64 (0.15) SEVNB | ||||||
Feldspathic porcelain (Vita Mark 2) | HV, 6.3 (0.3) | 69.7 res | 2.4 | 1.19 (0.05) SEPB | 20.3 (1.7) | 311 (41) | |
HV1, 6.1 (0.4) | – | – | 1.45 (0.09) SEVNB | ||||
Leucite glass ceramic (Ivoclar IPS Empress CAD) | HV1, 5.6 (0.2) | 66.8 res | 2.4 | 1.03 (0.03) SEVNB | 15.9 (0.9) | 352 (33) | |
HV, 5.8 | – | SEVNB | |||||
HV, 6.2 | 62 | – | 1.3 (0.1) SENB | ||||
HV1, 5.3 (0.4) | – | – | 1.44 (0.1) SEVNB |
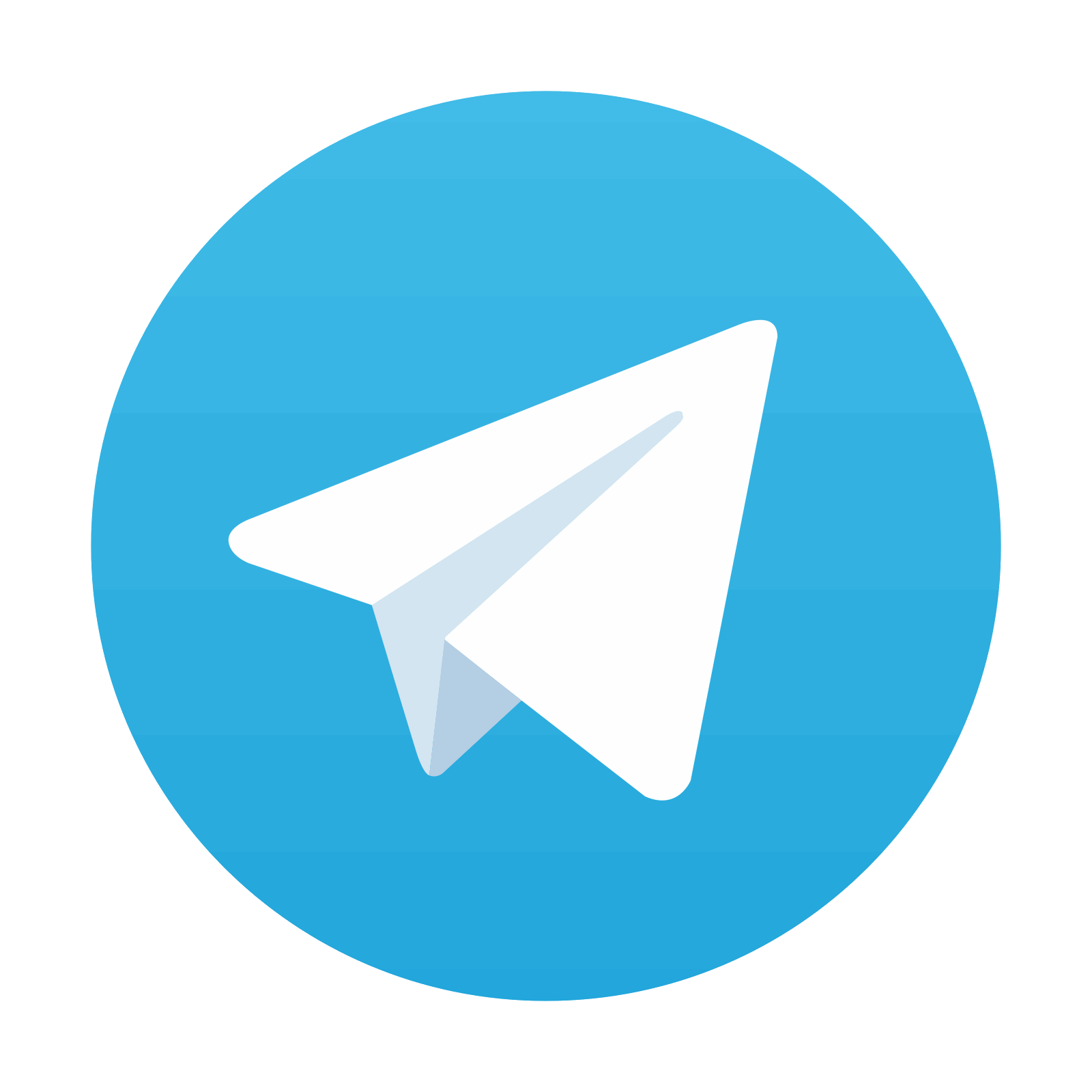
Stay updated, free dental videos. Join our Telegram channel

VIDEdental - Online dental courses
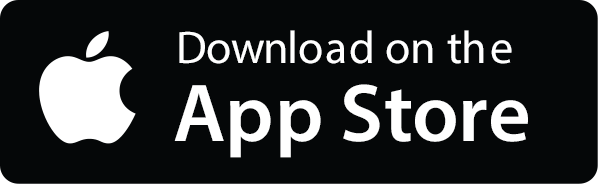
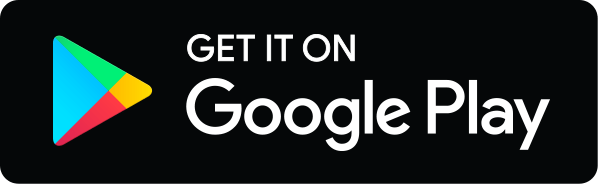
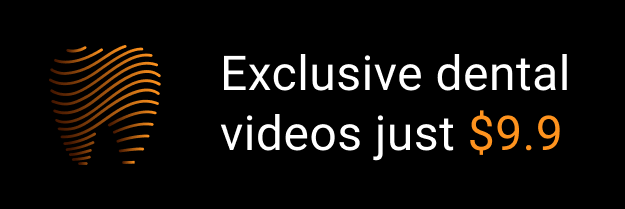