Abstract
Objectives
Tetragonal zirconia polycrystal (TZP) materials are widely used for full ceramic partial dentures, even though their mechanical properties might change during service. A key property for the durability of the constructs is thought to be an inhibition of crack propagation by phase transformation toughening. Because dental prosthesis are ground and polished for adjustment purposes, it is important to understand the effects of mechanical surface treatments, on localized transformation and around the propagating cracks.
Methods
Sintered samples of commercially available 3 mol-% Yittria-doped TZP were ground and polished and the surface structure and phase composition were compared with those of re-transformed annealed samples. Microindentation was used to induce cracks and nanoindentation was performed to determine the local variety of hardness and indentation modulus, coupled with XRD and SEM investigations.
Results
Y-TZP polished surfaces exhibited 9% monoclinic phase content and have reduced hardness and indentation modulus amounting 16.3 GPa and 210.6 GPa, respectively. Y-TZP re-transformed annealed sample revealed 19.4 GPa and 242.3 GPa, respectively. A localized reduction of the stiffness around the crack tips on the annealed surface reveals an irregular arrangement of t-m-transformed grains. Electron micrographs show more damage on the transformed surface following microindentation than on indented annealed surfaces.
Significance
Y-TZP prostheses are adapted and roughened by clinicians prior to bonding to teeth. Annealing recovers properties and microstructure that is changed by the adaptation of the outer layer. This might be important to ensure long-term toughening functionality of the dentures and optimal comfort for the patients.
1
Introduction
Tetragonal zirconia polycrystals (TZP) ceramics are used in prosthetic dentistry because they combine an esthetically pleasing appearance with biocompatibility and excellent mechanical properties In addition to a high stiffness, TZP ceramics are characterized by a distinct high fracture toughness. TZP ceramics are stabilized, often with yttria, such that toughening arises from stress-induced transformations of the meta-stable tetragonal phase into the thermodynamically stable monoclinic phase. This transformation (t-m-transformation) leads to a volume increase that results in the appearance of a compressive stress field that arrests or slows down crack propagation. The toughening mechanisms acting in TZP have been described in detail elsewhere, e.g. . The finished and sintered dentures require adaptation so as to match patient comfort needs. However, mechanical manipulations will induce surface stresses that may trigger the t-m-transformation thus decreasing the material toughness and durability . Indeed it has been shown that even though bending strength may increase for certain surface treatments due to compressive residual stresses connected with the phase transformation, toughness always decreased .
Indentation methods have been advantageously applied to elucidate mechanical characteristics of zirconia. Microindentation has been used to measure hardness and Young’s modulus of a variety of stabilized zirconia formulations . For higher resolution measurements, nanoindentation is of great use, because it is well suited to measure near-surface properties of flat samples . The grain polymorphs (monoclinic, cubic or tetragonal) as well as size and crystallographic orientation all have significant effects on the Young’s modulus and the material hardness . Different studies have further shown that the measured mechanical properties are strongly influenced by the load that is applied during indentation .
The extent of the transformed zones in TZP has been investigated by various methods including transmission electron microscopy (TEM) , X-ray diffraction (XRD) , optical interference microscopy , Raman spectroscopy and scanning powder-diffraction based contrast tomography (μCT-XRD) . The investigations showed that the thickness of the transformed layer varies, depending on the surface treatment, with different grains transforming to different extents: partially, fully or not at all . In the vicinity of Vickers indentations, the extent of the transformed phase decreases almost linearly with distance from the center of the indent . In addition to the reduction in toughness due to the phase transformation, other mechanical properties are also affected: microhardness decreases with an increase in the monoclinic phase fraction .
The effects of standard dental adaptation (grinding and polishing) techniques on 3 mol% yttria-stablised TZP constructs (Y-TZP) are to-date not fully understood. So far, the influence of the t-m-transformation on the mechanical properties on the one hand and the extent of transformation following mechanical treatments on the other hand have been investigated separately. In contrast, local variations of hardness and elastic modulus due to a non-homogeneous transformation have not been looked at. We therefore examined sintered untreated as well as mechanically manipulated samples to better understand the effects and extent of transformation. We used microindentation to induce small and locally limited t-m-transformation zones on surfaces of mechanically treated dental Y-TZP samples and we performed virtually non-destructive nanoindentation to compare the different samples in respect to the surface treatments, and to determine the spatial variation of mechanical properties.
2
Materials and methods
2.1
Sample preparation and treatment
A commercially available 3 mol-% Y-TZP (DC-Shrink, Bien Air, Bienne, Switzerland) was used. Seven samples were machined from bulk “green” (pre-sintered) blocks using a water-cooled diamond saw (Isomet, Buehler GmbH, Duesseldorf, Germany) and were subsequently ground flat on 1200 grit SiC paper with a micro-grinding system (400CS, Exakt, Norderstedt, Germany) to form 10 mm diameter discs that were 2.5 mm thick. Each sample was sintered at 1530 °C for 2 h in a VITA Zyrcomat oven (Vita Zahnfabrik, Bad Saeckingen, Germany) according to recommended procedures .
Six of the sintered samples were subsequently trimmed on a polishing machine (Minimet 1000, Buehler Ltd., Lake Bluff, IL, USA) using a series of grinding diamond disks of decreasing grit-size down to 6 μm, followed by polishing with 3 and 1 μm diamond polishing pastes. The load delivered by the grinding-arm of the polishing machine was set to 10 N with a rotation speed of 15 rpm. We consider this to be an extremely gentle grinding process and assume that this treatment to be on the lower end of what is induced during chairside adjustment of the TZP dentures. A smoothing of the surface roughness of the sintered surface was necessary to be able to perform the nanoindentation measurements which cannot take place on the rough sintered (non-polished) samples. Three of the polished samples were annealed at 1530 °C for 2 h so as to re-transform the monoclinic phase fraction that we assume was induced by polishing. Consequently, we ended up with three types of samples: Sintered with no further manipulations, as a reference, (“sintered”), polished following sintering (“polished”) and annealed after polishing (“annealed”).
2.2
Sample characterization
The phase compositions of the sintered, polished and annealed samples were measured by X-ray diffraction (XRD; Nonius PDS120, Bruker AXS, Karlsruhe, Germany with an INEL CPS-120 curved position-sensitive detector INEL Ltd., Swindon, UK). The XRD measurements were performed in reflection mode using a Cu Kα source ( λ = 0.15406 nm) with recording times of 12–24 h, using an angle of incidence of 5°. The peaks were matched with PDF files Nos. 42-1164, 49-1642 and 37-1484 for the tetragonal, cubic and monoclinic phases, respectively. The diffracted signals of the phases in our setup originate from a penetration depth of 1–3 μm . All intensities were normalized with respect to the tetragonal (1 1 1) peak. The volume fraction of monoclinic phase V m was determined in accordance to Eq. (1) as previously described :
V m = 1.311 ( I m ( 1 ¯ 1 1 ) + I m ( 1 1 1 ) ) I t ( 1 0 1 ) + 1.311 ( I m ( 1 ¯ 1 1 ) + I m ( 1 1 1 ) )
where I t and I m represent the integrated intensity of the tetragonal (1 0 1) and monoclinic (−1 1 1) and (1 1 1) peaks, respectively. The tetragonal (1 0 1) peak corresponds to the indexing reported by Toraya et al. and Teufer assuming a body-centered tetragonal crystal structure, whereas some literature data are based on an equivalent face centered tetragonal system .
The sample surfaces were also observed by scanning electron microscopy (SEM, FEI Quanta 600 FEG, FEI, Eindhoven, Netherlands) using the low vacuum mode so as to avoid surface coating. The averages grain size of these samples were calculated using the circle method [DIN EN ISO 643]. Additionally grain boundaries were identified, and marked to determine the area of each single grain (Image P2, HK Messsysteme GmbH, Berlin, Germany) and to calculate the grain size distribution. Note however, that grains were not visible on the polished surfaces due to grinding scatches and hence our analysis is limited to the sintered and annealed samples. This analysis was done to ensure that the surface grain structure that develops after annealing was similar to that of conventionally sintered samples.
2.3
Indentation experiments
A microhardness conventional tester equipped with a Vickers diamond indenter (MHT-10, Anton Paar, Graz, Austria) was used to indent and initiate cracks on the Y-TZP surfaces of polished and annealed samples. Each sample was thus indented with a load of 4 N with a dwell time of 15 s. We used 4 N loadings because this load is large enough to induce phase transformation and to produce cracks around the microindent.
A scanning nanoindenter (Ubi 1, Hysitron Inc., Minneapolis, USA) was used to measure representative polished and annealed samples. A tetrahedral Berkovich diamond indenter tip was used. The zone where microindentation was performed was identified using the in-built optical microscope of the nanoindenter. Two types of indentation experiments were of interest: nanoindentation measurements performed on intact regions of the outer surface of the polished and annealed specimens, respectively, as well as nanoindentations on both the polished and annealed samples in areas where cracks – emanating from the corners of the microindented area – were seen. The specimens were loaded at a rate of 1000 μN/s up to a maximum load of 10 mN and held under load for a dwell time of 15 s followed by a release at the same rate. The instrument was calibrated by performing indents of increasing depth in fused quartz with a known indentation modulus of 69.6 GPa . Typical load-displacement curves for the nanoindentation experiments on the polished and annealed surfaces are shown in Fig. 1 a. The data were analysed using TriboScan software (Hysitron Inc., Minneapolis, USA, version 8.2.0.14) following the methods described by Oliver and Pharr . Parameters of interest included peak load P max and peak displacement h max . The load-displacement-curves were fitted with a second order polynomial in the region between 20 and 95% of the maximum load and used to determine the slope of the unloading curve at the maximum load. This information was used to calculate the unloading contact stiffness S and contact area A c . The hardness H and indentation modulus E r (also called: reduced Young’s modulus) were then calculated as described by Eqs. (2) and (3) .
H = P max A C
E r = π 2 A C S
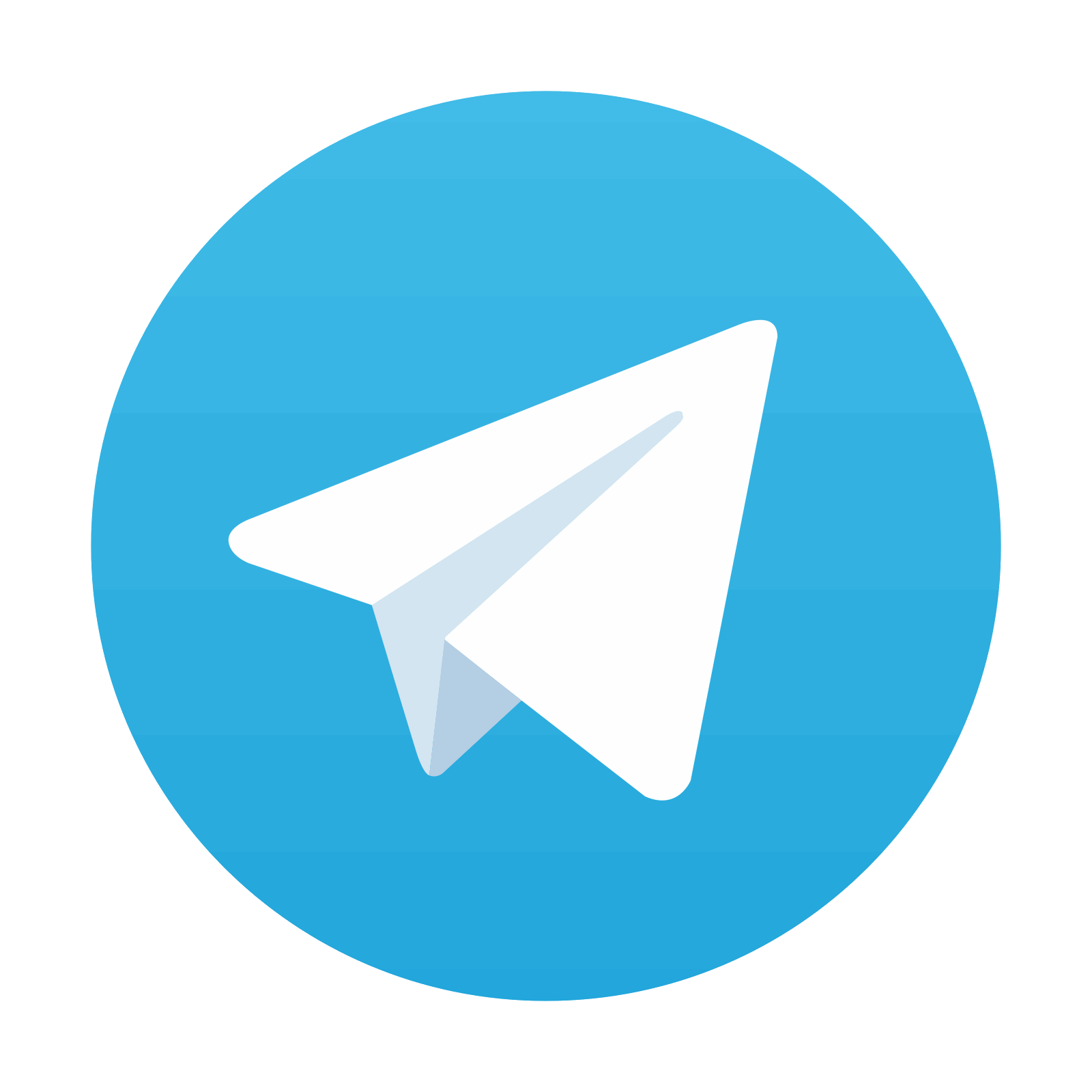
Stay updated, free dental videos. Join our Telegram channel

VIDEdental - Online dental courses
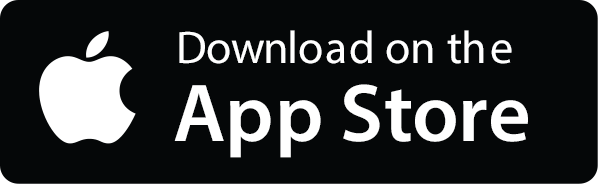
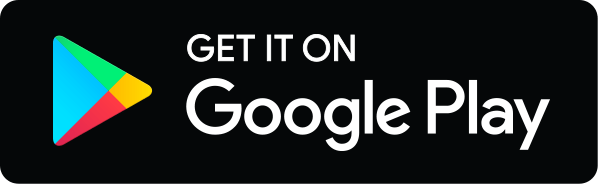