Abstract
Objectives
Aim of the research is to compare the orthodontic appliances fabricated by using rapid prototyping (RP) systems, in particular 3D printers, with those manufactured by using computer numerical control (CNC) milling machines.
3D printing is today a well-accepted technology to fabricate orthodontic aligners by using the thermoforming process, instead the potential of CNC systems in dentistry have not yet been sufficiently explored.
Materials and methods
One patient, with mal-positioned maxillary central and lateral incisors, was initially selected. In the computer aided virtual planning was defined that, for the treatment, the patient needed to wear a series of 7 removable orthodontic appliances (ROA) over a duration of 21 weeks, with one appliance for every 3 weeks. A non-contact reverse engineering (RE) structured-light 3D scanner was used to create the 3D STL model of the impression of the patient’s mouth.
Numerical FEM simulations were performed varying the position of applied forces (discrete and continuous forces) on the same model, simulating, in this way, 3 models with slice thickness of 0.2 mm, 0.1 mm (RP staircase effect) and without slicing (ideal case). To define the areas of application of forces, two configuration “ i ” and “ i −1” of the treatment were overlapped.
6 patients to which for three steps (3rd, 4th and 5th step) were made to wear aligners fabricated starting from physical models by 3D printing (3DP-ROA) and afterwards, for the next steps (6th, 7th and 8th step), aligners fabricated starting from physical models by CNC milling machine (CNC-ROA), were selected.
Results
For the 6 patients wearing the CNC-ROA, it was observed a best fitting of the aligner to the teeth and a more rapid teeth movement than the 3DP-ROA (2 weeks compared to 3 weeks for every appliance).
FEM simulations showed a more uniform stress distribution for CNC-ROA than 3DP-ROA.
Conclusions
In this research, 6 different case studies and CAD-FEM simulations showed that, to fabricate an efficient clear and removable orthodontic aligner, it is necessary to consider a compromise of several factors. A lower staircase effect (lower layer thickness) and a higher physical prototype accuracy allow a better control of tooth movement.
1
Introduction
Dental aligners allow to generate and transmit a system of forces and moments to the teeth and their surrounding periodontal ligaments. They can be divided into two main groups: fixed and removable devices.
Traditional treatment with fixed orthodontic appliances creates some plaque retention sites and thus increases a patient’s risk to develop white spot lesions, caries and periodontitis. For these reasons patients with fixed appliances must follow a rigid oral hygiene protocol to avoid these side effects. How to approach these issues were studied in .
In 1999, Align Technology, Inc. (San Jose, CA, USA) introduced a new generation of appliances in orthodontics: a removable alternative to fixed appliance therapy in correcting malocclusion, marketed under the trade name Invisalign .
However, although Align Technology, Inc. has been manufacturing removable orthodontic appliances for over 10 years, the concept of removable appliances is far from new, it was developed in 1944 by Harold D. Kesling . One of the limitations that prevented these positioning appliances from becoming a new standard in orthodontic care, was the lack of appropriate instruments and methodologies.
In the last years there has been a growing interest in orthodontic treatments with removable appliances, thanks to the latest technological advancements in scanning, CAD/FEM software and rapid prototyping (RP) techniques .
Nowadays several companies offer this type of technology to dentists and orthodontists worldwide.
The straightening teeth process is based on sequential appliances (aligners) made of a clear thermoplastic material. The aligners are worn on a biweekly regimen, and a progressive alignment of 0.2 mm translation or 1° rotation per tooth is designed in each aligner .
The primary benefit of the clear and removable customized aligners in moving the dentition is the ability to remove them when eating, brushing and flossing, and the superior comfort and ease of use. Furthermore they are “invisible” and for many patients, aesthetic considerations during treatment are as important as other factors, such as comfort, pain, length of treatment or cost .
The fabrication process ( Fig. 1 ) of the teeth clear removable aligners begins with digital scans (reverse engineering (RE) acquisition – step 1) of the patient teeth, so that a 3D model can be created. This step can be carried out, starting from a plaster model of the teeth created, from an impression of the patient’s mouth, using a non-contact RE system or directly on the patient’s mouth using an intraoral scanner.

Specialist orthodontic technicians then plan out each individual movement of the teeth according to the treatment plan as prescribed by the orthodontist (computer aided virtual straightening – step 2).
A 3D physical model is then manufactured by a RP system (physical model fabrication – step 3), for each single step of the sequence defined in the virtual planning.
Finally from this physical model, the clear orthodontic aligner (0.4 mm thick) is manufactured by a vacuum thermoforming process in which a polyurethane resin sheet is stretched over each of the physical model (aligner fabrication – step 4).
Step 3 of the process is carried out by using RP systems. Align Technology, Inc. uses SLA (Stereolithographic Apparatus) machines from 3D Systems: 35 SLA systems manufacture about 40,000 parts a day for a yearly total of 8–10 million pieces .
ClearCorrect LLC, another US company producing clear, removable orthodontic aligners based on digital technology, uses 3D printing systems by Objet Ltd. as an integral part of its mass-scale manufacturing of custom-made orthodontic aligners .
So RP models play a crucial role in automating the aligner production process, but in any rapid prototyping process, the layer by layer building process introduces an error on the amount of material used compared to the volume specified by the computer aided design model. This error causes the staircase effect on the surface profile ( Fig. 2 ) and adversely affects the dimensional accuracy as well as surface finish for different part build orientations. Inclined and curved surfaces show staircase effects more predominantly than other surfaces. The orientation, at which the part is built, has a significant effect on the quality of various surfaces of the part .

Part’s orientation also affects other factors such as the build time, the complexity of support structure, shrinkage, curling, trapped volume, and material flow in many rapid prototyping processes.
Stair-stepping inaccuracies can be reduced either by decreasing the layer thickness and by orienting the part or using the adaptive slicing which adapts the thickness of each build layer to better match the part surface geometry ; nevertheless, today there is no way the staircase effect can be totally eliminated.
RP systems are also called with the term “additive manufacturing” (AM) for distinguish them from computer numerical control (CNC) systems, which instead subtract material to obtain the final shape.
In the paper the authors take into account a CNC milling 5-axis machine to manufacture orthodontic appliances. Although the RP process is more efficient than CNC traditional technique as the former does not require much human input (CNC machining instead involves CAM programming, and so, much time is spent in programming toolpaths), and models are generated quickly and independently of the shape complexity, CNC systems allow to get higher accuracy than RP systems and it does not present the staircase effect. CAD-FEM simulations and six different case studies are used to compare the appliances fabricated in the two ways.
2
Materials and methods
2.1
3D virtual DATA of the patient teeth
One patient with mal-positioned maxillary central and lateral incisors was selected.
3D data of the patient teeth were generated from the plaster model obtained from an impression of the patient’s mouth.
A non-contact RE structured-light 3D scanner (Sint Scan 130, by Sintesi Sud, Italy) was used to create the 3D STL model ( Fig. 3 ).
Within a computer assisted environment, CAD-like operations are then made to separate teeth, so the whole 3D model the patient’s teeth is ready for the analysis and the virtual planning of orthodontic treatment (performed with the software package OrthoAnalyzer™ by 3Shape). In our case study, the sequence of different steps to move the teeth were defined: a series of 7 removable orthodontic appliances (ROA) were planned, each for 3 weeks, for a 21-week treatment ( Fig. 4 ).
Figs. 5 and 6 show the 3D STL models obtained, respectively, considering the ideal case (without slicing) and a RP slicing of 0.2 mm.
CNC systems allow to get very smooth surfaces and higher accurate contours than RP systems; moreover no staircase effect is generated as, instead, observed in the RP models ( Fig. 6 ).
In Table 1 the main characteristics of various RP and CNC systems, suitable to fabricate orthodontic aligners, are shown and compared.
System/manufacturer | Technology | Build envelope | Layer thickness | Accuracy |
---|---|---|---|---|
Objet 24/Objet Ltd. (merged with Stratasys by 2012) | Jetted photopolymer | 9.22 mm × 192 mm × 149 mm | 0.028 mm | ±0.1 mm |
ProJet™ 6000/3D Systems | Film transfer of photopolymer | 250 mm × 250 mm × 250 mm | 0.10 mm | 0.025–0.05 mm |
Mojo™/Stratasys | Fused deposition modeling (FDM) | 127 mm × 127 mm × 127 mm | 0.17 mm | Not specified |
ZPrinter ® 150/Z Corporation [Acquired by 3D Systems] | Three dimensional printing (3DP) | 236 mm × 185 mm × 127 mm | 0.10 mm | Not specified |
Roland DWX-50/Roland DG Corporation | Computer numerical control (CNC) | Disc external diameter: 98–100 mm height: 12–26 mm | 0 | ±0.01 mm |
Data are derived from the datasheets from Manufacturers.
In the paper, among these systems, the 3D printing Objet 24 by Objet Ltd and the 5-axis CNC milling machine, Roland DWX-50 by Roland DG Corporation were used.
In Table 2 for the two systems chosen in the paper, times and costs in the models manufacturing are compared (data from the Sintesi Sud Company, Italy).
System | Number of models | Total time | Mean time | Mean cost |
---|---|---|---|---|
Objet 24 | 8 | 7 h 30 min | 55 min | 5–10 € |
Roland DWX-50 | 1 | 80 min | 80 min | 2–5 € |
2.2
FEM simulations
To numerically simulate the difference of applied forces action, with and without staircase effect, FE analysis was carried out.
To deeply understand what happen on the teeth during the application of the aligners made by two different manufacturing processes, numerical FEM simulations were performed within NASTRAN (by MSC Corporation). For the FE pre-processing and post-processing phases HyperMesh V10.0 and HyperView (by Altair Engineering, Inc.) software were respectively used.
To perform FE analysis, it was necessary to individuate the areas of application of forces. For two consecutive steps they can be highlighted by overlapping the i th configuration with the i −1th configuration: Fig. 7 shows the overlapped areas on which forces have to be applied.
All models were modeled with TETRA (3D tetrahedral with 4 nodes) elements. 213,378 nodes and 882,700 elements were used.
Due to the high number of elements of the models, it was chosen to achieve a gradual mesh ranging from a minimum feature size of 0.02 mm in the areas of greatest interest (areas of application of the forces) to a maximum size of 1.40 mm in the other areas.
FEM simulations were performed considering 3 different cases by using the same model, varying only the position of the applied loads (forces pairs acting on each tooth). In this way it was possible to simulate models with slice thickness of 0.2 mm and 0.1 mm (RP models) and without slicing (ideal case). The base of the model was fixed ( Fig. 8 ).
Fig. 9 shows the local areas where loads were defined, obtained, as explained above, by overlapping two consecutive configurations of the treatment.
A linear static structural analysis was performed considering an unique material with the following mechanical properties: E = 70e3 MPa, ν = 0.30, and a load equal to 25 N, applied for each side of each tooth, varying the position of the applied forces (0.2 mm, 0.1 mm and case continuum) and assuring the same total pressure effect ( Fig. 10 ).
2.3
Case studies
6 patients were selected. The steps 1–4, described in Section 1 of the paper, were carried out.
For the step 3, a 3D Printing, Objet 24 by Objet Ltd and a 5-axis CNC milling machine, Roland DWX-50 by Roland DG Corporation, were used.
Fig. 11 shows virtual models before and after treatment for one of the six patients. For the same patient, in Table 3 are summarized the teeth to be moved and the type of movement.
Teeth | Rotation | Angulation | Inclination | Left/right | Extrusion/intrusion | Forward/backward |
---|---|---|---|---|---|---|
15 | 3° | 2.7 mm | ||||
14 | 2.4 mm | |||||
13 | 13° | −17.4° | −3.9° | −3.8 mm | 2.2 mm | |
12 | 0° | −0.9 mm | 3.4 mm | |||
11 | 3° | −0.3 mm | ||||
21 | 3° | 0.4 mm | ||||
22 | −2° | 4° | 0.9 mm | 1.6 mm | ||
23 | −24° | 8.7° | −3.5° | 2.7 mm | −1.5 mm | |
24 | 0.6 mm | 1.7 mm | ||||
25 | 0.5 mm | 1.5 mm | ||||
34 | 12° | |||||
33 | 1° | 4° | 0.4 mm | |||
32 | 6° | 0.2 mm | ||||
31 | −2° | |||||
41 | −1° | |||||
42 | 2° | 0.1° | 3° | |||
43 | 4° | |||||
44 | 6° |
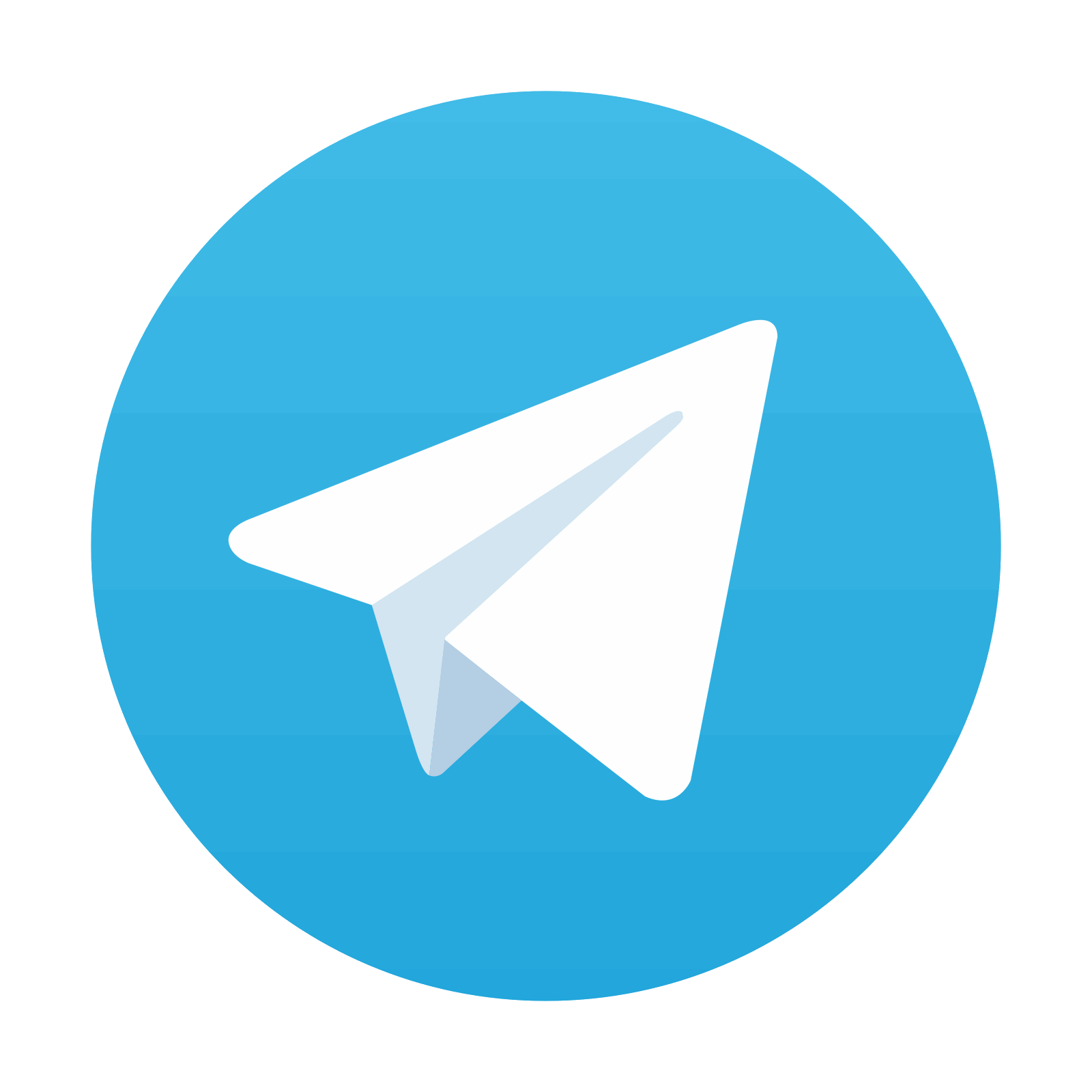
Stay updated, free dental videos. Join our Telegram channel

VIDEdental - Online dental courses
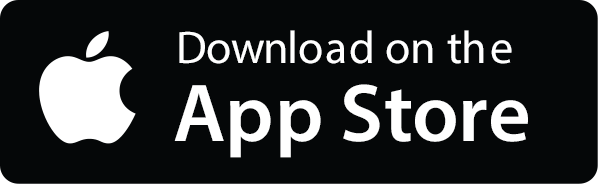
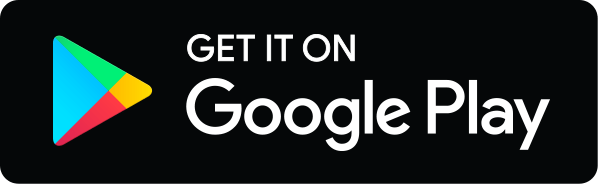
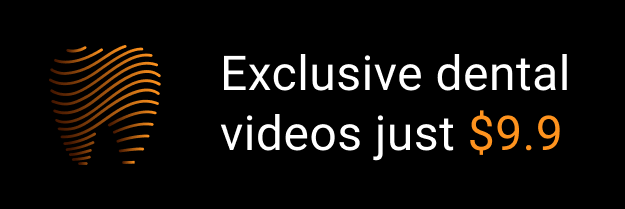