Abstract
The objective of this study was to analyze changes in expression pattern of Slit1 and Robo2, and to clarify the relationship between these changes and functional recovery of the axotomized inferior alveolar nerve (IAN) without repair using a rat IAN axotomy model. Slit1 and Robo2 were weakly expressed in samples taken from trigeminal ganglion (TG) and IAN of sham surgery rats. In axotomized rats, expression levels increased significantly from day 2 to day 28 post-axotomy, with peaks on days 14 (Slit1) and 7 (Robo2) after axotomy (relative to sham: Slit1 in TG P < 0.0005, Slit1 in IAN P = 0.003, Robo2 in TG P < 0.0005, and Robo2 in IAN P < 0.0005). Over-expressed Slit1 and Robo2 in both the TG and IANs of axotomized rats did not return to sham levels during the 28-day observation period of this study. The regeneration and functional recovery of axotomized IAN was evaluated by jaw opening reflex (JOR) recorded before and after axotomy. JOR occurrence (0% on day 7, 35% on day 14, and 85% on day 28) increased gradually, and the relative threshold of electrical stimulation eliciting JOR decreased gradually (1000.0 ± 0.0% on day 7, 854.3 ± 132.5% on day 14, and 302.6 ± 92.3% on day 28). On day 28 after axotomy, JOR occurrence and the relative JOR threshold had almost returned to those of sham rats. These findings suggest that Slit1 and Robo2 are involved in the regeneration and functional recovery of the axotomized IAN.
The inferior alveolar nerve (IAN) is located within a bony canal in the mandible, in close proximity to the root tips of the mandibular molar teeth. This nerve can be damaged during the removal of third molars or as a result of orthognathic surgery or mandibular fracture. Many of these injuries may allow for complete sensory reinnervation of the denervated tissues.
Previous studies have examined the reinnervation of teeth after IAN axotomy but have not evaluated the mechanisms underlying IAN regeneration. A better understanding of the mechanisms of IAN regeneration is vital to the development of new therapeutic strategies.
During axonal regeneration following nerve injury, axonal guidance is controlled by environmental cues. Recently, studies have shown that peripheral nerve injury causes changes in axon guidance cues and immunoreactivity within the primary sensory neurons and peripheral nerves. Previous studies have shown that Slit proteins are important guidance cues and that their interaction with Robo receptors plays an important role in neuronal migration, axonal elongation, and axonal branching. Although Slit1 and Robo2 are known to be involved in neuronal development at various early embryonic stages, whether Slit1 and its receptor Robo2 play a role in regeneration and functional recovery of the adult injured IAN following axotomy is unknown.
The objective of this study was to analyze changes in expression pattern of Slit1 and Robo2, and to clarify the relationship between these changes and spontaneous functional recovery of the axotomized IAN without repair, using a rat IAN axotomy model. To evaluate the functional recovery of the axotomized IAN, the jaw opening reflex (JOR) was measured before and after axotomy.
Materials and methods
Animal surgery and tissue preparation
All animals were treated according to the guidelines of animal care. A total of 60 male Sprague–Dawley rats weighing 250–300 g were used. For the immunoprecipitation and immunohistochemical analyses, 35 rats were anaesthetized intraperitoneally with sodium pentobarbital (50 mg/kg). To expose the bilateral IAN of each rat, a small incision was made in the skin covering the masseter muscle; the masseter muscle was partially separated and the alveolar bone was exposed. The bone surface was removed using a dental drill, and a mandibular canal was fenestrated. Once the IAN was exposed, it was carefully separated from the surrounding vasculature and transected. The cut ends of the axotomized IAN were replaced carefully into the mandibular canal, and the muscle and skin were closed and sutured. The axotomized rats were allowed to recover and survive for 2, 7, 14, and 28 days ( n = 7 for each period). Rats without IAN axotomy were used as a sham group ( n = 7); in this group, the skin was incised, the masseter separated and the mandibular canal fenestrated. All rats were given the antibiotic penicillin G procaine (Phoenix Pharmaceutical, Inc., St. Joseph, MO, USA) the day before, the day of, and the day after surgery (30,000 U/250 kg). After axotomy, rats were fed with powdered food.
Seven of the axotomized rats were euthanized on days 2, 7, 14, and 28 following axotomy; the non-axotomized rats were euthanized on day 0 ( n = 7, sham rats). Rats were euthanized using an overdose of sodium pentobarbital (>50 mg/kg, intraperitoneally), after which both the trigeminal ganglion (TG) and hemimandibles were quickly harvested bilaterally. In preparation for immunohistochemical analysis, the right sides of each rat’s TG and hemimandible were immediately flash-frozen in dry ice. Hard tissues from the right sides of the harvested hemimandibles were decalcified for 3 weeks using 10% ethylenediaminetetraacetic acid (EDTA) in 0.1 M phosphate buffer. The right sides of both the TGs and the decalcified hemimandibles were stored at −80 °C until sectioning. The left sides of both the TGs and the distal stumps of the axotomized IANs of all rats were rapidly collected and frozen in liquid nitrogen to prepare for immunoprecipitation analysis. These tissues were also stored at −80 °C.
For the electrophysiological analysis of JOR, a unilateral axotomy of the right IAN was performed on 25 adult rats.
Immunoprecipitation analysis
Frozen tissue samples were homogenized in lysis buffer (1× Tris-buffered saline (TBS), 1% Nonidet P-40, 0.5% sodium deoxycholate, 0.1% sodium dodecylsulphate (SDS), 0.004% sodium azide, phenylmethanesulphonyl fluoride (PMSF), protease inhibitor cocktail, sodium orthovanadate, dimethyl sulphoxide (DMSO), water) (#sc24948; Santa Cruz Biotechnology, USA). Homogenates were centrifuged for 15 min at 4 °C (14,000 rpm), after which supernatants were collected. Supernatants (500 μg/200 μl) were incubated overnight at 4 °C with lysis buffer containing polyclonal goat anti-Slit1 (#sc26597; Santa Cruz) and polyclonal rabbit anti-Robo2 (#sc25673; Santa Cruz) diluted at 200 μg/ml. In negative controls, supernatants were incubated in lysis buffer without the addition of antibodies. The supernatants from these experiments were then mixed and incubated using protein G-magnetic beads for 30 min at room temperature (#8740; Cell Signaling Technology, USA). Protein G antibody–protein complexes were precipitated using a magnetic separation rack, then washed three times with lysis buffer, resuspended in lithium dodecylsulphate (LDS) sample buffer, boiled for 10 min, and analyzed by Western blotting.
Immunoprecipitated proteins were electrophoresed on 4–12% NuPAGE Bolt gels and transferred onto polyvinylidene difluoride (PVDF) membranes using the iBlot Dry Blotting System (Invitrogen, Carlsbad, CA, USA). Membranes were blocked with 5% non-fat milk solution in Tris-buffered saline containing 0.1% Tween (TBS-Tw) for 1 h at room temperature and incubated with polyclonal goat anti-Slit1 and polyclonal rabbit anti-Robo2 diluted at 1:100 (Invitrogen). Blots were rinsed, exposed to peroxidase-coupled secondary antibodies, diluted at 1:3000, washed, and developed using an Amersham ECL-Advanced Western Blotting Detection Kit (GE Healthcare, Buckinghamshire, UK). The intensity of each signal and quantification of the relative protein levels were measured using ImageJ software. The relative protein levels in the axotomized rats on each post-axotomy day were compared to those of the sham rats. The values for each post-axotomy time point were calculated as the percentage of the mean of shams.
Immunohistochemistry of the TG
The TG tissue that was collected for immunohistochemical study was frozen in dry ice and cut into 20-μm sections using a cryostat (Leica Instruments, Wetzlar, Germany). TG tissue sections were fixed in 4% paraformaldehyde in 0.1 M phosphate-buffered saline (PBS), rinsed, pre-treated for antigen retrieval with 0.1 M PBS containing 1% SDS, rinsed, and immersed for 1 h in 0.1 M PBS containing 0.3% Triton X-100 and 10% normal donkey or 10% normal goat serum. The sections were incubated overnight at 4 °C with polyclonal goat anti-Slit1, polyclonal rabbit anti-Robo2, monoclonal mouse anti-S100α/β (#sc130110; Santa Cruz), and monoclonal mouse anti-glial fibrillary acidic protein (GFAP) (#3670; Cell Signaling) (diluted 1:100 in 0.1 M PBS). These antibodies were detected with Alexa Fluor 633 goat anti-mouse (#A21050; Invitrogen), Alexa Fluor 488 donkey anti-goat (A11055; Invitrogen), Alexa Fluor 555 goat anti-rabbit (A21428; Invitrogen), and Alexa Fluor 488 goat anti-rabbit (A11008; Invitrogen) secondary antibodies, respectively. Tissue sections were counterstained with 4′,6-diamidino-2-phenylindole (DAPI) and were evaluated under a fluorescence microscope (Nikon Eclipse Ni) connected to a CCD camera (Nikon DS-Fi1c). For quantification of Slit1 and Robo2 expression, images (magnification 40×) of three defined regions of interest per tissue, each measuring 62,500 μm 2 were obtained (Nikon Eclipse Ni) and the intensity of fluorescence was analyzed using Metamorph Image Analysis software (version 6.3; Molecular Devices, Sunnyvale, CA, USA). Three sections were processed for each animal. The mean fluorescence intensity values were calculated using data from the three sections analyzed. Images were analyzed by an independent assistant who was blinded to the study conditions.
Immunohistochemistry of the hemimandible
The decalcified hemimandibles that were spared for immunohistochemical analysis were pre-fixed in 10% formaldehyde solution, decalcified with 10% EDTA in 0.1 M phosphate buffer for 3 weeks, and embedded in paraffin blocks. Sections of 5 μm thickness were cut using a microtome. IAN sections were deparaffinized and fixed in 4% paraformaldehyde in 0.1 M PBS, rinsed, pre-treated for antigen retrieval using 0.1 M PBS containing 1% SDS, and then rinsed and immersed for 1 h in 0.1 M PBS containing 0.3% Triton X-100 and 10% normal donkey or 10% normal goat serum. The sections were incubated with the antibodies described above and were then evaluated using fluorescence microscopy. For quantification of Slit1 and Robo2 expression, images (magnification 40×) of three defined regions of interest per tissue, each measuring 62,500 μm 2 were obtained (Nikon Eclipse Ni) and the intensity of fluorescence was analyzed using Metamorph Image Analysis software. Three sections were processed for each animal. The mean fluorescence intensity values were calculated using data from the three sections analyzed. Images were analyzed by an independent assistant who was blinded to the study conditions.
Electrophysiological analysis of the jaw opening reflex
To assess the functional recovery of the axotomized IAN, the JOR, elicited by electrical stimulation of the IAN, was measured before IAN axotomy (sham, n = 4) and on days 7, 14, and 28 following axotomy (each group n = 7). The electrophysiological analysis of the JOR has been described in a previous study. Briefly, each rat was deeply anaesthetized and the trachea cannulated for respiration. Continuous inhalation of 0.5–1.0% halothane (AstraZeneca, Kings Langley, Hertfordshire, UK) was used to maintain a stable depth of anaesthesia. Next, a pair of silver wire electrodes (inter-electrode distance = 0.5 mm; diameter = 0.2 mm) was inserted into the right mandibular canal (0.5 mm proximal or distal to the axotomized site) to electrically stimulate the right IAN. The electrodes were kept in place by fixation to the adjacent mandibular bone with light-cured dental resin. A hook-type enamel steel wire electrode was implanted into the digastric muscle, along the direction of the muscle fibres, to record its evoked electromyographic (EMG) activity. The distal and proximal regions from the axotomized site were stimulated electrically, and the evoked EMG activity was recorded from the digastric muscle. A single rectangular pulse (duration = 0.2 ms, intensity = 0.01–1.0 mA, frequency = 1 Hz) was used for IAN stimulation. The stimulus intensity was increased gradually, and the lowest intensity that constantly evoked digastric EMG activity was determined as the threshold of electrical intensity that could elicit the JOR. To attain comparable responses, the stimulus intensity current was adjusted to 1–10 times the threshold in each rat. The evoked threshold intensities caused by the stimulation of the distal or proximal regions were measured to calculate the relative value of the JOR threshold. In axotomized rats, if the JOR threshold was 10 times higher than the threshold intensity following electrical stimulation of the proximal site of the axotomized IAN, the JOR threshold was calculated as 1000%.
The JOR occurrence in the axotomized rats was defined to measure the digastric EMG activity elicited by the electrical stimulation of the axotomized IAN. The EMG activity was amplified by a laboratory-made amplifier. All signals were stored on a personal computer at a sampling rate of 5000/s and were later analyzed offline with Spike2 software for Windows, version 7.05 (Cambridge Electronic Design Limited, Science Park, Milton Road, Cambridge, UK). After the electrophysiological recordings had been made, the rats were euthanized using an overdose of sodium pentobarbital. The digastric muscle was then dissected to confirm the location of the electrodes.
Statistical analysis
All data were analyzed using PASW Statistics 18 for Windows statistical software (SPSS Inc., Chicago, IL, USA). Significant differences between groups were determined using the Kruskal–Wallis test. The data are reported as median values ± min–max values; P -values less than 0.05 were considered statistically significant.
Results
Slit1 protein is expressed in neuronal and non-neuronal cells of the TG in adult non-axotomized rats and is up-regulated following IAN axotomy
Slit1 detected by immunoprecipitation analysis was expressed at low levels in the TGs of sham rats and was up-regulated following axotomy. Compared to the samples taken from sham rats, Slit1 signal intensity was significantly higher at 2, 7, 14, and 28 days following axotomy (relative to shams, P = 0.015, P = 0.008, P < 0.0005, and P = 0.035, respectively) ( Fig. 1 a). Slit1 signal intensity was highest at 14 days after axotomy.

Immunostaining revealed that Slit1 expression in TG neurons was increased at 2, 7, 14, and 28 days following IAN axotomy as compared to the samples taken from sham rats. Significantly increased Slit1 expression was observed in the TG of axotomized rats 2 days post-axotomy and it continued to increase until 14 days post-axotomy. At day 14 post-axotomy, the expression levels gradually decreased but did not return to the levels of sham rats by day 28 following IAN axotomy. Trigeminal ganglia consist of heterogeneous sensory neurons, satellite glial cells, Schwann cells, macrophages, and fibroblasts. To further determine whether the up-regulation of Slit1 was also from glial cells, double immunostaining of Slit1 either with S-100 or GFAP, both of which are markers for satellite glial cells and Schwann cells in TG, was performed. As shown in Fig. 1 b and 1c, a few Slit1 immunostaining cells were also co-localized with satellite glial cells surrounding the neurons, indicating that IAN axotomy activates satellite glial cells in TG to release Slit1. When compared to the samples taken from sham rats, Slit1 staining intensity was significantly higher at 2, 7, 14, and 28 days following IAN axotomy (relative to shams, all P < 0.0005) ( Fig. 1 d).
Slit1 protein is expressed in the IAN of adult non-axotomized rats and is up-regulated following IAN axotomy
Slit1 detected by immunoprecipitation analysis was expressed at low levels in the IANs of sham rats and was up-regulated following axotomy. Compared to the samples taken from sham rats, Slit1 signal intensity was significantly higher in the distal stumps of axotomized IANs at 2, 7, 14, and 28 days following axotomy (relative to shams, P = 0.023, P = 0.006, P < 0.0005, and P = 0.027, respectively) ( Fig. 2 a). Slit1 signal intensity was highest at 14 days after axotomy.

Immunostaining revealed that the Slit1 expression (green) in the distal stumps of axotomized IANs was increased at 2, 7, 14, and 28 days following IAN axotomy as compared to the samples taken from sham rats. Significantly increased Slit1 expression was observed in the distal stumps of axotomized IANs at 2 days post-axotomy and it continued to increase until 14 days post-axotomy. At day 14 post-axotomy, the expression levels gradually decreased but did not return to the sham levels by day 28 following IAN axotomy. Double immunostaining revealed that Slit1 and S100 were co-localized in some non-neuronal cells including reactive Schwann cells in the distal stump of axotomized IANs that underwent Wallerian degeneration at day 14 following axotomy ( Fig. 2 b). It can be inferred that IAN axotomy activates Schwann cells in the distal stump of the axotomized IAN to release Slit1. When compared to the samples taken from sham rats, Slit1 staining intensity was significantly higher at 2, 7, 14, and 28 days following axotomy (relative to shams, all P = 0.003) ( Fig. 2 c).
Robo2 protein is expressed in the neuronal and non-neuronal cells of TGs in adult non-axotomized rats and is up-regulated following IAN axotomy
Robo2 detected by immunoprecipitation analysis was expressed at low levels in TGs of sham rats and was up-regulated following axotomy. Compared to the samples taken from sham rats, Robo2 signal intensity was significantly higher at 2, 7, 14, and 28 days following axotomy (relative to shams, P = 0.007, P < 0.0005, P = 0.006, and P = 0.023, respectively) ( Fig. 3 a). Robo2 signal intensity was highest at 7 days after axotomy.
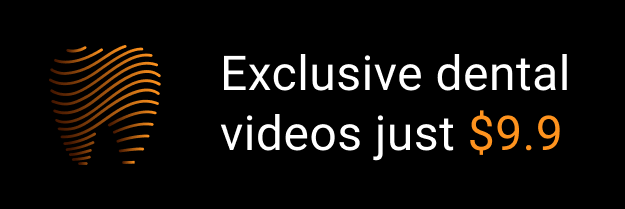