CAMELIA ALB
FLORIN ALB
Chapter V
CERAMICS USED IN ESTHETIC RESTORATIONS
CERAMICS USED IN ESTHETIC RESTORATIONS
Introduction
Ceramics are currently the only materials capable of reproducing the natural qualities of human teeth as perfectly as possible, and are also the most biocompatible of all dental materials. However, two obstacles have limited the application of all-ceramic systems in all types of restorations for quite some time: their low flexural resistance, and the laboratory technology needed, which is far more complex and time consuming than with metal-ceramic or composite restorations.1
The important progress made over the past two decades – especially the improvement of the mechanical properties of high-strength ceramics and the development of new, simplified laboratory procedures – have led to better reproducibility of all-ceramic restorations. This has increased their clinical use over the metal-ceramic technique by over 50% in some countries. The trend is to continually extend the application of all-ceramic systems in all fields of dentistry – both for their esthetic results, as well as for the reduced manufacturing costs, which are currently lower for a CAD/CAM-produced, all-ceramic crown than for a metal-ceramic one.2 We can confidently assert that in due course all-ceramic systems will dominate restorative dentistry.
Numerous ceramic systems have appeared in recent years, from the most conservative ones that can be used even without any tooth preparation, to those with applications in extended edentulous spaces. These systems have different properties and use different laboratory materials and technologies. They therefore have diverse clinical indications, which could become confusing for the general practitioner who is not an expert in the field of dental materials. This chapter provides details on the existing ceramic systems and how they are used in clinical practice. Its aim is to help practitioners to better understand the all-ceramic systems that are currently available.
5.1.1 A short history
The term “ceramic” is of Greek etymology, deriving from “keramos”, which means “burnt clay”. The basic components of ceramic used to be clays (argils), which produced solid (but brittle) objects, such as ceramic pots, when heated in a furnace. The technology for manufacturing porcelain (the most noble of ceramic products) was developed in China in 2697 BC and reached Europe (via Venice) only at the end of the 15th century.3
The first attempts to manufacture artificial ceramic teeth for dentures are attributed to the French pharmacist Alexis Duchateau in 1774. In 1844, Samuel Stockton White started the large-scale production of ceramic teeth for removable dentures in Philadelphia, USA. In 1886, Charles H Land (known as the father of porcelain dentistry) made the first ceramic inlay on a platinum foil after an impression had been taken in a patient’s mouth. In 1903 in Detroit, Land also produced the first full-coverage ceramic crown (the jacket crown) made of a high-fusing ceramic fired on a platinum foil over a die, which had very low fracture resistance and was recommended only for anterior teeth.
At the beginning of the 20th century, the results with these ceramic products were poor, mainly due to insufficient knowledge regarding their properties and behavior. This led to flaws appearing during the sintering process and a lack of adhesion between ceramic restorations and the dental tissues. Furthermore, weak phosphate cements were being used to lute them. Their popularity declined even more in the 1940s with the discovery of acrylic resins. Ceramics were less and less used until clinical failures of acrylic resins began to appear (eg, reduced resistance to wear and color changes over time).
In 1962, Weinstein and Weinstein patented a new type of ceramic based on leucite, an aluminosilicate with a high coefficient of thermal expansion (CTE). This discovery made the ceramic CTE closer to the CTE of the alloy, leading to metal-ceramic fixed restorations with improved properties. Therefore, the 1960s are considered the beginnings of metal-ceramics.4
An important step in improving the esthetic properties of ceramics was the introduction of ceramic sintering under a vacuum, which reduced the porosity and led to a more homogeneous product with a higher translucency.
5.1.2 Structure and properties of ceramics
Ceramic is an inorganic material made of metal elements (Al, Ca, Mg, K) and non-metal elements (Si, O, B, F) which form oxides, nitrides, borides, or silicates, as well as complex mixtures of these materials. The intermolecular bonding in ceramics can be ionic or covalent; their different proportions producing changes in their chemical properties. Most ceramics (not only dental porcelain) are obtained by heating up a powder, or a liquid in which powder particles have been dissolved, to a temperature where the particles will merge, forming a solid mass. The result is a class of materials with specific properties: high fusing temperatures, strength, rigidity (although brittle), high abrasion resistance (but weak in tension), high compression resistance, and good electrical and thermal insulators. These materials can be modified through additives, thus obtaining an infinite range of colors.5 The clinical relevance of the molecular structure of ceramics is reflected in the optical properties of each ceramic type: when the glass matrix (the noncrystalline phase) dominates, the ceramic will be more translucent, and vice versa; ie, when the crystalline content is higher, the ceramic will be more opaque.6
Generally, when dentists use the word “ceramic,” it refers only to a limited class of materials used for indirect prosthetic restorations. It is important to note that, based on structure and properties, there are other examples of “unconventional” ceramics with a range of applications in dentistry, such as ceramics used in the composition of investment materials, as inorganic fillers for composite resins, or glass-ionomer cements (GICs).5
5.1.3 The composition of dental ceramics
The ceramics for general use in cookware, computer parts, and heat shields in vehicles are argil ceramics (also known as “pottery ceramics” or clays). These are composed of three basic elements: feldspar (the main component); quartz, and kaolin in various percentages. Dental porcelain is different from ceramics in general use, and although initially it used to contain a small percentage of kaolin (4% to 5%), this has been completely eliminated in modern ceramics.
As with the majority of glass products, dental ceramics contain minerals such as feldspar (K2OAl2O36SiO2) (80%); important quantities of silica, the most widespread mineral on earth (SiO2) (14% to 18%); reduced quantities of alumina (Al2O3) (2%); and traces of other oxides that significantly influence their properties.
In addition to the basic components, other substances are found in dental ceramics:
• Pigments providing the color of the ceramic: titanium oxide for yellow/brown shades; manganese oxide for violet; iron oxide for brown; copper oxide for green; and cobalt oxide for blue.
• In the past, uranium oxides used to be added to obtain the fluorescence of ceramic, but because of their reactivity, they have been replaced with lanthanide oxides.7
To conclude, modern dental ceramics have two basic components: an amorphous glass matrix – made of the silica network – and a crystalline phase. The latter determines the mechanical, physical, chemical, and optical properties of dental ceramics. The ratio between the two components varies; the higher the glass content, the higher the translucency, but the lower the resistance to crack propagation. Generally, the ceramic used for all-ceramic systems will contain between 35% and 90% of the crystalline phase, for improved mechanical properties.7
The first generations of feldspathic ceramics (known today as conventional or traditional feldspar ceramics) had several disadvantages: a sintering contraction higher than 40%; a high fracture risk (therefore they were used only for anterior teeth); and a low flexural resistance. They were, however, also very hard and rigid, although brittle. New techniques, as well as materials with increased resistance to tension and flexion, have been researched and developed.
5.1.4 Properties of dental ceramics
•Very high elasticity modulus (alumina = 380 MPa).
•High compression strength (150 to 900 MPa).
•Low resistance to traction (20 to 60 MPa).
•Lacks resistance to fracture.
•The maximum plastic deformation it can withstand is 0.1%.
•These materials are extremely sensitive to surface microcracks.
• The hardness of conventional ceramics was 460 to 660 VHN (Vickers hardness number), so they used to be harder than natural tooth enamel, generating the abrasion of opposing teeth. The new ceramics with low fusing temperatures have values of 380 VHN, very close to natural enamel. The ceramics used for the core (eg, alumina) have a hardness of 1,200 VHN, which is why they are always covered by a feldspar or vitreous ceramic.5
• Average density equals 1.0 to 3.8 g/cm3 – the density determines the weight of a ceramic restoration (which is less than the weight of a metal structure).
• Ceramic is a refractory material with a very high fusing temperature.
• The coefficient of thermal expansion (CTE) has reduced values: 1 to 15 × 10-6/°C. This is an advantage for the all-ceramic crowns as it acts as a good insulator, but becomes an inconvenience for metal-ceramic crowns. This is why low-fusing ceramics are used, with a melting temperature of about 100°C to 200ºC lower than the solidus temperature of the alloy.
The optical properties of ceramics greatly interest patients because they directly correlate with the esthetic result. As mentioned in the section on the composition of dental ceramics (5.1.3), translucency decreases with more crystalline phase. Thus, the best optical properties belong to the glass ceramics (Empress, Dicor), as well as the feldspathic ceramics, as translucency is lower and opaqueness higher for more resistant ceramics with a greater crystalline phase – such as the infiltrated ceramics (spinell, alumina, zirconia).6
The chemical properties
• Ceramics have low reactivity and are considered an inert material in the oral environment.
• Ceramics are not affected by large variations in oral pH, ie, they are not attacked by acids that exist in the oral cavity.5
Biological properties
• Ceramics are dental materials with a very good biocompatibility, being almost totally inert to the tissues of the oral cavity. Glazed ceramic is a plaque-resistant material and is thus periodontally friendly.
5.2 CERAMICS USED IN DENTISTRY
As there are many all-ceramic systems on the dental materials market, several classifications have been made in an attempt to organize them. Criteria used for classifications were: the fusing temperature; ceramics composition; laboratory technology; and their clinical indications.
Classification based on the fusing temperature
•High fusing temperature – over 1,300°C.
•Average fusing temperature – 1,100°C to 1,300°C.
•Low fusing temperature – 850°C to 1,100°C.
•Very low fusing temperature – 680°C to 850°C.7
Classification based on ceramic composition
•Category 1 – glass-ceramics (contain mainly silica or quartz).
•Category 2 – glass-ceramics with crystalline filling (usually leucite or another type of high-fusing temperature glass).
•Category 3 – crystalline ceramics with glass fillers (mainly alumina).
•Category 4 – polycrystalline ceramics (alumina and zirconia).8
Classification based on laboratory technology
Additive systems:
•Layering technique (Optec HSP, Vitadur, Duceram LFC).
•Castable ceramics (Cerapearl, Dicor).
•Infiltration and sintering (In-Ceram).
•Pressing technique (PS Empress, Cerestore, Optec, OPC, Cerapress).
Subtractive systems:
•Mechanical milling.
•Computerized milling: CAD/CAM.
Classification based on their clinical indications
•Veneering ceramics – generally layered over the metal structure or an oxide ceramic core.
•Ceramics for the framework of removable partial dentures.
•Ceramics for suprastructures on implants.
The authors have decided to describe in detail only two of the classifications after Giordano and McLaren,8 believing that these will facilitate the understanding of the differences between the various ceramic systems.
5.2.1 Types of ceramics based on their microstructure
There are four large classes:
•Category 1 – glass-ceramics (contain mainly silica).
•Category 2 – glass-ceramics with crystalline fillers (usually based on leucite or another type of glass with a high fusing point).
•Category 3 – crystalline ceramics (mainly alumina with a glass matrix).
•Category 4 – polycrystalline ceramics (alumina and zirconia).9
5.2.1.1 Amorphous glass-ceramics
5.2.1.2 Glass-ceramics with secondary crystalline phase
The glass matrix is identical to that in amorphous glass-ceramics, the difference being the amount and the type of crystals introduced in the ceramic, or grown in the glass phase. The most commonly used ceramic crystals today are leucite, lithium disilicate, and fluorapatite.8,10 These materials are used in blocks for the CAD/CAM technique for CEREC (Sirona Siemens) – Vitablocs Mark II (Vita Zahnfabrik) – because they have the lowest failure rate in inlays and onlays (approximately 1% per year) (Figs 5-1a to 5-1c).
Low-leucite content ceramics
Low-leucite content ceramics – often incorrectly called feldspar ceramics – are used as veneering materials over the metal framework, over ceramic copings, or for ceramic veneers using the refractory die technique. The first ceramic powder introduced on the market had a variable size and distribution of leucite crystals, and had a very low flexural resistance. The new materials contain a homogeneous distribution of leucite crystals, being less abrasive and having a high flexural resistance. These materials are used especially for producing metal-ceramic crowns, an example being Vita VM 13 (Vita Zahnfabrik)9 (Figs 5-2a to 5-2d).
Fig 5-1 a–c Clinical case: IPS e.max crown on tooth 21. (Images courtesy of Dr Florin Lăzărescu.)
High-leucite content glass-ceramics
High-leucite content glass-ceramics (approximately 50%) start as a glass matrix around some leucite crystals. At the second thermal treatment, they grow in size and form crystals that will block crack propagation, thus improving their mechanical and physical properties, such as high resistance to fracture (120 MPa). These materials are better known under their commercial names, such as: Empress (Ivoclar Vivadent), Finesse (Dentsply), PM9 (Vita), as well as Empress CAD milling blocks used for CEREC and E4D (Figs 5-3a and 5-3b).
Fig 5-2 a–d Clinical case before and after cementation of metal-ceramic crowns.
Lithium disilicate-based glass-ceramic
Lithium disilicate-based glass-ceramic, with a 70% crystalline component and a higher resistance to flexion (around 360 MPa), was first introduced by Ivoclar as Empress II. It is currently available as IPS e.max, with two versions: one for pressing, and the other for CAD/CAM milling. Although it has a high ratio of crystalline phase, the material is translucent enough to be used in esthetic restorations (veneers, crowns) because of the low refraction index of the lithium disilicate crystals. Furthermore, because of its high mechanical resistance, it can be used as a ceramic core that will be layered with a special ceramic containing fluorapatite crystals.
Fig 5-3 a, b Clinical case before and after cementing of an Empress ceramic bridge 32 to 42. (Images courtesy of Dr Ionut Brânzan.)
5.2.1.3 Crystalline ceramic with interpenetrating phase
Crystalline ceramic with an interpenetrating phase was introduced in 1988 by the Vita Company as the InCeram system, which contained at least two components that intertwined and expanded from depth to surface. First, a porous matrix was obtained, which was later “saturated” with the second-phase glass (lanthanum aluminosilicate glass) through capillarity. This system was introduced as an alternative to the metal-ceramic because it has a high-strength ceramic core (350 to 650 MPa flexural resistance)10 that can be layered with veneering material (VM). Today, these crystalline alumina copings can be produced either by sintering the alumina onto a duplicated die, or by milling it in a CAD/CAM machine (Vita InCeram Alumina for inLab).
5.2.1.4 Single-phase polycrystalline ceramics
Single-phase polycrystalline ceramics contain only one crystalline component and are obtained by the sintering of the crystals that do not have a glass matrix, such as aluminum oxide (alumina) and zirconium dioxide (zirconia). This system was first introduced by Procera (Nobel Biocare); the ceramic powder being compressed, then milled and sintered at 1,600°C, generating a very thick and resistant core (600 MPa), but with a 20% contraction after sintering, which leads to a poor marginal fit. Another single-phase ceramic is zirconium dioxide, which is not used in its pure form, but is partially stabilized by the addition of other oxides – the majority of zirconium dioxide-based ceramics used in dentistry contain a 3% addition of yttrium.11 Its resistance to flexion is about 900 to 1,100 MPa, higher than any other dental ceramic, so extended restorations can be made, including full bridge, complex dental implant-supported frameworks. Partial removable dentures can also be produced, even in functional stress areas, just like metal frameworks12 (Figs 5-4a to 5-4d and 5-5a and 5-5b).
Practical applications
Some practical applications of the classification based on microstructures are:
• The ceramics from categories 1 and 2 can be etched with hydroflouric acid, so they can create a better adhesive bonding, while those from groups 3 and 4 cannot be etched, so their adhesive forces are lower (Figs 5-6a to 5-6d and 5-7a to 5-7d).
• The glass content in a ceramic material makes it more transparent, which means it can be used in the esthetic area, although it has a lower flexural resistance. On the opposite end of the spectrum, polycrystalline solids have the best mechanical properties, but they are very opaque and are therefore mainly used as the core or infrastructure in extended bridges, as well as in functional stress areas with other veneering ceramics.8,9
Fig 5-4 a–d Clinical case before and after the IPS e.max crown on tooth 11. (Images courtesy of Dr Ionut Brânzan).
Fig 5-5 a, b Clinical case before and after cementing InCeram crowns on teeth 21 and 22. (Images courtesy of Dr Ionut Brânzan).
Fig 5-6 a–d Clinical case before and after cementing microveneers on the maxillary incisors.
Fig 5-7 a Laminate veneers made on refractory dies.
< div class='tao-gold-member'>
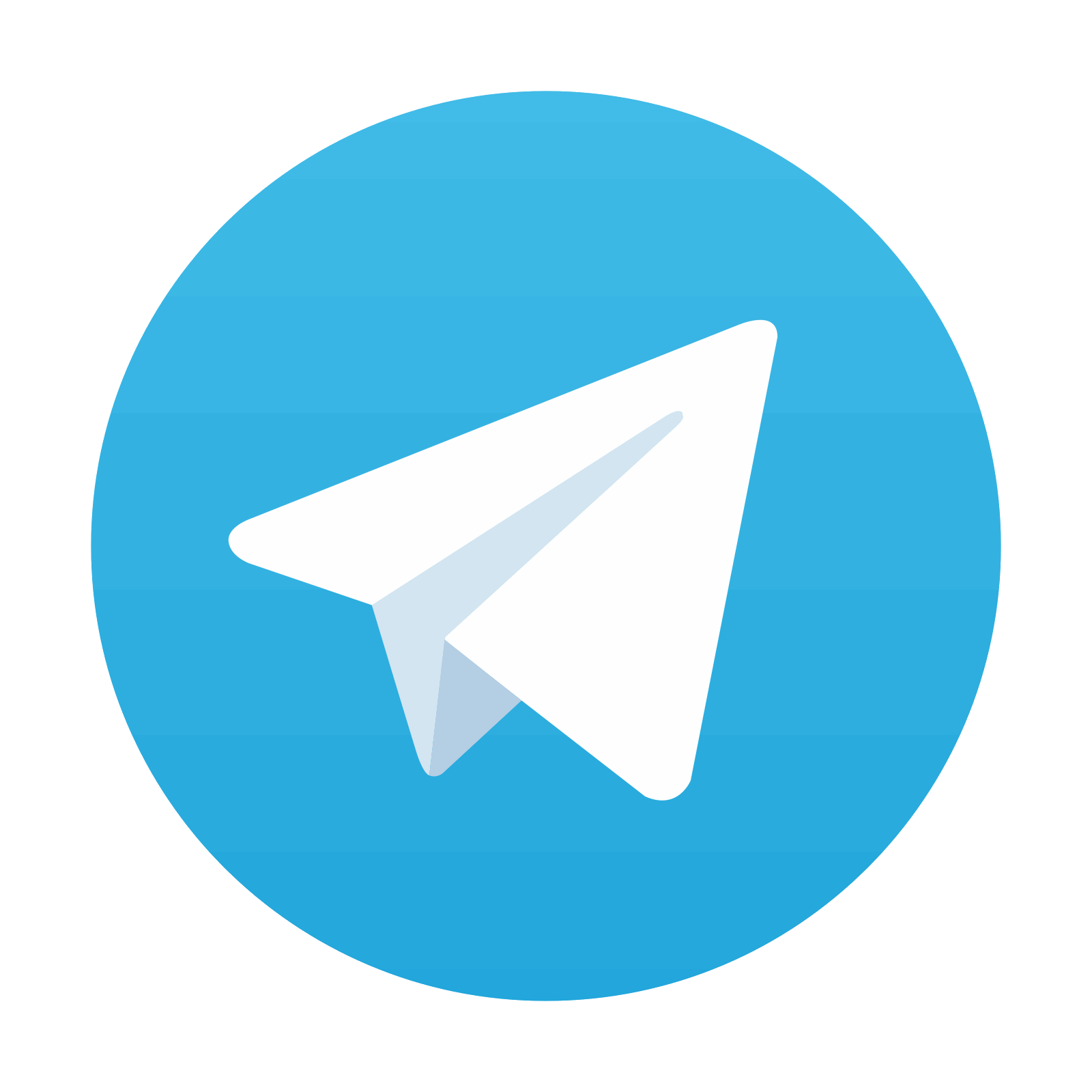
Stay updated, free dental videos. Join our Telegram channel

VIDEdental - Online dental courses
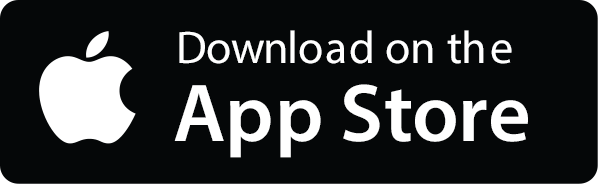
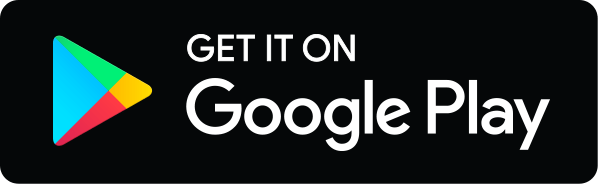