Abstract
Objective
To adequately address clinically important issues such as osseointegration and soft tissue integration, we screened for the direct biological cell response by culturing human osteoblasts and gingival fibroblasts on novel zirconia-based dental implant biomaterials and subjecting them to transcriptional analysis.
Methods
Biomaterials used for osteoblasts involved micro-roughened surfaces made of a new type of ceria-stabilized zirconia composite with two different topographies, zirconium dioxide, and yttria-stabilized zirconia (control). For fibroblasts smooth ceria- and yttria-stabilized zirconia surface were used. The expression of 90 issue-relevant genes was determined on mRNA transcription level by real-time PCR Array technology after growth periods of 1 and 7 days.
Results
Generally, modulation of gene transcription exhibited a dual dependence, first by time and second by the biomaterial, whereas biomaterial-triggered changes were predominantly caused by the biomaterials’ chemistry rather than surface topography. Per se, modulated genes assigned to regenerative tissue processes such as fracture healing and wound healing and in detail included colony stimulating factors ( CSF2 and CSF3 ), growth factors, which regulate bone matrix properties (e.g. BMP3 and TGFB1 ), osteogenic BMPs ( BMP2 / 4 / 6 / 7 ) and transcription factors ( RUNX2 and SP7 ), matrix collagens and osteocalcin, laminins as well as integrin ß1 and MMP-2.
Significance
With respect to the biomaterials under study, the screening showed that a new zirconia-based composite stabilized with ceria may be promising to provide clinically desired periodontal tissue integration. Moreover, by detecting biomarkers modulated in a time- and/or biomaterial-dependent manner, we identified candidate genes for the targeted analysis of cell-implant bioresponse during biomaterial research and development.
1
Introduction
Clinical experience in conjunction with several in vitro studies has shown that physicochemical and topographical properties of novel implant biomaterials desired for interactions with biological interfaces significantly affect initial response of target cells. Furthermore, the aforementioned properties play a crucial role for tissue integration during implant healing (reviewed in Refs. ). Therefore, the development process of innovative implants should particularly consider tissue-convenient chemical compositions and surface topographies, to adequately address cell and tissue compatibility in view of the prospective clinical application. In the clinical context, desirable implant properties in addition to osseointegration should support soft tissue integration, since loss of dental implants often occurs in response to bacterial-challenged bone loss, the latter emerging from the leakiness of the gingival soft tissue barrier function. To seek for such desired implant properties, cell-based in vitro studies with respect to dental materials in general have proven to be precious screening tools , as they allow to circumvent the complexity of the in vivo situation, which is characterized by a plethora of cell and tissue interactions. Hence, in vitro cell systems, which are targeted to evaluate implant chemistry-related and implant surface-related support of soft tissue as well as osseointegration, result not only in cost and time savings, but also lead to less animal testing .
In the context of implant biomaterial development for hard- and soft tissue integration, a new ceria-stabilized zirconia-based composite (Ce-TZP) was developed within the European project named LongLife to overcome Low Temperature Degradation (LTD) of yttria-stabilized zirconia ceramics (Y-TZP) , while maintaining high toughness and strength. Moreover, the LongLife project aimed at creating zirconia-based surfaces with modifications, which improve not only osseo- but also soft tissue integration.
During implant healing, bone matrix synthesis by osteoblasts and the subsequent formation of new bone directly on the implant surface represent a prerequisite for successful hard tissue integration and should therefore be supported by the implant surface . In addition to peri-implant bone healing, soft tissue integration (STI) of implants is achieving more and more importance, since, as said before, the attachment of gingival connective tissue to the transcutaneous part of the implant (abutment), plays a vital role in the formation and maintenance of a proper seal between the oral environment and the peri-implant bone. This connective tissue interface, with the gingival fibroblasts in particular, functions as barrier for junctional epithelial depth migration and prevents bacterial invasion that can finally lead to gingival recession or pocket formation, and inflammation driven bone resorption, causing finally implant loss . For this reason, the expression of genes encoding for biomolecules that initiate and maintain the adhesion of gingival soft tissue to the abutment surface is of particular importance during peri-implant healing. In this context, a previous in vitro study, which employed target cells, identified putative STI-relevant genes in gingival soft connective tissue fibroblasts. Herein, putative STI-associated genes include cell functions such as (1) the ECM formation, (2) cell adhesion, (3) protease driven ECM turn over, and (4) inflammation . Moreover, Mendonca et al. have shown modulation of genes encoding for biomolecules of osteogenic differentiation in human mesenchymal stem cells in response to different implant surfaces . With respect to such work it should be noted that transcriptional analysis enables for the detection of the most direct cell response to a novel implant biomaterial.
We in the present study tested the hypothesis whether alterations of mRNA levels of up to 90 genes could be detected as a direct response of implant-relevant target cells, namely primary human osteoblasts derived from alveolar bone (AO) and gingiva fibroblasts (GF) to the novel zirconia-based surfaces, and whether gene expression will be modulated by time, i.e. the study-innate culture periods of 1 and 7 days.
The panel of genes under study encode for biomarkers generally found in regenerative tissue processes such as fracture healing and wound healing. In particular, they assign for cell attachment, proliferation, differentiation, ECM formation and turnover, as well as inflammation. As indicated above, test biomaterials were made of a recently developed innovative composite, in which equiaxial α-Al 2 O 3 and elongated SrAl 12 O 19 phases are dispersed in a ceria-stabilized zirconia matrix (Ce-TZP) . The specimens had three different surface configurations in case of osteoblasts, namely (i) dense Ce-TZP, (ii) porous Ce-TZP and (iii) hydrophilic ZrO 2 coating, and exclusively dense Ce-TZP surfaces in case of fibroblasts. Since from a clinical point of view, rough implant surfaces better support osteoblast-triggered hard tissue integration , roughened surfaces with R a ≈ 0.6 μm for osteoblast cultures were employed. On the other hand, smooth surfaces with a R a threshold of 0.2 μm impede unwanted biofilm formation, and are therefore favorable for soft tissue integration . Thus, smooth biomaterials with aforementioned R a values were prepared for fibroblasts. In this context it should be noted that with respect to the roughened surfaces employed in our study we observed a good plaque performance, i.e. no favoritism of biofilm formation with matched already used implant surfaces (unpublished data). Since the just described biomaterials represent novel implant surfaces, we included the clinically established yttria-stabilized zirconia (Y-TZP), which served as a reference in our study.
2
Materials and methods
2.1
Zirconia sample preparation and surface treatment
The disks used as substrates for cell culture were prepared from tetragonal partially stabilized zirconium dioxide containing 3% of yttrium oxide (Y-TZP) by uniaxial pressing. After pre-sintering the surface of disks for osteoblast culturing, substrates were machined with conventional hard metal tools to obtain an arithmetic mean roughness R a of about 0.6 μm. The disks for fibroblast culturing were not machined leaving them with the as-pressed surface roughness of 0.2 μm. To compare conventional Y-TZP to the Ce-TZP-based composite material while retaining the same topography, a coating of both materials was applied by the same procedure on pre-sintered (fibroblasts) or pre-sintered and machined (osteoblasts) substrates of Y-TZP. Depending on the coating material the obtained samples for osteoblasts are named Y-TZP AO and Ce-TZP AO1 , those for fibroblasts Y-TZP GF and Ce-TZP GF . The coatings were applied by spraying of a colloidal suspension of the respective ceramic powders. The suspensions were prepared by ball milling of the ceramic powders using zirconia milling media, a poly acrylic acid as dispersant and polyethylene glycol as binder. When the coating was applied on the pre-sintered substrate, the homogeneous shrinkage of both the substrate and the coating allowed both being densified and tightly linked. The thickness of the dense coating was a few microns. For Ce-TZP with a porous surface structure (Ce-TZP AO2 ), the Ce-TZP-based composite material was applied on sintered substrates of Y-TZP. When the coating was densified, shrinkage was only allowed in one direction due to the already dense substrate contributed to an increased porosity of the coating. In order to obtain a hydrophilic surface modification for osteoblast cultures, pure zirconium dioxide (ZrO 2 ) coatings were deposited on samples with a dense Ce-TZP composite coating like Ce-TZP AO1 . These coatings were prepared by direct current magnetron sputtering (DCMS) of pure zirconium targets in an argon–oxygen atmosphere. The relative oxygen flow was adjusted to obtain sputtering in the fully oxidized mode. The coating thickness was (90 ± 20) nm and the initial water contact angle 10° ± 3°. Zirconia disks were sterilized by low-temperature hydrogen peroxide gas plasma sterilization STERRAD 100/100S (Advanced Sterilization Products [A.S.P], Johnson & Johnson Medical, Irvine, CA).
2.2
Surface analysis
The surface morphology and topography of the zirconia-based disks were analyzed by scanning electron microscopy (LEO435VP scanning electron microscope, Zeiss, Oberkochen, Germany) and interferometry (MicroXam; Phaseshift, Tucson, USA). Evaluations were made at three different areas on the disk surfaces, and values measured by interferometry (IFM) were averaged. To characterize the surface in height, spatial and surface enlargement three parameters were selected: S a describes the average height deviation in μm, S ds measures the density of summits over the evaluated area in 1/mm 2 , and S dr describes the surface enlargement compared to a totally flat reference area in % or as a ratio. Before parameter calculation, a digital (Gaussian) filter of 50 × 50 μm was applied in order to remove errors of form and waviness. The specimens used for scanning electron microscopy (SEM) were sputter coated with gold-palladium for 60 s at 60 mA (SCD050; Balzers, Liechtenstein).
2.3
Isolation and cultivation of osteoblasts and fibroblasts
Human osteoblasts were prepared from alveolar bone explants obtained from a 42-year old healthy male patient during implant site preparation procedure. Primary cultures of human gingiva fibroblasts were obtained from a 38-year old healthy male donor as described previously . The collection of dental bone and gingiva samples was approved by the Ethics Committee of the Albert-Ludwigs-University, Freiburg, Germany (vote Nr. 411/08_121010). Human alveolar bone osteoblasts (AO) and gingival fibroblasts (GF) were cultured in Dulbecco’s Modified Eagle’s Medium (PAA Laboratories, Coelbe, Germany) supplemented with 1% (w/v) glutamine (Gibco, Invitrogen, Karlsruhe, Germany), 10% (w/v) fetal calf serum (Biochrom AG, Berlin, Germany) and 0.2% (w/v) (50 μg/ml) kanamycin (Sigma–Aldrich, Taufkirchen, Germany). The cells were maintained in a humidified 37 °C incubator with 5% CO 2 . All experiments were carried out with AO of passage 6 and GF of passage 9. For gene expression analysis of short-term cultures 3 × 10 4 AO and 2 × 10 4 GF cells per disk were seeded on three disks per material/surface (triplicate) and lysed for mRNA isolation at day 1. For AO and GF cells cultured for 7 days, 0.8 × 10 4 cells were seeded per disk. In this manner, it was possible to yield sufficient mRNA amounts for the subsequent gene expression analysis while ensuring comparable cell densities of the respective cell cultures.
2.4
Analysis of gene transcription
The relative gene expression of up to 90 genes was determined on the mRNA level by quantitative RT-PCR (qPCR) using the RT 2 Profiler PCR Array technology (Qiagen, Hilden, Germany). Total RNA of three samples per material/surface was pooled and isolated after 1 and 7 days culture period (RNeasy Mini Kit; Qiagen), and analyzed with the Experion Automated Electrophoresis System (Bio-Rad Laboratories, Munich, Germany). Synthesis of cDNA was performed with 259 ng RNA for AO and 228 ng for GF cells using the RT 2 PreAmp DNA Synthesis Kit (Qiagen) according to the manufacturer’s protocol. In order to get sufficient cDNA for the following qPCR, one quarter of the cDNA previously obtained from reverse transcription (equivalent to 65 ng of total RNA for AO and 57 ng for GF) was pre-amplified using the RT 2 PreAmp cDNA Synthesis Kit together with the RT 2 PreAMP Pathway Primer Mix (Qiagen). Real time PCR reactions were carried out and analyzed with the CFX96 Real-Time PCR Detection System (Bio-Rad Laboratories) using the RT 2 SYBR Green qPCR Master Mix from Qiagen. Analysis of relative gene expression in AO was performed with the Human Osteogenesis RT 2 Profiler PCR Array (Qiagen, Cat. PAHS-026Z), and in GF with a Custom PCR Array based on the Extracellular Matrix and Adhesion Molecules RT 2 Profiler PCR Array (Qiagen, Cat. PAHS-013Z). The customized PCR Array for GF additionally included four further genes, namely CD34 molecule ( NM_001773 ), Lysyl oxidase ( NM_002317 ), H3 histone, family 3A ( NM_002107 ) and Interleukin 6 (interferon, beta 2) ( NM_000600 ). qPCR of the arrays was performed according to the manufacturer’s instructions. The data obtained from AO experiments were normalized to the housekeeping genes beta actin ( ACTB , NM_001101 ), beta-2-microglobulin ( B2M , NM_004048 ), Glyceraldehyde-3-phosphate dehydrogenase ( GAPDH , NM_002046 ), Hypoxanthine phosphoribosyltransferase 1 ( HPRT1 , NM_000194 ), Ribosomal protein, large, P0 ( RPLP0 , NM_001002 ), and analyzed using the comparative ΔΔCT method using the RT 2 Profiler PCR Array Data Analysis version 3.5. Since ACTB and GAPDH were modulated in GF during culture, relative gene expression in GF was normalized to B2M , HPRT1 and RPLP0 , and as described for AO analyzed by the comparative ΔΔCT method.
2.5
Statistical analysis
The cell culture experiments were performed in triplicate in three independent experiments (biological replicates). Statistical evaluation of qRT-PCR data was performed by using the PCR Array Data Analysis software which included the Student’s t-test for identifying statistically significant differences between gene expression levels. Data obtained by interferometry were analyzed using One-Way-ANOVA followed by Tukey’s post hoc test (p < 0.05).
2
Materials and methods
2.1
Zirconia sample preparation and surface treatment
The disks used as substrates for cell culture were prepared from tetragonal partially stabilized zirconium dioxide containing 3% of yttrium oxide (Y-TZP) by uniaxial pressing. After pre-sintering the surface of disks for osteoblast culturing, substrates were machined with conventional hard metal tools to obtain an arithmetic mean roughness R a of about 0.6 μm. The disks for fibroblast culturing were not machined leaving them with the as-pressed surface roughness of 0.2 μm. To compare conventional Y-TZP to the Ce-TZP-based composite material while retaining the same topography, a coating of both materials was applied by the same procedure on pre-sintered (fibroblasts) or pre-sintered and machined (osteoblasts) substrates of Y-TZP. Depending on the coating material the obtained samples for osteoblasts are named Y-TZP AO and Ce-TZP AO1 , those for fibroblasts Y-TZP GF and Ce-TZP GF . The coatings were applied by spraying of a colloidal suspension of the respective ceramic powders. The suspensions were prepared by ball milling of the ceramic powders using zirconia milling media, a poly acrylic acid as dispersant and polyethylene glycol as binder. When the coating was applied on the pre-sintered substrate, the homogeneous shrinkage of both the substrate and the coating allowed both being densified and tightly linked. The thickness of the dense coating was a few microns. For Ce-TZP with a porous surface structure (Ce-TZP AO2 ), the Ce-TZP-based composite material was applied on sintered substrates of Y-TZP. When the coating was densified, shrinkage was only allowed in one direction due to the already dense substrate contributed to an increased porosity of the coating. In order to obtain a hydrophilic surface modification for osteoblast cultures, pure zirconium dioxide (ZrO 2 ) coatings were deposited on samples with a dense Ce-TZP composite coating like Ce-TZP AO1 . These coatings were prepared by direct current magnetron sputtering (DCMS) of pure zirconium targets in an argon–oxygen atmosphere. The relative oxygen flow was adjusted to obtain sputtering in the fully oxidized mode. The coating thickness was (90 ± 20) nm and the initial water contact angle 10° ± 3°. Zirconia disks were sterilized by low-temperature hydrogen peroxide gas plasma sterilization STERRAD 100/100S (Advanced Sterilization Products [A.S.P], Johnson & Johnson Medical, Irvine, CA).
2.2
Surface analysis
The surface morphology and topography of the zirconia-based disks were analyzed by scanning electron microscopy (LEO435VP scanning electron microscope, Zeiss, Oberkochen, Germany) and interferometry (MicroXam; Phaseshift, Tucson, USA). Evaluations were made at three different areas on the disk surfaces, and values measured by interferometry (IFM) were averaged. To characterize the surface in height, spatial and surface enlargement three parameters were selected: S a describes the average height deviation in μm, S ds measures the density of summits over the evaluated area in 1/mm 2 , and S dr describes the surface enlargement compared to a totally flat reference area in % or as a ratio. Before parameter calculation, a digital (Gaussian) filter of 50 × 50 μm was applied in order to remove errors of form and waviness. The specimens used for scanning electron microscopy (SEM) were sputter coated with gold-palladium for 60 s at 60 mA (SCD050; Balzers, Liechtenstein).
2.3
Isolation and cultivation of osteoblasts and fibroblasts
Human osteoblasts were prepared from alveolar bone explants obtained from a 42-year old healthy male patient during implant site preparation procedure. Primary cultures of human gingiva fibroblasts were obtained from a 38-year old healthy male donor as described previously . The collection of dental bone and gingiva samples was approved by the Ethics Committee of the Albert-Ludwigs-University, Freiburg, Germany (vote Nr. 411/08_121010). Human alveolar bone osteoblasts (AO) and gingival fibroblasts (GF) were cultured in Dulbecco’s Modified Eagle’s Medium (PAA Laboratories, Coelbe, Germany) supplemented with 1% (w/v) glutamine (Gibco, Invitrogen, Karlsruhe, Germany), 10% (w/v) fetal calf serum (Biochrom AG, Berlin, Germany) and 0.2% (w/v) (50 μg/ml) kanamycin (Sigma–Aldrich, Taufkirchen, Germany). The cells were maintained in a humidified 37 °C incubator with 5% CO 2 . All experiments were carried out with AO of passage 6 and GF of passage 9. For gene expression analysis of short-term cultures 3 × 10 4 AO and 2 × 10 4 GF cells per disk were seeded on three disks per material/surface (triplicate) and lysed for mRNA isolation at day 1. For AO and GF cells cultured for 7 days, 0.8 × 10 4 cells were seeded per disk. In this manner, it was possible to yield sufficient mRNA amounts for the subsequent gene expression analysis while ensuring comparable cell densities of the respective cell cultures.
2.4
Analysis of gene transcription
The relative gene expression of up to 90 genes was determined on the mRNA level by quantitative RT-PCR (qPCR) using the RT 2 Profiler PCR Array technology (Qiagen, Hilden, Germany). Total RNA of three samples per material/surface was pooled and isolated after 1 and 7 days culture period (RNeasy Mini Kit; Qiagen), and analyzed with the Experion Automated Electrophoresis System (Bio-Rad Laboratories, Munich, Germany). Synthesis of cDNA was performed with 259 ng RNA for AO and 228 ng for GF cells using the RT 2 PreAmp DNA Synthesis Kit (Qiagen) according to the manufacturer’s protocol. In order to get sufficient cDNA for the following qPCR, one quarter of the cDNA previously obtained from reverse transcription (equivalent to 65 ng of total RNA for AO and 57 ng for GF) was pre-amplified using the RT 2 PreAmp cDNA Synthesis Kit together with the RT 2 PreAMP Pathway Primer Mix (Qiagen). Real time PCR reactions were carried out and analyzed with the CFX96 Real-Time PCR Detection System (Bio-Rad Laboratories) using the RT 2 SYBR Green qPCR Master Mix from Qiagen. Analysis of relative gene expression in AO was performed with the Human Osteogenesis RT 2 Profiler PCR Array (Qiagen, Cat. PAHS-026Z), and in GF with a Custom PCR Array based on the Extracellular Matrix and Adhesion Molecules RT 2 Profiler PCR Array (Qiagen, Cat. PAHS-013Z). The customized PCR Array for GF additionally included four further genes, namely CD34 molecule ( NM_001773 ), Lysyl oxidase ( NM_002317 ), H3 histone, family 3A ( NM_002107 ) and Interleukin 6 (interferon, beta 2) ( NM_000600 ). qPCR of the arrays was performed according to the manufacturer’s instructions. The data obtained from AO experiments were normalized to the housekeeping genes beta actin ( ACTB , NM_001101 ), beta-2-microglobulin ( B2M , NM_004048 ), Glyceraldehyde-3-phosphate dehydrogenase ( GAPDH , NM_002046 ), Hypoxanthine phosphoribosyltransferase 1 ( HPRT1 , NM_000194 ), Ribosomal protein, large, P0 ( RPLP0 , NM_001002 ), and analyzed using the comparative ΔΔCT method using the RT 2 Profiler PCR Array Data Analysis version 3.5. Since ACTB and GAPDH were modulated in GF during culture, relative gene expression in GF was normalized to B2M , HPRT1 and RPLP0 , and as described for AO analyzed by the comparative ΔΔCT method.
2.5
Statistical analysis
The cell culture experiments were performed in triplicate in three independent experiments (biological replicates). Statistical evaluation of qRT-PCR data was performed by using the PCR Array Data Analysis software which included the Student’s t-test for identifying statistically significant differences between gene expression levels. Data obtained by interferometry were analyzed using One-Way-ANOVA followed by Tukey’s post hoc test (p < 0.05).
3
Results
3.1
Characterization of zirconia-based biomaterial surfaces
In Fig. 1 , SEM micrographs of biomaterials destined for osteoblast-triggered hard ( Fig. 1 A–D) and fibroblast-associated soft tissue integration ( Fig. 1 E and F) revealed distinct differences in terms of surface architecture. Except Ce-TZP AO2, all examined biomaterials, displayed similar compact and grainy surface texture ( Fig. 1 A, B, D, E and F), whereas Ce-TZP AO2 disks had a porous surface layer with apparently high interconnectivity of the pores ( Fig. 1 C). Regarding surface topography parameters, height deviation (S a values) and surface enlargement (S dr values) of osteoblast-destined biomaterials were generally higher ( Table 1 , S a range = 0.33–0.49 μm; S dr = 4.51–26.91%) rather than for fibroblast-specific surfaces ( Table 1 , up to 0.20 μm for S a and 3.88% for S dr ). These differences revealed rougher surface properties for osteoblast surfaces with matched fibroblast samples. Furthermore, among all examined surfaces Ce-TZP AO2 displayed the highest number of peaks per surface area and surface enlargement ( Table 1 , S ds = 168 728/mm 2 and S dr = 26.91%), thereby indicating it as highly fissured. In the present study, osteoblast surfaces with different chemical composition and similar surface topography were Y-TZP AO ( Fig. 1 A), Ce-TZP AO1 ( Fig. 1 B) and ZrO 2 ( Fig. 1 D), while surfaces with equal chemistry and differential topography were Ce-TZP AO1 ( Fig. 1 B), and Ce-TZP AO2 ( Fig. 1 C). For fibroblasts, biomaterials under study namely Y-TZP GF and Ce-TZP GF differed in surface chemistry, while surface topography was equal.
Symbol | S a [μm] ± SD | S ds (1/mm 2 ) ± SD | S dr (%) ± SD |
---|---|---|---|
Y-TZP AO | 0.57 (0.07) | 106 412 (1368) | 5.85 (0.58) |
Ce-TZP AO1 | 0.49 (0.13) | 123 624 (12 650) | 4.51 (0.79) |
Ce-TZP AO2 | 0.36 * (0.02) | 168 728 * (1274) | 26.91 * (1.45) |
ZrO 2_AO | 0.66 (0.07) | 105 194 (5317) | 7.92 (1.27) |
Y-TZP GF | 0.20 † (0.04) | 135 320 † (13 665) | 3.59 (0.40) |
Ce-TZP GF | 0.15 ‡ (0.00) | 157 527 *,‡ (1015) | 3.88 (0.13) |
* p < 0.05 statistically significant differences between Ce-TZP and matched Y-TZP.
† p < 0.05 statistically significant differences between Y-TZP GF and Y-TZP AO .
‡ p < 0.05 statistically significant differences between Ce-TZP GF and Ce-TZP AO1 (One Way ANOVA followed by Tukey’s post hoc test).
3.2
Detection of osteoblast response established on different zirconia surfaces by PCR array transcription
In order to examine early impact of novel implant biomaterials in the context of osseointegration, we examined osteoblast bioresponse to Ce-TZP AO1 , Ce-TZP AO2 and ZrO 2 surfaces by gene transcription analysis at days 1 and 7, and compared resulting expression profiles with those obtained from clinically established Y-TZP as control biomaterial. The results from this biomaterial comparison yielded distinct modulation of 28 genes on ZrO 2 surfaces when compared to Y-TZP and Ce-TZP ( Table 2 and Supplementary material S1), including the significant upregulation of cytokine genes, involved in immune cell activation ( Table 2 ). By contrast, gene profiles on both Ce-TZP surfaces were comparable with matched Y-TZP control and showed only slight modulation of 11 genes in total (S1).
Symbol | Description | Y-TZP versus | Ce-TZP AO1 versus | Ce-TZP AO2 versus | |||||||||
---|---|---|---|---|---|---|---|---|---|---|---|---|---|
Ce-TZP AO1 | Ce-TZP AO2 | ZrO 2 | Ce-TZP AO2 | ZrO 2 | ZrO 2 | ||||||||
d1 | d7 | d1 | d7 | d1 | d7 | d1 | d7 | d1 | d7 | d1 | d7 | ||
NOG | Noggin | – | – | – | – | – | −1.8 | – | – | – | −2.1 * | – | −1.6 |
RUNX2 | Runt-related transcription factor 2 | – | – | – | – | – | −1.6 | – | – | – | −1.5 * | – | – |
CSF2 | Colony stimulating factor 2 (granulocyte-macrophage) | – | −1.7 | – | −1.5 | 8.0 * | 2.5 | – | – | 7.5 * | 4.4 | 7.1 * | 3.9 |
CSF3 | Colony stimulating factor 3 (granulocyte) | n.d. | n.d. | n.d. | n.d. | 12.4 * | n.d. | – | – | 15.8 * | n.d. | 16.9 * | n.d. |
IGF1 | Insulin-like growth factor 1 | – | – | – | – | – | −2.7 | – | – | – | −3.8 * | 1.8 | −3.1 |
ICAM1 | Intercellular adhesion molecule 1 | – | – | – | – | 1.9 | 2.0 | – | – | 2.1 | – | 2.1 * | 1.8 |
COL5A1 | Collagen, type V, alpha 1 | – | – | – | – | – | −1.9 | – | – | – | −2.0 * | – | −1.7 |
COL15A1 | Collagen, type XV, alpha 1 | – | – | – | – | −1.7 | −4.2 | – | – | −1.9 * | −4.1 * | −1.7 * | −4.6 |
COMP | Cartilage oligomeric matrix protein | – | – | – | 2.0 | −2.7 | −4.1 | – | – | −2.2 | −5.7 * | −2.4 * | −7.9 * |
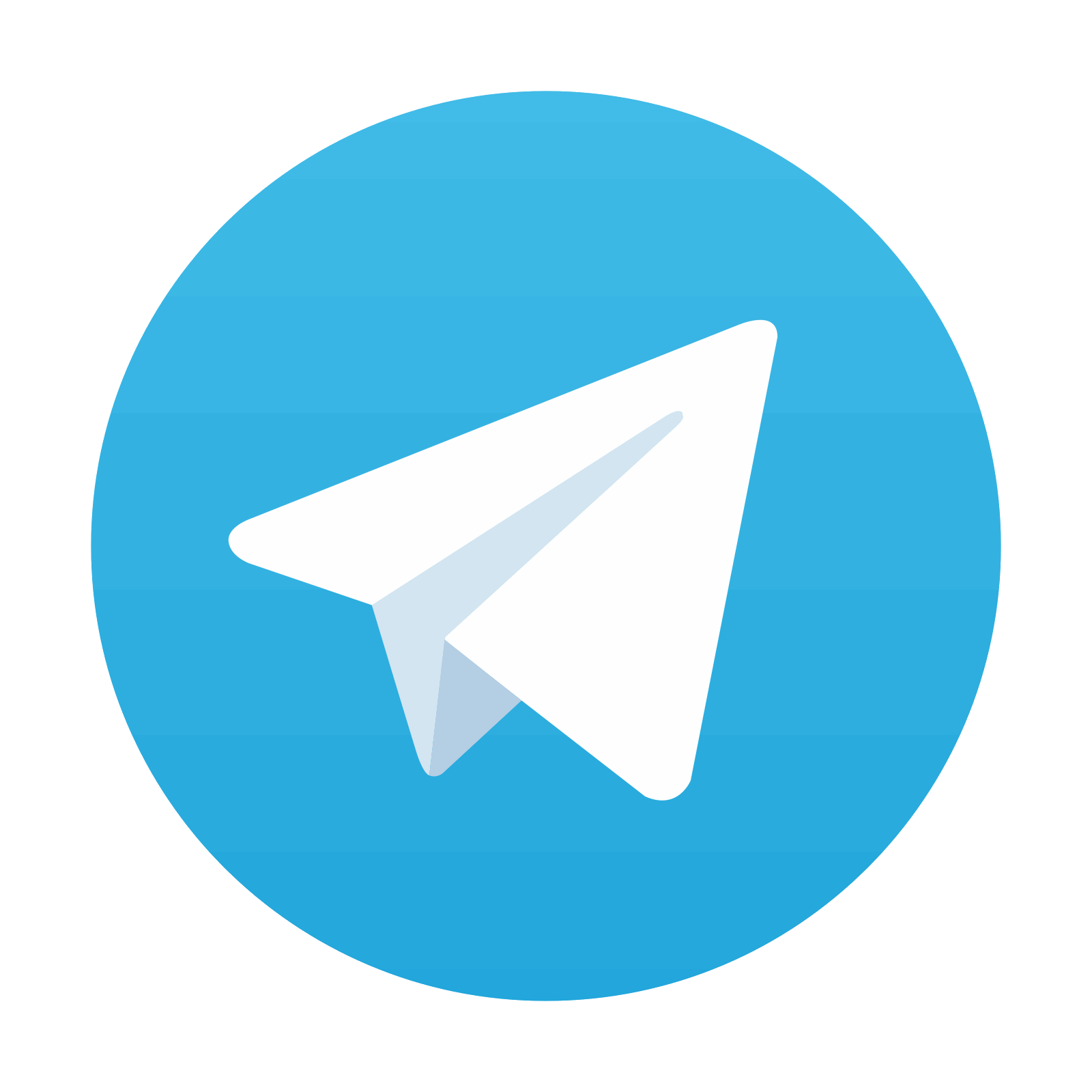
Stay updated, free dental videos. Join our Telegram channel

VIDEdental - Online dental courses
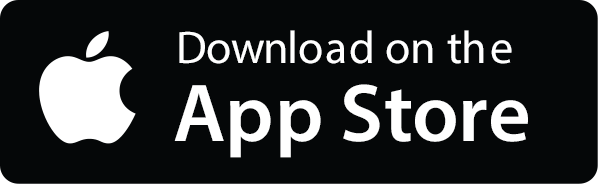
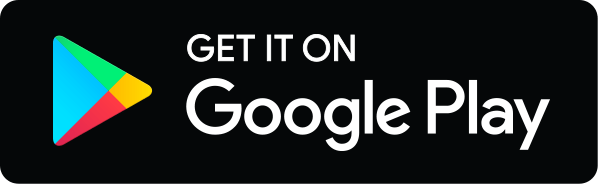
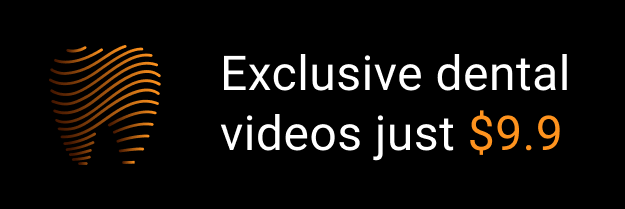