Abstract
Objectives
To optimize the compressive fracture strength ( σ ) and elastic modulus ( E ) of a glass-ionomer (GI) restorative using poly(acrylic) acid (PAA) weight average molecular weight ( M w ) mixtures.
Methods
174 PAA solutions were prepared (four control PAA M w s at three PAA concentrations (25, 35 and 45%) ( n = 12) and six M w mixtures (Groups A–F at nine blend ratios and three PAA concentrations ( n = 162))). The viscosity ( η ) of each PAA solution was determined using a digital viscometer. The PAA solutions were hand-mixed with a commercial GI restorative powder (Ionofil Molar; Voco, Cuxhaven, Germany) and σ and E were determined using cylindrical (6 mm height, 4 mm diameter) specimens ( n = 20) at 24 h. Data were analyzed using analyses of variance (ANOVA) (three-, two- and one-way) and regression analyses at p < 0.05.
Results
The three- and two-way ANOVAs highlighted significant effects of M w mixture, blend ratio and PAA concentration (all p < 0.0001) on the η , σ and E data. Regression analyses showed significant increases in η , σ and E ( p < 0.0001) with increasing M w from Groups A to F. There was no significant effect of blend ratio on the σ data for Groups A ( p = 0.178), D ( p = 0.747) and F ( p = 0.107) and on the E data ( p > 0.083).
Significance
The current approach to improving the mechanical properties of GI restoratives using PAA M w mixtures is encouraging, however, further manipulation of the GI restorative system by optimizing PAA M w mixtures, blend ratios and PAA concentrations is required to elicit further improvements in σ and E without impacting upon the η of the PAA solution.
1
Introduction
Glass-ionomers (GIs) are formed by an acid–base reaction between a proton donating polymeric acid (typically polyacrylic acid (PAA)) and an ion-leachable basic calcium fluoro-alumino-silicate glass powder . The acid attacks the periphery of the glass particles releasing calcium, fluoride and aluminum ions from the glass surface . As the reaction continues, the polymeric chains unwind as the negative charge increases and the polymer chains are cross-linked by the calcium and aluminum ions causing the cement to harden. The set GI can be regarded as a composite structure where the glass particles act as the filler in the salt-like polymeric matrix . Increasing the filler content of a GI increases the mechanical properties and the viscosity of the cementitious mass which increases the indications of GIs from a luting cement to a cavity liner and base or a posterior restorative . Additionally, the mechanical properties of the GI can be further improved by optimizing the polymeric matrix, namely the PAA weight average molecular weight ( M w ) , the PAA concentration and the PAA polydispersity index (PDi) . As a result, any improvement in the polymeric matrix could result in increased mechanical properties for the GI for a given filler volume fraction.
Polymers typically consist of a network of randomly orientated chain-like molecules which exist in entangled coil formations when the polymer is in a softened (melted) or solid state . The polymer chain entanglement theory, proposed by Edwards , considered a polymer chain to be contained within a theoretical tube formed by neighboring chains . Therefore, to break a single polymer chain and cause chain scission, a chain firstly needs to be stretched . However, any movement of the polymer chains laterally and longitudinally is restricted by the entanglements and the longer the polymer chain length the more stress that is required to remove it from its tube, which results in increased polymer strength . The research of Hill et al. and Wilson et al. , based on GIs produced from an anhydrous PAA, proposed that a critical weight average molecular weight ( M crit ) existed for GIs where more stress would be required to remove a chain from its theoretical tube than that required to cause chain scission. Additionally, Hill et al. suggested that an entanglement weight average molecular weight ( M ent ) existed (for anhydrous PAAs) below which the polymeric constituent in the resultant GI would fail to form entanglements. Using conventional PAA solutions to produce GIs, rather than the anhydrous PAAs used previously , the current authors previously proposed M crit to be lower (80,000) than the 100,000 theoretically predicted by Hill et al. and M ent to be below 5000 compared with the 7000 suggested for GIs prepared using anhydrous PAAs .
When using PAA solutions to prepare GIs, the viscosity of the liquid is increased with increasing PAA M w and PAA concentration . The increased viscosity of the PAA solutions used for conventional GI formulations exacerbates the difficulties associated with hand-mixing compared with the water or weak tartaric acid solution (<10% m/m) employed for anhydrous GI formulations. It has recently been shown that an optimum PAA concentration (in solution) existed for individual PAA M w s where the compressive fracture strengths and elastic moduli of the resultant conventional GIs were maximized . However, the relationship between PAA concentration and PAA M w was not a simple one owing to the PAA viscosity effects . The current study investigated six PAA M w mixtures prepared from four control PAAs with M w s which encompassed a range above M ent and at M crit identified for conventional GI solutions . The purpose of the study was to optimize the compressive fracture strengths and elastic moduli of GI restoratives using PAA M w mixtures.
2
Materials and methods
2.1
Materials
The powder constituent of a commercial hand-mixed GI restorative (Ionofil Molar; Voco GmbH, Cuxhaven, Germany; Lot No. 0947058, shade A3) was used to prepare all specimens in the current study. The four control PAAs were supplied as powders and were reported by the manufacturer to have M w s of 15,000 (P15), 25,700 (P26), 40,000 (P40) and 80,800 (P81) ( Table 1 ). The M w and number average molecular weight ( M n ) of the four control PAAs were previously quantified using gel permeation chromatography and the PDis were calculated by dividing M w by M n ( Table 1 ).
PAA | Manufacturer | M w | M n | PDi |
---|---|---|---|---|
P15 | Advanced Healthcare Limited, Tonbridge, England | 15,250 (1567) | 8680 (691) | 1.76 |
P26 | Robert Hill, Queen Mary, University of London, England | 25,910 (1863) | 13,680 (1550) | 1.89 |
P40 | Advanced Healthcare Limited, Tonbridge, England | 40,156 (2717) | 21,930 (2422) | 1.83 |
P81 | Robert Hill, Queen Mary, University of London, England. | 80,650 (5400) | 41,840 (3069) | 1.93 |
2.2
Polyacrylic acid solution preparation
Twenty-four hours prior to usage, the four control PAAs (P15, P26, P40 and P81) were prepared in solution at concentrations of 25, 35 and 45% by dispensing the PAA powder (0.5, 0.7 or 0.9 g, respectively) into 2 mL of distilled water at room temperature. Additionally, the six powder mixtures of the four control PAAs (Group A: P15 and P26; Group B: P15 and P40; Group C: P15 and P81; Group D: P26 and P40; Group E: P26 and P81 and Group F: P40 and P81) were dry blended at powder:powder ratios of 10:90, 20:80, 30:70, 40:60, 50:50, 60:40, 70:30, 80:20 and 90:10. Solutions of the M w mixtures (Groups A–F) at the dry blend ratios investigated were also prepared, 24 h before use, in accordance with the procedure for the control PAA solutions prepared at concentrations of 25, 35 and 45%.
2.3
Viscosity determination
A digital viscometer (Brookfield DV-E Viscometer; Brookfield Engineering Laboratories Inc., Middleboro, MA, USA) containing a small sample adaptor was employed to measure the viscosity ( η ) of the four control PAA solutions prepared at concentrations of 25, 35 and 45%. Additionally, the η of the six M w mixtures (Groups A–F), dry blended at five powder:powder ratios (10:90, 30:70, 50:50, 70:30, and 90:10) prepared at three PAA concentrations (25, 35 and 45%) were also determined. The inner chamber of the small sample adaptor was filled with 2.1 mL of a PAA liquid solution and a spindle was inserted carefully to avoid trapping air bubbles in the PAA solution. The viscometer rotated the spindle at 100 rpm in the PAA solution and the η in mPa s was recorded. The η for each of the 102 groups investigated (six M w mixtures × five blend ratios × three PAA concentrations ( n = 90) and four control PAAs × three PAA concentrations ( n = 12)) were determined on three occasions and the mean η was recorded.
2.4
GI restorative preparation
A powder to liquid mixing ratio of 4:1 (g:g) was used for each GI restorative group under investigation in accordance with manufacturers’ recommendations for Ionofil Molar. To prepare the GI, a glass slab was placed on a balance (Sartorius Expert; Sartorius AG, Goettingen, Germany) and 0.188 g of the PAA liquid solution was dispensed onto one end of the glass slab using an adjustable pipette (Gilson, Middleton, WI, USA). Ionofil Molar powder (0.75 g) was weighed onto the opposite end of the glass slab and separated into two parts. The first part of the powder was hand-mixed with the PAA liquid solution for 20 s using a stainless steel spatula. The remaining powder was added and hand-mixed so that the total mixing time was 40 s.
2.5
Compression testing protocol
To determine the compressive fracture strength, cylindrical specimens (6.0 ± 0.1 mm height and 4.0 ± 0.1 mm diameter) were prepared using a polytetraflouroethylene (PTFE) split-mold assembly . A PTFE base plate was covered with an acetate strip and the PTFE split-mold was placed on top and aligned using a 4 mm diameter holding pin. Two nylon sliding wedges were fitted to one side of the mold to ensure equal pressure was applied along the length of the split-mold . To minimize the incorporation of air bubbles into the specimens, the largest convenient portion of the GI was conveyed to one side of an individual hole in the split-mold and allowed to flow into the mold . A second acetate strip was placed on top of the filled split-mold assembly and a glass slab was applied to isolate the specimens from the surrounding atmosphere. The mold assembly was clamped using G-clamps to apply equal pressure to all specimens and ensure parallelism of the cylindrical specimen ends for uniform contact with the compressive platens of the testing apparatus . The specimens were maintained in a water-bath maintained at 37 ± 1 °C for 1 h. The mold was disassembled, the specimens removed and specimens which contained visible defects such as chipped edges, non-parallel ends or pores were discarded. The remaining specimens were stored in glass containers filled with 50 mL of distilled water in an incubator (Climate Cabinet; Firlabo, Meyzieu, France) at 37 ± 1 °C for a further 23 h. Twenty nominally identical cylindrical GI restorative specimens were manufactured for each of the 174 experimental groups under investigation (six M w mixtures × nine blend ratios (10:90, 20:80, 30:70, 40:60, 50:50, 60:40, 70:30, 80:20 and 90:10) × three PAA concentrations ( n = 162) and four control PAAs × three PAA concentrations ( n = 12)).
Prior to compressive testing, the mean diameter of each cylindrical specimen was determined from three measurements taken using a digital micrometer reading to 1 μm (Mitutoyo, Kawasaki, Japan). To mimic the oral environment, the specimens were tested ‘wet’ by placing a piece of wet filter paper (Whatman No. 1; Whatman PLC, Kent, England) on the flat ends of each cylindrical specimen in accordance with ISO 9917-1:2003 . A compressive load was applied to the long axis of the cylindrical specimen using a universal testing machine (Instron Model 5565, High Wycombe, England) operating at a cross-head speed of 1 mm/min and the maximum load at failure was recorded.
The compressive fracture strength ( σ ) in MPa of each cylindrical specimen was determined by dividing the fracture force by the original cross-sectional area
σ = 4 F f π d 2
where F f is the load at fracture (N) and d is the mean of the original diameter of the specimen (mm). The elastic modulus ( E ) in GPa of each cylindrical specimen was also determined by calculating the slope of the initial linear portion of the stress–strain plot generated for each individual cylindrical GI specimen tested in compression prior to fracture .
2.6
Statistical analyses
Statistical analyses (three, two and one-way analyses of variance (ANOVAs) and Tukey’s post hoc analyses where required), as well as regression analyses were made in software (SPSS 12.0.1; SPSS Inc., Chicago, IL, USA) at a significance value of p = 0.05. The twelve control groups were analyzed using three (one each for the η , σ and E data) two-way ANOVAs where the factors were the PAA M w (P15, P26, P40 and P81) and PAA concentration (25, 35 and 45%). To determine trends in the η , σ and E data with increasing PAA M w and PAA concentration regression analyses were conducted individually for the pooled η , σ and E data. One regression analysis each for the η , σ and E data with increasing PAA M w and one regression analysis each for the η , σ and E data with increasing PAA concentration were made.
To analyze the η data for the 90 groups, a three-way ANOVA with factors of M w mixture (Groups A–F), blend ratio (10:90, 30:70, 50:50, 70:30 and 90:10) and PAA concentration (25, 35 and 45%) was performed. The η data for the M w mixtures and blend ratio groups was pooled and a regression analysis conducted to check if there was a significant effect of increasing PAA concentration. A two-way ANOVA ( M w mixture × blend ratio) was made for the η data and the analysis was reduced further to 15 one-way ANOVAs (one for each M w mixture ( n = 6) and one for each blend ratio ( n = 9)). Six regression analyses were made (one for each individual M w mixture) to check for trends within the η data as the blend ratio was increased from 10:90 to 90:10. In addition, the η data for the blend ratio groups was pooled and a regression analysis was performed to determine if a significant trend was evident with increasing M w .
Additionally, the 162 groups for the σ and E data were analyzed individually using a three-way ANOVA ( M w mixture × blend ratio (10:90, 20:80, 30:70, 40:60, 50:50, 60:40, 70:30, 80:20 and 90:10) × PAA concentration). The σ and E data for the M w mixtures and blend ratio groups was pooled independently and regression analyses were conducted to check if there was a significant effect of increasing PAA concentration on σ and E . Two-way ANOVAs ( M w mixture × blend ratio) were conducted for both the σ and E data. The analyses were reduced to 30 one-way ANOVAs (15 each for σ and E : one for each M w mixture ( n = 6) and one for each blend ratio ( n = 9)). Twelve regression analyses were made for each individual M w mixture to check for trends within the σ and E data as blend ratio was increased from 10:90 to 90:10. In addition, the blend ratio data was pooled for the σ and E data sets and two regression analyses were performed, respectively to determine if significant trends were evident with increasing M w .
2
Materials and methods
2.1
Materials
The powder constituent of a commercial hand-mixed GI restorative (Ionofil Molar; Voco GmbH, Cuxhaven, Germany; Lot No. 0947058, shade A3) was used to prepare all specimens in the current study. The four control PAAs were supplied as powders and were reported by the manufacturer to have M w s of 15,000 (P15), 25,700 (P26), 40,000 (P40) and 80,800 (P81) ( Table 1 ). The M w and number average molecular weight ( M n ) of the four control PAAs were previously quantified using gel permeation chromatography and the PDis were calculated by dividing M w by M n ( Table 1 ).
PAA | Manufacturer | M w | M n | PDi |
---|---|---|---|---|
P15 | Advanced Healthcare Limited, Tonbridge, England | 15,250 (1567) | 8680 (691) | 1.76 |
P26 | Robert Hill, Queen Mary, University of London, England | 25,910 (1863) | 13,680 (1550) | 1.89 |
P40 | Advanced Healthcare Limited, Tonbridge, England | 40,156 (2717) | 21,930 (2422) | 1.83 |
P81 | Robert Hill, Queen Mary, University of London, England. | 80,650 (5400) | 41,840 (3069) | 1.93 |
2.2
Polyacrylic acid solution preparation
Twenty-four hours prior to usage, the four control PAAs (P15, P26, P40 and P81) were prepared in solution at concentrations of 25, 35 and 45% by dispensing the PAA powder (0.5, 0.7 or 0.9 g, respectively) into 2 mL of distilled water at room temperature. Additionally, the six powder mixtures of the four control PAAs (Group A: P15 and P26; Group B: P15 and P40; Group C: P15 and P81; Group D: P26 and P40; Group E: P26 and P81 and Group F: P40 and P81) were dry blended at powder:powder ratios of 10:90, 20:80, 30:70, 40:60, 50:50, 60:40, 70:30, 80:20 and 90:10. Solutions of the M w mixtures (Groups A–F) at the dry blend ratios investigated were also prepared, 24 h before use, in accordance with the procedure for the control PAA solutions prepared at concentrations of 25, 35 and 45%.
2.3
Viscosity determination
A digital viscometer (Brookfield DV-E Viscometer; Brookfield Engineering Laboratories Inc., Middleboro, MA, USA) containing a small sample adaptor was employed to measure the viscosity ( η ) of the four control PAA solutions prepared at concentrations of 25, 35 and 45%. Additionally, the η of the six M w mixtures (Groups A–F), dry blended at five powder:powder ratios (10:90, 30:70, 50:50, 70:30, and 90:10) prepared at three PAA concentrations (25, 35 and 45%) were also determined. The inner chamber of the small sample adaptor was filled with 2.1 mL of a PAA liquid solution and a spindle was inserted carefully to avoid trapping air bubbles in the PAA solution. The viscometer rotated the spindle at 100 rpm in the PAA solution and the η in mPa s was recorded. The η for each of the 102 groups investigated (six M w mixtures × five blend ratios × three PAA concentrations ( n = 90) and four control PAAs × three PAA concentrations ( n = 12)) were determined on three occasions and the mean η was recorded.
2.4
GI restorative preparation
A powder to liquid mixing ratio of 4:1 (g:g) was used for each GI restorative group under investigation in accordance with manufacturers’ recommendations for Ionofil Molar. To prepare the GI, a glass slab was placed on a balance (Sartorius Expert; Sartorius AG, Goettingen, Germany) and 0.188 g of the PAA liquid solution was dispensed onto one end of the glass slab using an adjustable pipette (Gilson, Middleton, WI, USA). Ionofil Molar powder (0.75 g) was weighed onto the opposite end of the glass slab and separated into two parts. The first part of the powder was hand-mixed with the PAA liquid solution for 20 s using a stainless steel spatula. The remaining powder was added and hand-mixed so that the total mixing time was 40 s.
2.5
Compression testing protocol
To determine the compressive fracture strength, cylindrical specimens (6.0 ± 0.1 mm height and 4.0 ± 0.1 mm diameter) were prepared using a polytetraflouroethylene (PTFE) split-mold assembly . A PTFE base plate was covered with an acetate strip and the PTFE split-mold was placed on top and aligned using a 4 mm diameter holding pin. Two nylon sliding wedges were fitted to one side of the mold to ensure equal pressure was applied along the length of the split-mold . To minimize the incorporation of air bubbles into the specimens, the largest convenient portion of the GI was conveyed to one side of an individual hole in the split-mold and allowed to flow into the mold . A second acetate strip was placed on top of the filled split-mold assembly and a glass slab was applied to isolate the specimens from the surrounding atmosphere. The mold assembly was clamped using G-clamps to apply equal pressure to all specimens and ensure parallelism of the cylindrical specimen ends for uniform contact with the compressive platens of the testing apparatus . The specimens were maintained in a water-bath maintained at 37 ± 1 °C for 1 h. The mold was disassembled, the specimens removed and specimens which contained visible defects such as chipped edges, non-parallel ends or pores were discarded. The remaining specimens were stored in glass containers filled with 50 mL of distilled water in an incubator (Climate Cabinet; Firlabo, Meyzieu, France) at 37 ± 1 °C for a further 23 h. Twenty nominally identical cylindrical GI restorative specimens were manufactured for each of the 174 experimental groups under investigation (six M w mixtures × nine blend ratios (10:90, 20:80, 30:70, 40:60, 50:50, 60:40, 70:30, 80:20 and 90:10) × three PAA concentrations ( n = 162) and four control PAAs × three PAA concentrations ( n = 12)).
Prior to compressive testing, the mean diameter of each cylindrical specimen was determined from three measurements taken using a digital micrometer reading to 1 μm (Mitutoyo, Kawasaki, Japan). To mimic the oral environment, the specimens were tested ‘wet’ by placing a piece of wet filter paper (Whatman No. 1; Whatman PLC, Kent, England) on the flat ends of each cylindrical specimen in accordance with ISO 9917-1:2003 . A compressive load was applied to the long axis of the cylindrical specimen using a universal testing machine (Instron Model 5565, High Wycombe, England) operating at a cross-head speed of 1 mm/min and the maximum load at failure was recorded.
The compressive fracture strength ( σ ) in MPa of each cylindrical specimen was determined by dividing the fracture force by the original cross-sectional area
σ = 4 F f π d 2
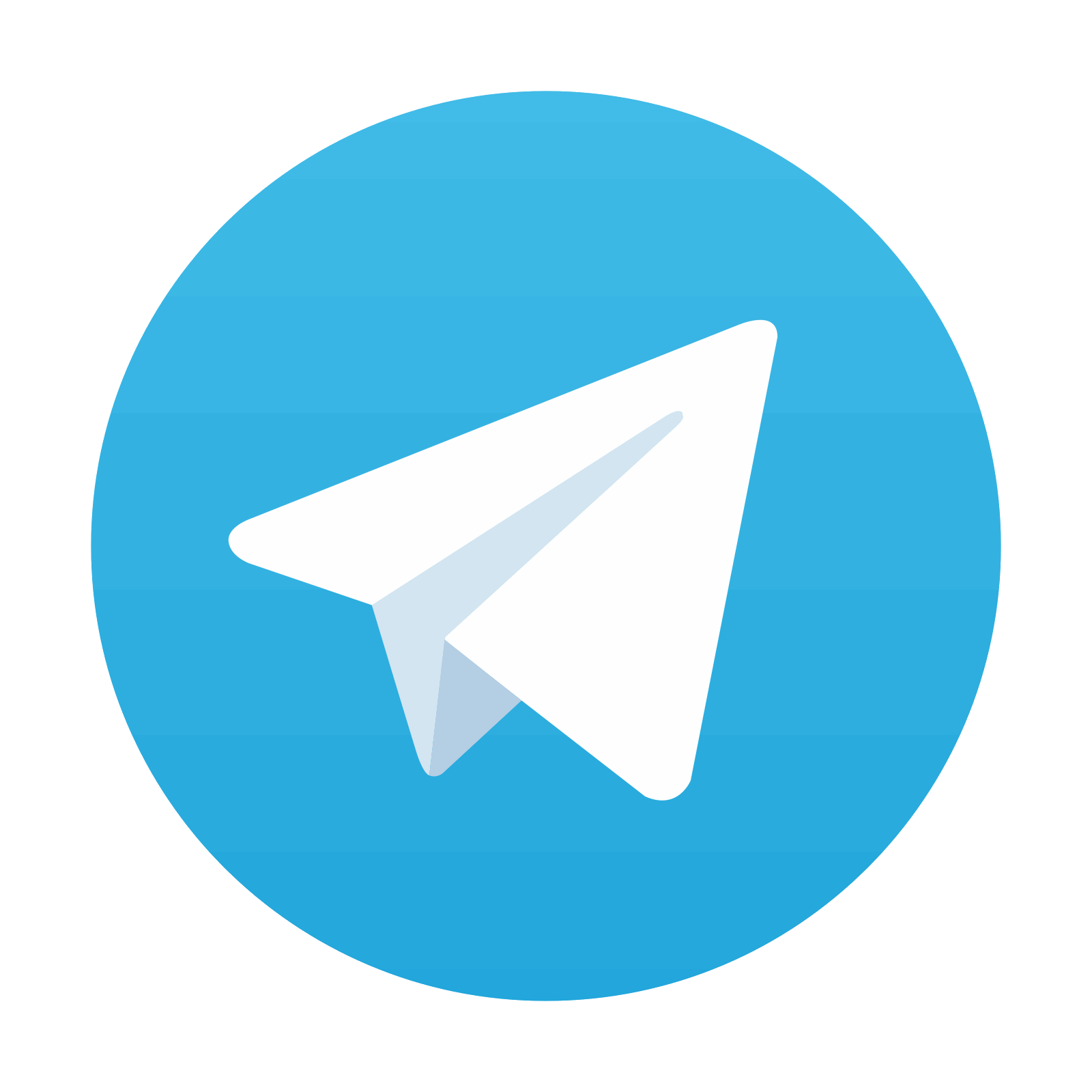
Stay updated, free dental videos. Join our Telegram channel

VIDEdental - Online dental courses
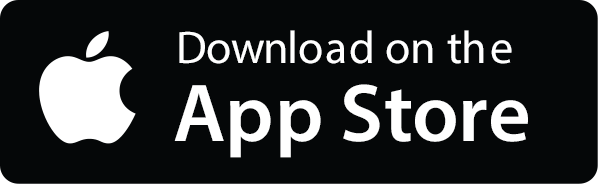
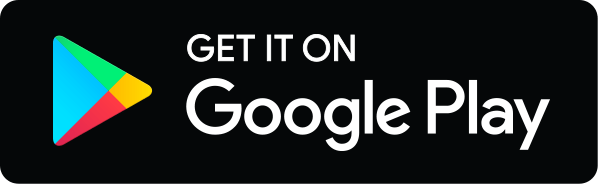