Abstract
Objective
To introduce and to examine the research progress and the investigation on hydraulic calcium silicate cements (HCSCs), well-known as MTA (mineral trioxide aggregate).
Methods
This review paper introduces the most important investigations of the last 20 years and analyze their impact on HCSCs use in clinical application.
Results
HCSCs were developed more than 20 years ago. Their composition is largely based on Portland cement components (di- and tri-calcium silicate, Al- and Fe-silicate). They have important properties such as the ability to set and to seal in moist and blood-contaminated environments, biocompatibility, adequate mechanical properties, etc. Their principal limitations are long setting time, low radiopacity and difficult handling.
New HCSCs-based materials containing additional components (setting modulators, radiopacifying agents, drugs, etc. ) have since been introduced and have received a considerable attention from laboratory researchers for their biological and translational characteristics and from clinicians for their innovative properties.
HCSCs upregulate the differentiation of osteoblast, fibroblasts, cementoblasts, odontoblasts, pulp cells and many stem cells. They can induce the chemical formation of a calcium phosphate/apatite coating when immersed in biological fluids.
These properties have led to a growing series of innovative clinical applications such as root-end filling, pulp capping and scaffolds for pulp regeneration, root canal sealer, etc.
The capacity of HCSCs to promote calcium-phosphate deposit suggests their use for dentin remineralization and tissue regeneration. Several in vitro studies, animal tests and clinical studies confirmed their ability to nucleate apatite and remineralize and to induce the formation of (new) mineralized tissues.
Significance
HCSCs play a critical role in developing a new approach for pulp and bone regeneration, dentin remineralization, and bone/cementum tissue healing. Investigations of the next generation HCSCs for “Regenerative Dentistry” will guide their clinical evolution.
1
Introduction
Interestingly, Portland cement appeared in dentistry before 1878 . MTA (mineral trioxide aggregate), a Portland cement-based formulation was developed more than 20 years ago as a root-end filling material but its potential for new clinical applications later became evident thanks to its innovative hydraulic properties and sealing ability. We have now more information on its properties and many new MTA-derived materials have been developed.
This paper uses the term hydraulic calcium silicate cement (HCSC) to refer to the entire family of MTA-like cements. A long list of new materials based on the original formulation and/or with minor modifications has been introduced in routine clinical practice ( Table 1 ). A light-curable HCSC is advisable in many clinical cases and at least one material is now on the market. Other experimental materials have been proposed, so many potential new products could soon be available and more very innovative HCSCs can be expected in the future.
Materials | Uses | Manufacturer | Composition |
---|---|---|---|
Biodentine | PC, Ag, REA, RP | Septodont, Saint-Maur-des-Fossés, France | Powder: tri-calcium silicate |
Liquid: aqueous calcium chloride solution and excipients | |||
Harward MTA Caps | PC, Ag, REA, RP | Harvard Dental International GmbH, Hoppegarten, Germany | Powder: mineral trioxide aggregate and bismuth oxide |
Ledermix MTA | PC, Ag, REA, RP, Af | Riemser, Riems, Germany | Powder: various mineral oxides |
Liquid: water | |||
MM MTA | PC, Ag, REA, RP, Af | Micromega, Besancon, France | Powder: tri-calcium and di-calcium silicate, calcium carbonate |
Liquid: H 2 O | |||
MTA Angelus | PC, Ag, REA, RP, Af | Angelus dental solutions, Londrina, PR, Brazil | Powder: SiO 2 , K 2 O, Al 2 O 3 , Na 2 O, SO 3 , CaO, Bi 2 O 3 , MgO, insoluble CaO, KSO 4 , NaSO 4 , crystallized silica |
Liquid: H 2 O | |||
MTA Plus | PC, Ag, REA, RP, A, RCS | Prevest Detpro Limited, Jammu, India | Powder: tri-calcium and di-calcium silicate |
Liquid 1: H 2 O | |||
Liquid 2: gel | |||
ProRoot MTA | PC, Ag, REA, RP, Af | Dentsply Tulsa, Johnson City, TN, USA | Powder: white Portland and bismuth oxide |
Liquid: H 2 O | |||
Tech Biosealer capping | PC, Ag | Isasan srl, Rovello Porro, Co, Italy | Powder: mixture of white CEM, calcium sulfate, calcium chloride, montmorillonite |
Liquid: DPBS (Dulbecco’s Phosphate Buffered Saline) | |||
TheraCal | PC, Ag | Bisco Inc, Schaumburg, IL, USA | Paste: 45% wt mineral material (type III Portland cement), 10% wt radiopaque component, 5% wt hydrophilic thickening agent (fumed silica), 45% methacrylic resin |
BioAggregate | RP | Innovative BioCeramix Inc, Vancouver, Canada | Powder: aluminum-free calcium silicate, monocalcium phosphate, tantalum oxide |
Liquid: deionized water | |||
Endo Sequence Bioceramic | REA, RP | Brasseler, Savanah, GA, USA | Powder: calcium silicate, calcium phosphate monobasic, calcium hydroxide, zirconium oxide, tantalum oxide, filler and thickening agents |
Liquid: H 2 O | |||
Retro MTA | REA, RP | BioMTA, Seoul, Republic of Korea | Powder: Tricalcium silicate, dicalcium silicate, tricalcium aluminate, tetracalcium aluminoferrite, free calcium oxide, bismuth oxide |
Liquid: deionized water | |||
Tech Biosealer root end | REA, RP | Isasan srl, Rovello Porro, Co, Italy | Powder: mixture of white CEM, calcium sulfate, calcium chloride, bismuth oxide, montmorillonite |
Liquid: DPBS (Dulbecco’s Phosphate Buffered Saline) | |||
iRoot SP, iRoot BP and iRoot BP Plus | RCS, Af | Innovative BioCeramix Inc, Vancouver, Canada | Paste: aluminum-free calcium silicate, calcium phosphate, calcium hydroxide, niobium oxide, zirconium oxide |
MTA Fillapex | RCS, Af | Angelus dental solutions, Londrina, PR, Brazil | Paste-Paste: Salicylate resin, diluting resin, natural resin, bismuth trioxide, nanoparticulated silica, MTA, pigments |
Ortho MTA | RCS, Af | BioMTA, Seoul, Republic of Korea | Powder: Tricalcium silicate, dicalcium silicate, tricalcium aluminate, tetracalcium aluminoferrite, free calcium oxide, bismuth oxide |
Liquid: deionized water | |||
Tech Biosealer endo | RP, RCS, Af | Isasan srl, Rovello Porro, Co, Italy | Powder: mixture of white CEM, calcium sulfate, bismuth oxide, montmorillonite, sodium fluoride |
Liquid: DPBS (Dulbecco’s Phosphate Buffered Saline) |
Despite of the dearth of information from the first in vitro experiments and the lack of clinical studies, HCSC gained the trust of many operators who proposed its clinical use for apicogenesis, pulp capping and other procedure. Tay and Pashley introduced the concept of “biomimetic remineralization” of partially demineralized dentin using MTA. This represented a major innovation and opened the way to potential new applications for HCSCs-based materials. At the moment the use of HCSCs-based technology for dentin remineralization is not far from the clinical application.
The history of calcium silicate Portland cements dates back to Roman times when a cement able to set in water made by grinding together lime and a volcanic product found at Puteoli (hence called pozzolana ) around Neapolis (the places described by Pliny the Elder). Pozzolana helped Roman concrete set quickly even when submerged in water, allowing the construction of bridges, harbors, aqueducts, monuments and buildings. During the middle ages the secret of cement was lost. In the 18th century John Smeaton, an English engineer, rediscovered the correct proportions of cement using clay and limestone. In 1824, Joseph Aspdin, an English bricklayer, patented a process for making what he called Portland cement. The first great bridge built in the USA in the late 19th century was made of Portland–Pozzolanic cements. Modern Portland cement is made by mixing substances containing lime, silica, alumina, and iron oxide and then heating the mixture until it almost fuses. Pozzolana is still the main component of many Portland cements.
Calcium silicate Portland cements set through a hydration reaction after mixing with water or water-containing liquids. Various hydration products form during the reaction, namely different phases of calcium silicate hydrate (CSH) as porous colloidal CSH gel and radial acicular CSH crystals, rombohedral crystals of portlandite (calcium hydroxide), needle-like crystals of ettringite (hexacalcium aluminate trisulphate hydrate) ( Fig. 1 ), and calcium monosulfoaluminate or calcium monocarboaluminate. Porous CSH hardens by the formation of a solid network within 1–6 h. The setting reaction requires several days to complete the hydration and hardening phases and includes dissolution and reprecipitation processes of the unhydrated di- and tri-calcium silicate phases (C 2 S and C 3 S) and formation of hydration products like calcium di- and tri-silicate hydrates and calcium hydroxide . In this phase, CSH has layer structure, with a layer growing radially from the calcium silicate particles and resulting in a fibrous needle-like complex structure, and cuboidal calcium hydroxide crystals form among the hydrating cement compounds ( Fig. 2 ). This phase is difficult to inspect under standard SEM. As demonstrated by Gandolfi et al. , environmental SEM (ESEM) analysis can provide more information on the morphology of the early stage of Portland and dental calcium silicate/MTA cements ( Fig. 3 a and b ). Original native Pozzolanic cements are very similar to HCSCs under ESEM ( Fig. 4 ). Moisture ( i.e. biological fluids) is essential to allow the setting reaction and to develop/activate the cement’s bioactivity, such as the formation of apatite . The following stages have been proposed by Gandolfi et al. for the nucleation of calcium phosphates on the HCSC surface:
- 1.
A solid–liquid interface forms on the mineral particles, and ion dissolution occurs almost immediately. Ca 2+ ions rapidly migrate into the mixing solution and portlandite (Ca(OH) 2 ) forms.
- 2.
Silicates are attacked by OH ion groups in an alkaline environment and a CSH phase forms on mineral particles. CSH is a porous, fine-grained/fibrous and disorganized hydrated silicate gel layer containing Si-OH silanol groups and negative surface charges that may act as nucleation sites for apatite formation.
- 3.
CSH contains an excess of calcium hydroxide/portlandite formed by OH − ions from dissociated water molecules and Ca 2+ ions from the cement particles, so a strong continuous outflux of calcium hydroxide from CSH occurs during the first hours after mixing causing a marked rise in the pH and an increase in calcium ion concentration in the surrounding environment. Portlandite crystals nucleate inside the hydrating wet paste and are also detectable on the surface by ESEM/EDX. The release of portlandite persists for a long time in immersion/storage conditions, as revealed by the high pH of the soaking medium which remains constant at 11.0–12.0, depending on the chemistry of the material.
- 4.
When exposed to a phosphate-containing fluid – simulated body fluids (SBFs) or body fluids – a sequence of reactions take place on the surface of HCSCs between calcium from the cement and phosphate from the solution, namely the absorption of Ca and P ions on the silanol groups (Si-OH) of the silica-rich CSH surface and the precipitation of calcium phosphates and apatite (as a result of local supersaturation) which matures into a B-type HCA phase at increasing storage times. The calcium phosphate apatite deposits form a layer of spherulites filling the superficial porosities ( Fig. 3 b) . EDX, FTIR and micro Raman analyses have been used to demonstrate the formation of calcium phosphate and apatite deposits on cements after soaking in different SBFs ( Figs. 5 and 6A, B ) .
Fig. 5 Kinetics of apatite formation in SBF of a HCSC Portland-based cement monitored over time by SEM and EDX analyses. Fresh cement mainly displayed Ca, Si, S, Cl and Al peaks (all components of the cement). After 7-day soaking in SBF the surface became covered by aggregated spherulites. During the soaking time the coating increased in thickness and continuity and consequently the cement surface shifted from a calcium silicate to a calcium phosphate composition. With long (28 days) soaking times, Si completely disappeared, while P resulted in a growing peak.Fig. 6 (A) Raman analyses of different freshly mixed calcium silicate cements . (B) Raman analyses of a conventional hydraulic calcium silicate cement after soaking in SBF. The apatite peaks appearing during soaking in SBF narrow and increase in intensity with aging .




2
Properties
HCSCs are evolving rapidly as they may be used for a number of clinical applications where previous materials failed. They can set in wet environments such as in the presence of water, blood, dentinal fluid, saliva, etc. , and have satisfactory/good biological properties. This is the principal reason they have gained great attention for clinical many applications.
Historically, gray ProRoot MTA was the only HCSC on the market for a long time, subsequently flanked by white ProRoot MTA. Many of the early studies were done on these two materials and they still represent the gold standard to compare newer cements. New HCSCs have been developed and marketed while many experimental materials/prototypes have been proposed to investigate their effectiveness and to pass/obviate some of the drawbacks of the original cements such as: long setting time, difficult handling and manipulation, risk of wash-out in a too moist environment ( i.e. bleeding sites), low radiopacity, and high cost. Table 1 is schematic list of HCSCs present on the market (marketed in some countries under different brand names) and their clinical uses.
2.1
Expansion and sealing ability
Interestingly, HCSCs expand by 0.2–6% of the initial volume. Water sorption induces some expansion and makes a strong contribution to the sealing capacity. Proteins (for example from soaking medium or from in vivo body fluids) reduce the expansion of HCSC materials and increase the setting time .
Some preliminary microleakage tests with dye solutions have been performed on HCSCs but the more accurate fluid filtration and micro-CT tests should receive more attention. Fluid filtration tests offer a dynamic idea of the sealing ability of gutta-percha/sealer obturation from coronal to root apex , while micro-CT gives a 3D quantitative evaluation of the gaps and porosities at the dentin/sealer/gutta-percha interface and inside the filling materials . Circumferential-spiral channels related to the 3D porosity distribution in the dentin/sealer/gutta-percha system have been described for HCSCs used as root canal sealers with the Thermafil system . That study demonstrated a more complex 3D architecture of the sealer around the plastic carrier and inside the root canal . Evaluation of the volume of open pores and impervious pores may be an indirect index of the risk of large voids affecting the internal morphology of the sealer .
Polymicrobial tests are further methods of detecting the sealing and antibacterial properties of a root canal sealer. Several studies demonstrated the antibacterial properties of HCSCs, namely against Enterococcus faecalis . This is probably related to the cement’s active calcium hydroxide release and diffusion across dentin, and alkalinizing activity .
2.2
Compressive strength
Compressive strength is correlated with the stage of hydration and the condensation pressure . The use of condensed dry powder unmixed with water may produce a valid seal in root-end filling . Acid etch procedures, usually performed before bonding system application, may modify the strength of some cements as seen from the significant decrease of white and gray MTA when exposed to an environment of pH 5.0 .
2.3
Push-out test
Push-out tests have been carried out to obtain more information on HCSCs mechanical properties and their relation to sealing ability. HCSCs tend to absorb water and expand thereby enhancing their push-out strength . HCSCs expansion increases microchemical cement retention along the internal walls and interface adaptation . Biomineralization can positively influence the push-out bond strength of HCSCs by increasing the mechanical retention , whereas removal of smear layer from root canal walls may undermine bond strength between HCSC and dentin .
2.4
Radiopacity
The radiopacity of Portland cements makes them unsuitable for clinical use. For this reason a number of radiopacifiers have been included in the composition of HCSCs. ProRoot MTA contains approx. 20% bismuth oxide, similar to MTA Angelus, Tech Biosealer root-end and Tech Biosealer endo and MM-MTA. Other cements contain different components like zirconium oxide, barium sulphate and calcium tungstate to improve their radiopacity . Unfortunately, bismuth oxide may reduce/impair the cement’s biocompatibility, as demonstrated in vitro by Gandolfi et al. . For these reasons, some commercial HCSCs are produced free from radiopacifier if dedicated to specific clinical applications such as pulp capping or apicogenesis.
2.5
Setting time
HCSCs are hydraulic materials, so they can set in the presence of water in approx. 40–120 min ( Table 2 ). The initial setting time is usually around 40–50 min, while final setting time is around 120–170 min and approx.75–80 min for HCSCs doped with various calcium phosphates . Recent materials such as Biodentine, MTA Plus and light-curable TheraCal showed notably shorter setting times : 9 min for Biodentine, 55 min for MTA Plus with gel and Tech Biosealer capping, 80 min for MTA Angelus, 130 min for MTA Plus and 250 min for ProRoot MTA .
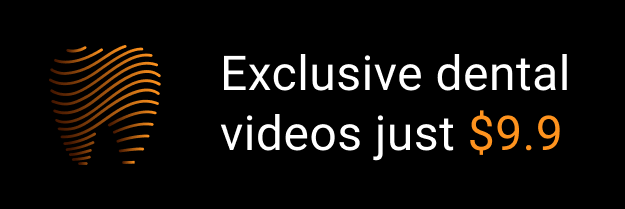