Introduction
BoneCeramic (Straumann, Basel, Switzerland) can regenerate bone in alveolar defects after tooth extraction, but it is unknown whether it is feasible to move a tooth through BoneCeramic grafting sites. The objective of this study was to investigate 3-dimensional real-time root resorption and bone responses in grafted sites during orthodontic tooth movement.
Methods
Sixty 5-week-old rats were randomly assigned to 3 groups to receive BoneCeramic, natural bovine cancellous bone particles (Bio-Oss; Geistlich Pharma, Wolhusen, Switzerland), or no graft, after the extraction of the maxillary left first molar. After 4 weeks, the maxillary left second molar was moved into the extraction site for 28 days. Dynamic bone microstructures and root resorption were evaluated using in-vivo microcomputed tomography. Stress distribution and corresponding tissue responses were examined by the finite element method and histology. Mixed model analysis of variance was performed to compare the differences among time points with Bonferroni post-hoc tests at the significance level of P <0.05.
Results
The BoneCeramic group had the least amount of tooth movement and root resorption volumes and craters, and the highest bone volume fraction, trabecular number, and mean trabecular thickness, followed by the Bio-Oss and the control groups. The highest stress accumulated in the cervical region of the mesial roots.
Conclusions
BoneCeramic has better osteoconductive potential and induces less root resorption compared with Bio-Oss grafting and naturally recovered extraction sites.
Highlights
- •
We studied periodontal tissue changes of moving teeth into BoneCeramic grafted alveolar defects.
- •
We evaluated dynamic bone microstructures and root resorption with micro-CT in rats.
- •
Orthodontic loading affects bone microstructure changes and root resorption during tooth movement.
- •
BoneCeramic has better osteoconductive potential and causes less root resorption than Bio-Oss.
Moving a tooth orthodontically into the region of an alveolar defect with insufficient buccolingual width can be problematic. It often causes root resorption, gingival recessions, periodontal pockets, and traumatic injuries to pulp vitality. Alveolar defects may appear in patients with cleft lip and palate. If the cleft involves the alveolus, the alveolar ridge defects in these sites impede tooth eruption and delay orthodontic tooth movement with prolonged treatment times. Alveolar defects may also be secondary to a large piece of buccal plate with the extraction of an impacted tooth, jaw inflammation, or cyst and trauma surgery. Similar scenarios can be seen during extensive remodeling of the edentulous alveolus after the extraction of damaged or periodontal-involved first molars. For some adolescent and young adults, once periodontal disease is under control, an effective way to replace a missing molar is to move the adjacent molar into the first molar area. However, alveolar bone in extraction sites quickly resorbs, with reduced height and width, primarily changing to dense cortical bone, which impedes the movement of teeth through the bone. Orthodontic tooth movement is not feasible without reestablishment of the alveolar width with bone grafts before movement.
To alleviate alveolar ridge resorption and regenerate alveolar bone in the defects, bone grafts, such as autogenous bone, allograft, or alloplast, can be placed in the extraction sites immediately after tooth extraction. Although autogenous bone is recognized as the gold standard for bone grafts because of the viability of transferable osteogenic cells, it requires a second surgery from the donor site. Patients tend to decline this treatment modality because of the additional discomfort. Alternatively, deproteinized bovine bone mineral (Bio-Oss; Geistlich Pharma, Wolhusen, Switzerland) can be used as a graft material for augmenting an alveolar ridge defect. However, Bio-Oss degrades slowly and induces uncertain immune responses and fibrous encapsulation with healing.
A new synthetic bone substitute, BoneCeramic (Straumann, Basel, Switzerland), has been used for ridge preservation because it can be rapidly replaced by regenerative bone. BoneCeramic consists of 60% hydroxyapatite and 40% beta tricalcium phosphate. After grafting, beta tricalcium phosphate is rapidly resorbed and completely replaced by regenerative bone. Meanwhile, the hydroxyapatite, resorbed slowly, serves as a good matrix scaffold for ingrowth of new blood vessels and attachment of bone-forming cells. Bone can be greatly regenerated because BoneCeramic can be totally resorbed and subsequently replaced by host bone in a shorter time. However, whether it will hamper orthodontic tooth movement in an augmented area of alveolar ridge is still unknown.
To evaluate whether a tooth can be moved into an extraction site with bone grafts, one should investigate the rate or distance of orthodontic tooth movement with accompanied bone responses or adverse side effects. The aims of this experiment were (1) to evaluate whether it is feasible to move a tooth in an alveolar ridge augmented with BoneCeramic, and (2) to evaluate real-time 3-dimensional (3D) changes in root resorption and bone microarchitectures, and tissue responses when moving teeth into BoneCeramic and Bio-Oss grafts after extractions. Understanding the dynamic responses in bone graft resorption, bone regeneration, and root resorption will help to establish treatment guidelines for patients.
Material and methods
All procedures were approved by the institutional animal care and use committee of the School of Stomatology, Capital Medical University, Beijing, China. After they were acclimated, 60 male Sprague Dawley rats (age, 5 weeks) (specific pathogen free level 3) had the maxillary left first molar extracted under anesthesia by chloral hydrate (2 mL/kg) injected intraperitoneally. A rectangular (3 × 2 × 2 mm) alveolar ridge defect was created in the socket by completely resecting the intraradicular septa between the tooth roots at the extraction sites with a low-speed dental handpiece. The animals were randomly assigned to 3 groups (n = 20 per group) to receive BoneCeramic (60% hydroxyapatite, 40% beta tricalcium phosphate granules), Bio-Oss (natural bovine spongiosa granules, 0.25-1 mm), or no grafting. The mucosa was reflected and sutured to cover the socket to prevent the materials from leaking. Signs of infection, animal weight, and health conditions were monitored.
Four weeks after the extractions, a groove was prepared at the cervical third of the incisors under anesthesia. A nickel-titanium coil spring (0.2 mm in diameter; IMD, Shanghai, China) was ligated between the maxillary left second molar and incisors using an 0.08-in ligature wire secured with bonding adhesive (Transbond; 3M Unitek, Monrovia, Calif). The spring was activated for approximately 1 mm to produce 10 g of continuous force to move the molar forward into the extraction site ( Fig 1 , A ).
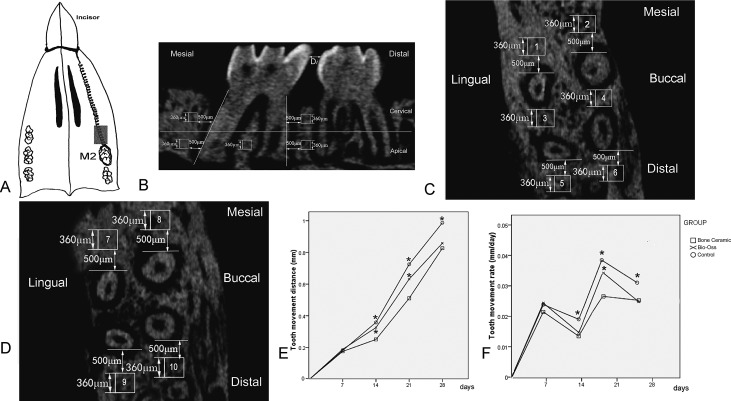
After spring installation, each animal was scanned using an in-vivo microcomputed tomography system (SkyScan1076; Bruker, Kontich, Belgium) on days 0, 7, 14, 21, and 28. The spring was removed for each in-vivo microcomputed scan and reattached after scanning. The palate was secured at the supine position parallel to the stage in a carbon composite animal holder. The head was scanned through 180° of rotation at a 0.5° increment with 2 seconds per degree with resolution of 18 μm per pixel. Data were further reconstructed to provide axial cross-section images with SkyScan NRecon software (version 1.4.4; Bruker) and converted into 16-bit bitmapped TIFF images with a resolution of 1024 × 1024 pixels.
Tooth movement distance (D i ) (mm) was measured 3 times and averaged at each time point (i = 0, 7, 14, 21, and 28 days). Tooth movement rate (V i ) was then calculated as Vi=(Di−Di−1)/7
V i = ( D i − D i − 1 ) / 7
( Fig 1 , B ).
The alveolar bone was equally divided into the cervical and apical regions by a selected middle axial slice from the root apex to the furcation. In the apical region, 6 regions of interest (360 μm 3 ) were selected: 500 μm mesial to the mesiobuccal and the mesiolingual roots, 500 μm distal to the distobuccal and the distolingual roots, and the intraradicular septa between the mesiobuccal and distobuccal roots and between the mesiolingual and distolingual roots ( Fig 1 , C ). In the cervical region, 4 regions of interest (360 μm 3 ) were selected: 500 μm mesial to the mesiobuccal and the mesiolingual roots, and distal to the distobuccal and the distolingual roots ( Fig 1 , D ).
Three-dimensional microarchitecture parameters for trabecular bone were measured as the following: bone volume fraction (BV/TV), indicating the ratio of bone volume to total volume; mean trabecular thickness (Tb.Th), indicating the local thickness at each voxel representing bone; trabecular number (Tb.N), indicating the average number of trabeculae per unit of length; and trabecular separation (Tb.Sp), indicating the mean distance between trabeculae.
After segmenting the roots from the images using Mimics software (version 17.0; Materialise, Leuven, Belgium), the cervical, apical, and total root volumes of each root were measured for each time point. The volumes of root resorption in each region on days 7, 14, 21, and 28 were calculated by subtracting the root volume at each time point from the root volume on day 0.
After 28 days, the animals were killed, and the maxillae were dissected, fixed, decalcified, and embedded in paraffin and serially sectioned (5 μm) along the mesiodistal axis of the second molar. The sections were stained with hematoxylin and eosin.
A 3D finite element method model of the maxillary second molar (day 0) was constructed with the Mimics software and imported into Ansys software (Ansys, Canonsburg, Pa). The geometry of the model consisted of 50019 isoparametric tetrahedral-node solid elements and 69731 nodes. The width of the periodontal ligament was set at 0.2 mm, and Young’s modulus of the tooth, periodontal ligament, and bone were set as 19,600, 0.7, and 13,700 MPa, respectively. The Poisson ratios of the tooth, periodontal ligament, and bone were 0.15, 0.49, and 0.15, respectively. A 10-g force was applied in the finite element method model in the same direction as the spring attached intraorally. Movement was suppressed in 6 degrees of freedom for the nodes on the bottom edge of the alveolar bone. Tooth displacement and stress distribution analyses were performed with the Ansys software.
Statistical analysis
For each parameter, mixed-model measures analysis of variance was performed to compare the differences among time points and between groups with Bonferroni post hoc tests using the Statistical Package for the Social Sciences (version 11.5; SPSS, Chicago, Ill). The significance level was set at P <0.05.
Results
The greatest amount of tooth movement was observed in the control group, followed by the Bio-Oss group and then the BoneCeramic group ( Fig 1 , E ). In all groups, tooth movement rates accelerated from days 0 to 7 and slightly decelerated from days 7 to 14. This similar tooth movement pattern repeated with acceleration from days 14 to 21, followed by deceleration from days 21 to 28 ( Fig 1 , F ).
In all regions of interest, the BV/TV, Tb.N, and Tb.Th significantly decreased from days 0 to 7 but increased from days 14 to 28, except for regions of interest 2 and 8 in all groups. Tb.Sp significantly decreased from days 0 to 7 but fluctuated from days 14 to 28 in all groups ( Fig 2 ). The typical changes of BV/TV, Tb.N, Tb.Th, and Tb.Sp in all regions of interest during tooth movement were found in regions of interest 1, 2, 7, 8, and 10 ( Tables I-III ; Fig 2 ). Regions of interest 3 to 6 and 9 are omitted because of their similar changes to regions of interest 1, 2, 7, 8, and 10.
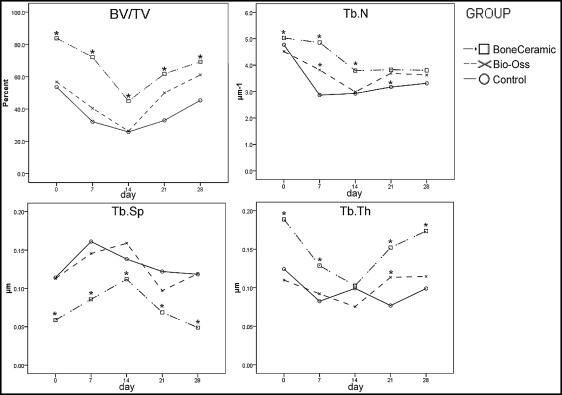
Day | Group | ROI 1 | ROI 2 | ||||||
---|---|---|---|---|---|---|---|---|---|
BV/TV | Tb.N | Tb.Sp | Tb.Th | BV/TV | Tb.N | Tb.Sp | Tb.Th | ||
0 | A | 82.98 (7.21) ∗ | 5.31 (0.12) ∗ | 0.06 (0.01) ∗ | 0.18 (0.02) ∗ | 80.82 (5.12) ∗ † | 5.22 (0.14) ∗ | 0.13 (0.01) ∗ | 0.18 (0.01) ∗ |
B | 58.48 (8.22) | 4.78 (0.22) | 0.10 (0.01) | 0.10 (0.01) | 48.41 (4.12) ∗ | 4.11 (0.10) | 0.14 (0.02) | 0.11 (0.09) | |
C | 57.38 (8.24) | 5.86 (0.13) | 0.08 (0.01) | 0.11 (0.01) | 23.78 (5.67) | 4.08 (0.09) | 0.08 (0.01) | 0.09 (0.01) | |
7 | A | 78.99 (5.21) ∗ | 4.96 (0.21) ∗ † | 0.09 (0.02) ∗ | 0.12 (0.02) ∗ | 57.57 (4.23) ∗ † | 4.25 (0.14) | 0.18 (0.02) ∗ | 0.12 (0.02) |
B | 45.24 (4.22) | 3.12 (0.14) ∗ | 0.12 (0.01) | 0.09 (0.01) | 42.47 (4.67) ∗ | 4.10 (0.09) | 0.14 (0.01) | 0.11 (0.02) | |
C | 35.08 (3.09) | 2.17 (0.11) | 0.16 (0.02) | 0.09 (0.01) | 39.43 (5.78) | 2.47 (0.13) ∗ | 0.12 (0.01) | 0.06 (0.01) ∗ | |
14 | A | 45.22 (3.56) ∗ | 4.76 (0.08) ∗ | 0.09 (0.01) ∗ | 0.09 (0.01) | 32.33 (6.79) | 4.69 (0.10) ∗ † | 0.11 (0.01) ∗ | 0.12 (0.02) |
B | 21.25 (4.63) | 3.01 (0.09) | 0.19 (0.02) | 0.06 (0.01) | 29.25 (2.54) | 3.52 (0.12) ∗ | 0.13 (0.02) | 0.06 (0.02) ∗ | |
C | 22.03 (5.21) | 3.12 (0.10) | 0.11 (0.02) | 0.09 (0.01) | 28.33 (2.57) | 2.50 (0.13) | 0.12 (0.01) | 0.11 (0.01) | |
21 | A | 68.90 (4.11) ∗ | 4.27 (0.11) | 0.07 (0.01) ∗ | 0.16 (0.02) ∗ † | 43.37 (4.12) | 5.10 (0.09) | 0.10 (0.01) ∗ | 0.12 (0.02) ∗ † |
B | 58.33 (3.56) | 4.28 (0.12) | 0.10 (0.01) | 0.11 (0.01) ∗ | 42.24 (4.67) | 4.29 (0.08) | 0.11 (0.01) | 0.10 (0.02) ∗ | |
C | 34.58 (3.09) | 3.21 (0.14) ∗ | 0.10 (0.01) | 0.06 (0.01) | 21.37 (3.29) ∗ | 2.85 (0.13) | 0.12 (0.01) | 0.0 (0.01) | |
28 | A | 69.06 (3.41) ∗ | 4.87 (0.09) | 0.05 (0.01) ∗ | 0.17 (0.02) ∗ | 42.46 (5.67) | 5.51 (0.12) ∗ † | 0.08 (0.01) ∗ | 0.11 (0.02) |
B | 66.70 (3.56) | 4.90 (0.12) | 0.13 (0.02) | 0.13 (0.02) | 43.33 (4.43) | 4.46 (0.08) ∗ | 0.09 (0.01) | 0.10 (0.01) | |
C | 43.73 (4.11) | 3.57 (0.15) | 0.13 (0.02) | 0.08 (0.01) | 23.40 (2.47) ∗ | 3.29 (0.13) | 0.12 (0.02) | 0.07 (0.01) ∗ |
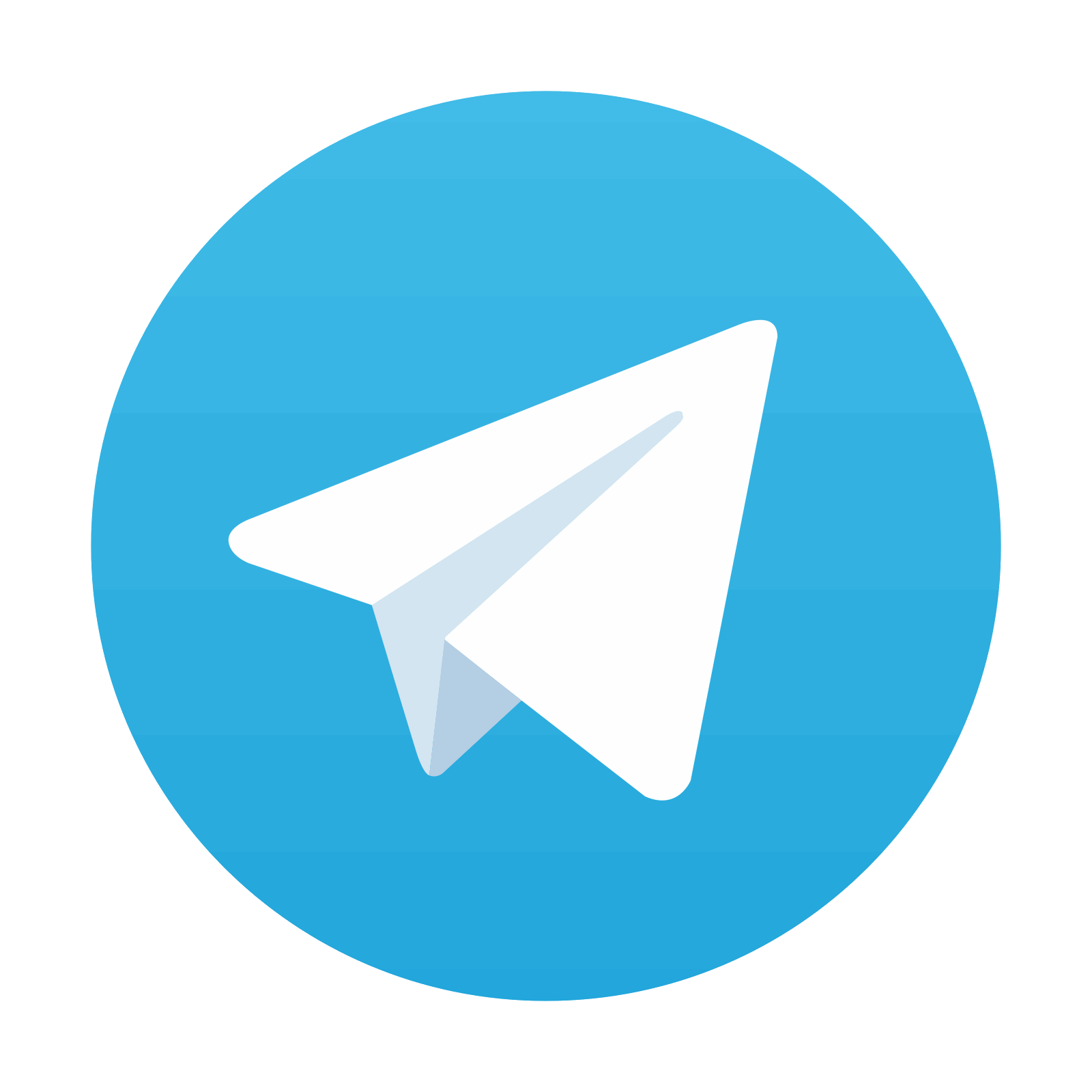
Stay updated, free dental videos. Join our Telegram channel

VIDEdental - Online dental courses
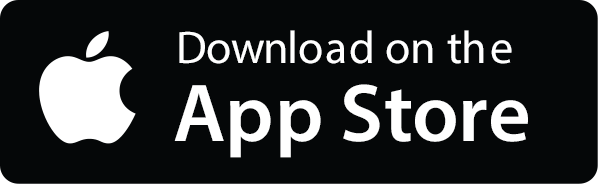
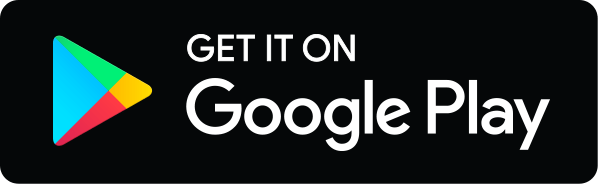
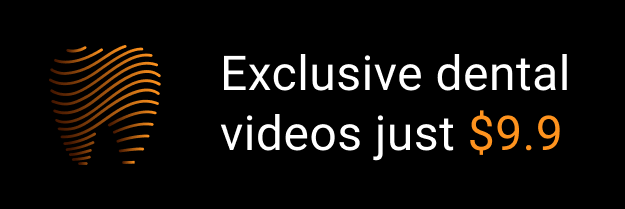