Introduction
The aims of this research were to use high-performance liquid chromatography to assess the bisphenol A (BPA) released from an orthodontic adhesive (Transbond XT; 3M Unitek, Monrovia, Calif) with various light-curing tip distances and to correlate the release to the degree of conversion.
Methods
One hundred thirty-eight premolar brackets were divided into 3 groups of 40 each for the high-performance liquid chromatography analysis and 3 groups of 6 each for assessing the degree of conversion. Fourier transform infrared spectroscopy was used for this purpose. Each group was studied at light-curing tip distances of 0, 5, and 10 mm. Statistical analyses were performed by using 2-way analysis of variance (ANOVA), post-hoc multiple comparisons Tukey HSD tests, and paired t tests. Pearson correlation was used to assess the correlation between the degree of conversion and BPA release.
Results
BPA release was greater in specimens cured with a greater light-curing tip distance. The degree of conversion decreased with increased light-curing tip distances. A negative correlation was found between BPA release and degree of conversion.
Conclusions
Clinicians should ensure that the adhesive is completely cured by keeping the light-curing tip as close to the adhesive as clinically possible.
Orthodontic bonding resins are exposed to oral fluids and are in contact with tissues throughout treatment. Leaching from resin can occur at 2 times: during the setting period of the resin and later when the resin is degraded. Leaching during the first process is related to the degree of conversion (DC). The DC of adhesives is of particular importance, since it modulates the physical and mechanical properties of the material, especially solubility and degradation.
Leaching results in the release of monomers such as bisphenol A (BPA). The implications of BPA released from dental biomaterials was first reported in a study that assessed dental sealants. BPA causes skin allergies, adverse effects on the reproductive systems of animals, cell death via necrosis, and high hemolytic activity. Terhune et al have suggested clinicians should be cautious in preventing extended contact of any of these materials with a patient’s skin, mucosa, and gingiva.
The depth of cure of resin-based composite decreases with increasing irradiation distance. The light-curing unit tip should ideally be in direct contact with the resin composite; however, this is not always clinically possible. Inability to place thelight tip near the bonding material might reduce intensity and provide a lower degree of polymerization.
Hence, the purposes of this study were to quantitatively assess the BPA released from the Transbond XT (3M Unitek, Monrovia, Calif) when varying the light-curing tip distances by 0, 5, and 10 mm with high performance liquid chromatography (HPLC) after accelerated chemical aging on days 1, 7, 21, and 35 and to evaluate the DC by using Fourier transform infrared (FTIR) spectroscopy on day 1. BPA release was also correlated to the DC.
Material and methods
A visible light-cured adhesive, Transbond XT, was used. From 138 premolar stainless steel brackets (3M Unitek), 120 were used to assess BPA release with HPLC, and 18 were used for estimating the DC with the FTIR spectrometer (Perkin-Elmer, Norwalk, Conn). BPA released and the DC values were assessed at 3 light-curing tip distances from the bracket: 0, 5, and 10 mm.
The varying distances of the curing tip were standardized by using a specially fabricated wooden box on which a yellow tile was placed. Sample preparation and the DC assessment with the FTIR spectrometer were similar to those described by Gioka et al. A 0.9-mm round metallic piece of wire with the predetermined distance from the bracket wing was used to confirm the curing distance. 0.9-mm diameter stainless steel wires 5 mm long (for the 5-mm group) and 10 mm long (for the 10-mm group) from the bracket wing were used to confirm the curing distance.
The brackets were pressed until they were seated on the tile. Excess resin was removed before polymerization. The yellow tile was covered by a cellulose film to facilitate detachment of the bracket-adhesive complex with recovery of the set material. Irradiation was performed from the labial surface of the bracket for 40 seconds with a halogen curing unit (3M ESPE, Seefeld, Germany).
We divided 120 brackets into 3 groups of 40 brackets each. Each group represented a light-curing tip distance of 0, 5, and 10 mm. The 40 brackets from each group were divided into 2 samples of 20 brackets each, representing the average number of brackets in a patient. These 20 brackets were immersed in a sterile glass tube containing 15 mL of absolute alcohol. Thus, each group consisted of 2 sterile glass tubes which in turn comprised 20 brackets per tube. The procedure used for assessing BPA release was similar to that described by Eliades et al. Briefly, the bonded brackets were stored in an incubator for 5 minutes at 37°C and 50% relative humidity, and immersed in sterile glass tubes containing 15 mL of absolute alcohol (99% v/v) to induce accelerated aging. During the immersion period, the solution was agitated for 10 seconds, twice a day. The processed 50 μL amounts of alcohol solution from each group were removed at days 1, 7, 21, and 35 and filtered through 0.2-μm nylon filter paper injected in the instrument and analyzed with the HPLC device (model LC2010A-HT, Shimadzu, Kyoto, Japan) The column was calibrated with known concentrations (standard, 0.1–3 ppm) of BPA (Merck, Darmstadt, Germany) in ethanol. The linear fittings of the calibration curves were used to calculate the concentration of monomers in the alcohol solution based on the area of chromatographic peaks at the corresponding retention time. The HPLC assays were performed in triplicate for each time period, and the results were averaged. Under the conditions of the experiment, the detection threshold of BPA was estimated at 0.1 ppm (0.1 μg/L).
The DC assessment was done by using FTIR. Eighteen brackets were divided into the 3 groups of 6 brackets each to represent the light-curing tip distances of 0, 5, and 10 mm. Resin polymerization was performed at room temperature. Immediately after polymerization, the cellulose strip was discarded, and the light-cured specimens were stored in light-proof boxes to prevent further exposure to light irradiation.
The light-cured specimens, which in clinical conditions corresponded to the material in contact with the enamel, were measured and prepared as 5-mg resin disks that were then placed between 0.01-mm potassium bromide disks. The disks were then transferred to the FTIR spectrometer. Processing the samples and recording their spectra occurred within 24 hours. The spectrum was also obtained for uncured adhesive by smearing the paste onto the potassium bromide disks. Spectra obtained for the adhesive from FTIR were recorded under the following conditions: 4000 to 400 cm −1 wave number range, 4 cm −1 resolution, and 16 scans co addition. The DC was estimated on a relative percentage basis with the 2-frequency method and tangent baseline technique. Aliphatic (C=C) bond-stretching vibrations, with a characteristic infrared absorption peak about 1638 cm −1 , were chosen as the analytical frequency, and the aromatic (C..C) bond-stretching vibrations, which do not interfere with the polymerization reactions about 1609 cm −1 , were selected as the reference frequency. By using the change in the ratio of the aliphatic C=C to the reference frequencies before and after curing, the DC was calculated with the formula:
RDB is the residual double bond that is calculated as
RDB = Ap ( C = C ) × Am ( C .. C ) Am ( C = C ) × Ap ( C .. C )
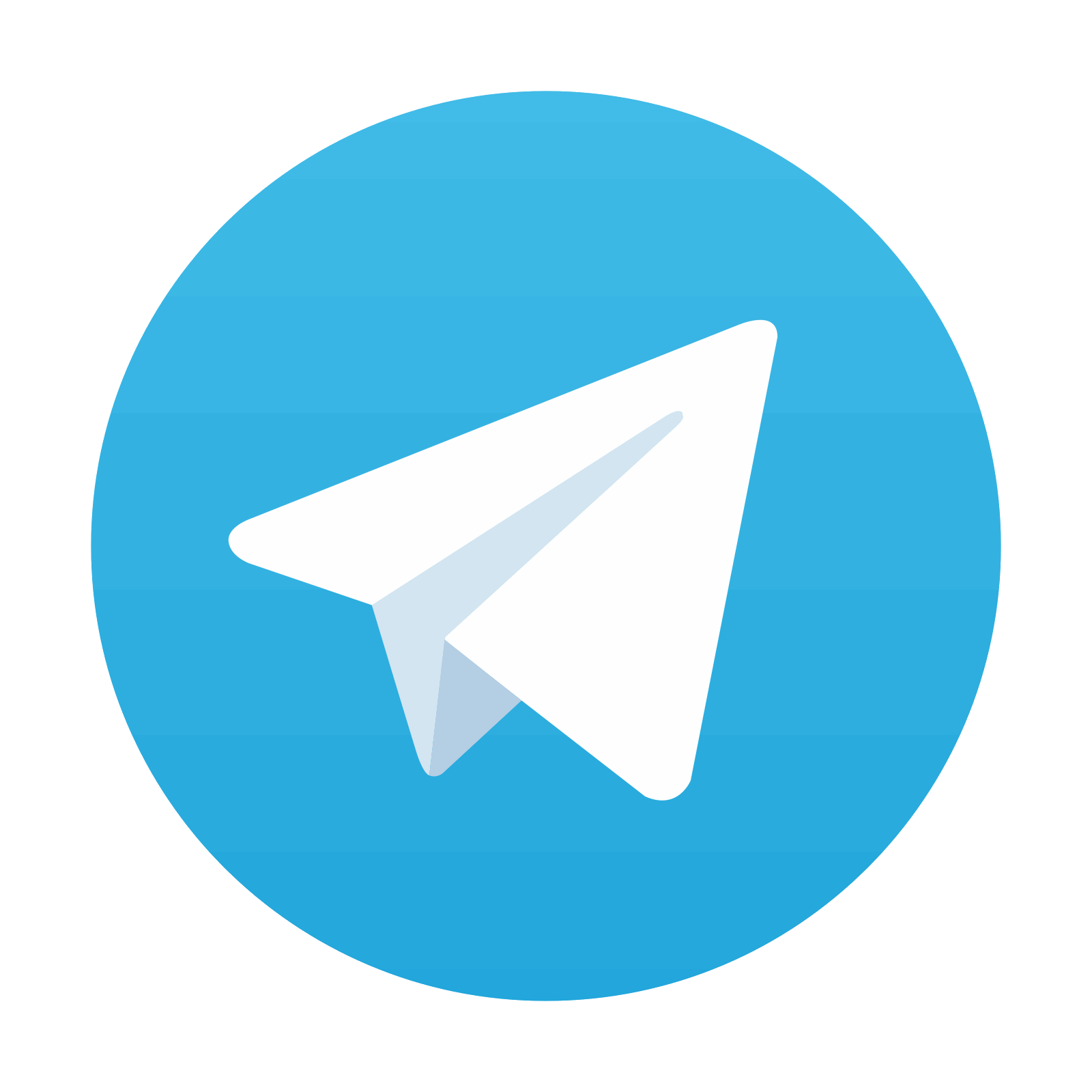
Stay updated, free dental videos. Join our Telegram channel

VIDEdental - Online dental courses
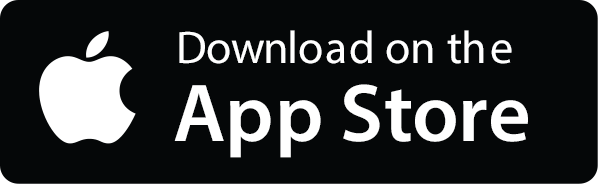
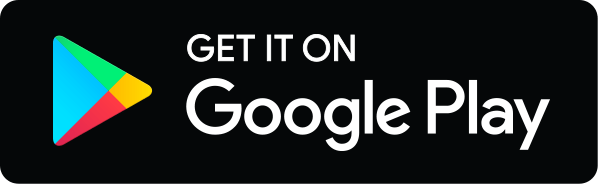