Graphical abstract

Highlights
- •
Material-dependent biomineralization achieved in vitro .
- •
Performance of gold standard acid-etched titanium confirmed in in vitro test system.
- •
Biomineralization leads to functional integration of dental implant material.
- •
Use of carbon coating increases biomineralization and osseointegration.
- •
Established in vitro test system mimics in vivo situation after implantation.
Abstract
Objectives
Before application in dental practice, novel dental materials are tested in vitro and in vivo to ensure safety and functionality. However, transferability between preclinical and clinical results is often limited. To increase the predictive power of preclinical testing, a biomimetic in vitro test system that mimics the wound niche after implantation was developed.
Methods
First, predetermined implant materials were treated with human blood plasma, M2 macrophages and bone marrow stromal stem cells. Thereby, the three-dimensional wound niche was simulated. Samples were cultured for 28 days, and subsequently analyzed for metabolic activity and biomineralization. Second test level involved a cell-infiltrated bone substitute material for an osseointegration assay to measure mechanical bonding between dental material and bone. Standard and novel dental materials validated the developed test approach.
Results
The developed test system for dental implant materials allowed quantification of biomineralization on implant surface and assessment of the functional stability of mineralized biomaterial-tissue interface. Human blood plasma, M2 macrophages and bone marrow stromal stem cells proved to be crucial components for predictive assessment of implant materials in vitro . Biocompatibility was demonstrated for all tested materials, whereas the degree of deposited mineralized extracellular matrix and mechanical stability differed between the tested materials. Highest amount of functional biomineralization was determined to be on carbon-coated implant surface.
Significance
As an ethical alternative to animal testing, the established in vitro dental test system provides an economic and mid-throughput evaluation of novel dental implant materials or modifications thereof, by applying two successive readout levels: biomineralization and osseointegration.
1
Introduction
Dental implants have become a widely accepted and preferred tooth replacement therapy for the treatment of tooth and hard and soft tissue loss . Increasing life expectancy and preservation of population’s quality of life in all age groups lead to a growing need for oral implants . Thus, approximately 100,000–300,000 dental implant placements are performed annually in the USA alone and the global dental implant market was worth $3.3 billion in 2010 with expectations that this will grow further . However, some implants still fail after implantation for a plethora of reasons such as peri-implantitis , lack of osseointegration , material corrosion and, more recently, hypersensitivity .
In 1965, when Brånemark discovered the biocompatibility of titanium implants and the capability of titanium to integrate into host tissue, he introduced the term osseointegration and fostered an intensive research in the field of biomaterials . This resulted in dental implants being manufactured from titanium and its alloys, with processed titanium becoming the gold standard in oral implantology . Despite its excellent biocompatibility, high strength and low modulus of elasticity , some limitations, such as the resulting unnatural color of peri-implant soft tissue , corrosion and hypersensitivity , still exist and need to be overcome.
Thus, current research focuses on developing novel materials and material modifications, which both need to be tested for their safety, suitability, and effectiveness, if they are to be used as replacement dental structures in human beings. Zirconia ceramic has the potential to overcome at least some of the above-mentioned shortcomings of the actual – titanium-based – gold standard, and is therefore used in current research and development work.
Evaluation of newly developed materials is divided into three successive phases: in vitro tests, animal experiments and, finally, clinical studies. Only a small percentage of new materials/drugs/medicinal products passes all phases . Most materials fail because following this route, the barriers to success become more stringent, and the complexity of the test environment steadily increases. In line with rising complexity, evaluation costs and time also increase from the first phase to last phase. Thus, financial loss is highest when materials perform well during the in vitro phase but fail in (pre)clinical studies . Prior to clinical investigation, in vitro models allow easy, fast and cost-effective (in comparison to in vivo studies) evaluation of specific material properties such as surface topography or (bio)chemical composition and their effects on adhesion, proliferation and differentiation of various relevant cell types including osteoblasts , mesenchymal stromal stem cells (MSC) or cell lines . Although determination of these output parameters, with osseointegration capability as the ultimate criteria , can be used as an important indicator for evaluation of a cells’ response to the materials in vitro , such setups do not reflect the complexity of the surrounding physiological microenvironment. Indeed, many materials fail when tested in clinical trials or even prior to this in animal experiments, clearly indicating a need for a reliable in vitro test system that yields highly reproducible results with consideration to the biological response of the patient after implantation . Therefore, the aim of this study was to develop and validate the applicability of an in vitro test system that mimics a human intra-oral hard and soft tissue wound niche.
We aspired to increase the predictive power of preclinical evaluation of dental materials and consequently combined relevant biological components in our in vitro experimental design.
Following implantation, the created wound starts to heal. Wound healing is a strictly regulated process, which is highly conserved between the different organs and consists of four specifically timed and overlapping steps: hemostasis, inflammation, proliferation and tissue remodeling . The first step of wound healing begins with the cessation of bleeding and the formation of a fibrin clot (thrombus) . Due to a subsequent release of growth factors and pro-inflammatory cytokines, cells of the immune system, such as neutrophils, monocytes and lymphocytes, migrate to the wound site and promote the transition to inflammation . At the site of injury, monocytes also differentiate into macrophages, which are prominent players in the wound healing process . During the inflammatory process, they control the inflammatory response by recruiting additional leukocytes for phagocytosis of cell debris and bacteria. Simultaneously, through the release of different cytokines, the macrophages recruit and activate cells involved in normal tissue functioning . Thereby, macrophages prepare the wound for the next step, the proliferation stage. In this step, many processes take place in order to repair the wound, including angiogenesis, collagen deposition and wound consolidation . The last and final step is the remodeling of the tissue to a state similar to the initial organ structure .
When considering the body’s response to implants, the overall hypothesis of this study was that a reliable assessment of dental biomaterials can be undertaken, when a test system closely resembling the complexity of the wound healing process is used ( Fig. 1 ). Therefore, relevant human biological components were combined in a 3D test system that allowed the long term testing of dental implant materials. The following components were considered relevant due to their role in wound healing process: (1) Human plasma to simulate formation of fibrin clot. (2) M2 macrophages for recruitment of other cell types to the wound site. (3) Human mesenchymal stromal stem cells (hMSCs) as progenitor cells of osteoblasts, present in jaw bone and responsible for bone tissue formation, hence, osseointegration upon differentiation into osteogenic lineage.

In brief, following (i) the identification of components required for a reliable assay to assess dental implants, (ii) an assay that allows for distinguishing between different implant materials on the basis of biomineralization was developed. Moreover, (iii) biomineralization in vitro was confirmed as suitable readout parameter to predict the strength of the functional bonding between the recipient’s tissue and dental implant.
2
Materials and methods
Culturing of primary human cells as well as the dental implant test system were performed at 37 °C, 5% CO 2 in humidified atmosphere.
Analyses were performed at room temperature unless otherwise stated.
2.1
Ethical clearance statement
Granted by the Ethics Committee of the University of Wuerzburg (182/10, November 25th 2015) and informed consent of the donors, leukocyte concentrate and spongiosa tissue were used for isolation of relevant cell types. The detailed description of both isolation methods follows in the sections below.
2.2
Components of dental implant test system
2.2.1
Human plasma
Frozen human plasma (A, Rh+) was obtained from the Bavarian Red Cross blood donation service (Blutspendedienst des Bayerischen Roten Kreuzes, Munich, Germany). The contents of five plasma bags were mixed in equal quantities, aliquoted and stored at −80 °C until use.
For coagulation, plasma was mixed with 15 mM CaCl 2 (Merck, #1.02391, Darmstadt, Germany) and incubated for 30 min at 37 °C.
2.2.2
Isolation of monocytes and differentiation into M2 macrophages
Monocytes were isolated from fresh leukocyte concentrate under the approval of the Local Ethics Committee of the University of Wuerzburg (182/10, November 25th, 2015) and informed consent of the donors. Monocytes were isolated as described previously by ficoll gradient centrifugation (GE Healthcare, #17544203, Freiburg, Germany) and subsequent negative magnetic cell separation (Miltenyi Biotec, #130-096-537, Bergisch Gladbach, Germany). For differentiation into M2 macrophages, the obtained monocytes were cultured in RPMI 1640 GlutaMAX™ medium (Gibco™, Thermo Fisher Scientific, #61870, Dreieich, Germany) supplemented with 10% (by volume) fetal calf serum (FCS; Gibco™, Thermo Fisher Scientific, #10270106, LOT 41F1142K, Dreieich, Germany) and 40 ng/mL (by mass) recombinant human M-CSF (PeproTech, #300-25, Hamburg, Germany). Cells were cultured for 6 days, with complete medium exchange after 3 days.
2.2.3
Isolation and osteogenic differentiation of human mesenchymal stromal stem cells (hMSCs)
hMSCs were isolated from spongiosa tissue of a patient undergoing orthopedic hip replacement surgery, but who was otherwise healthy. The procedure was performed under the approval of the Local Ethics Committee of the University of Wuerzburg (182/10, November 25th, 2015) and informed consent of the patient (female, 56 years). hMSCs were isolated according to the procedure described in Noth et al. and cultured in expansion medium: DMEM/F-12 GlutaMAX™ medium (Gibco™, Thermo Fisher Scientific, #31331, Dreieich, Germany) supplemented with 10% (by volume) FCS (Biochrom, #S0115, LOT 0435A, Berlin, Germany), 5 ng/mL FGF-2 (Active Bioscience, #1370.950.050, Hamburg, Germany), 100 units/mL penicillin and 100 μg/mL (by mass) streptomycin (both SIGMA-ALDRICH, Merck, #P4333, Darmstadt, Germany). Complete medium exchange was performed every 3–4 days and cells were subcultured when 80% confluence was reached. Cells were used at passage 3 for the experiments.
For osteogenic differentiation of hMSC, cells at 100% confluence were cultured in osteogenic differentiation medium: DMEM, high glucose, GlutaMAX™ (Gibco™, Thermo Fisher Scientific, #61965, Dreieich, Germany) supplemented with 10% (by volume) FCS (Bio&SELL, #BS.FCS 0.500 EUA, LOT BS211507.5A, Feucht/Nuremberg, Germany), 50 μg/mL (by mass) L-ascorbic acid 2-phosphate (SIGMA-ALDRICH, Merck, #A8960, Darmstadt, Germany), 10 mM β-glycerophosphate (SIGMA-ALDRICH, Merck, #G9422, Darmstadt, Germany), 100 nM dexamethasone (SIGMA-ALDRICH, Merck, #D4902, Darmstadt, Germany), 100 units/mL penicillin and 100 μg/mL (by volume) streptomycin (both SIGMA-ALDRICH, Merck, #P4333, Darmstadt, Germany). Cells were cultured for 28 days with complete medium exchange every 2–3 days.
2.3
Dental implant test system
A bottom-up dental human implant test system composed of two consecutive levels was developed to address two different scientific questions: biomineralization and osseointegration. Both levels required the dental implant material being tested to take the form of small bowl with flat bottom (inner diameter: 15 mm; inner height: 7 mm) placed inside of commercially available 12-well plates (e.g. from TPP Techno Plastic Products AG, #92012, Trasadingen, Switzerland) for easy handling during 28 days of culture.
2.3.1
Biomineralization setup
The biomineralization setup was used to evaluate fundamental prerequisites of implant materials like biocompatibility and facilitation of extracellular matrix deposition. To accomplish this, each bowl of dental implant material was filled with 88.5 μL human plasma CaCl 2 solution, incubated for 30 min for coagulation and subsequently seeded with 5.31 × 10 4 M2 macrophages in 450 μL RPMI + 10% (by volume) FCS medium (cell density defined in ; equates 3 × 10 4 /cm 2 ). Following 2 h incubation period for initial cell attachment, 2 × 10 5 hMSC in 450 μL RPMI + 10% (by volume) FCS medium was added (cell density determined in preliminary test; corresponds 100% confluence; data not shown). Addition of human plasma, M2 macrophages and hMSC was done on a staggered basis to mimic the wound healing process; starting with fibrin clot formation, followed by migration of cells to the wound site. On the second day of culturing, the medium was exchanged with 900 μL of osteogenic differentiation medium. For implant material testing, constructs were cultured for 28 days with complete osteogenic differentiation medium exchange every 2–3 days.
2.3.2
Osseointegration setup
This setup was used to evaluate osseointegration of implant materials on bone substitute material. Here, CERASORB® M cylinders of 10.5 mm diameter and 4 mm height, manufactured by curasan AG (Kleinostheim, Germany), were used to mimic jaw bone. The osseointegration setup was based on the biomineralization setup, hence, assembling was done as previously described except for initially using only 150 μL medium for seeding of each specific cell type. Additionally, 2 h after human MSCs seeding, a CERASORB® M cylinder was placed on top of the plasma-M2 macrophages-hMSCs construct. Finally, 600 μL RPMI + 10% (by volume) FCS medium was carefully added to yield the same final medium volume as in the biomineralization setup. On the second day of culturing, the medium was exchanged with 900 μL of osteogenic differentiation medium. For implant material testing, constructs were cultured for 28 days with complete osteogenic differentiation medium exchange every 2–3 days.
2.4
Dental implant materials
All dental implant materials used for the establishment of the two-level in vitro dental test system were obtained from Natural Dental Implants AG (Berlin, Germany) in the form of custom-made small bowls with a flat bottom.
2.4.1
Titanium-based materials
Pure Grade IV titanium was used for the production of two different surface formulations. “Machined titanium” resulted after milling and cutting raw material, and provided a blank material for study A (proof-of-concept study of biomineralization setup). “Acid-etched titanium” was obtained after performing additional post-processing steps: sandblasting; etching in hydrofluoric and sulfuric acid; and rinsing with clean water under ultrasound application. “Acid-etched titanium” is the actual gold standard among dental implant materials, thus serving as a positive control for studies A and C (proof-of-principle study of osseointegration setup).
2.4.2
Ceramic-based materials
Zirconia ceramics were used for production of two different surface formulations, evaluated within study B and study C. “Unmodified ceramic” resulted after sintering of raw material, etching in hot hydrofluoric acid and rinsing. “Carbon-coated ceramic” was obtained after performing additional surface coating with carbon-rich deposits that resulted in a variety of different carbon dangling bonds up to diamond-like particles.
2.5
Analyses
2.5.1
Cell metabolic activity assay
1 mg/mL (by mass) 3-(4,5-dimethyl-2-thiazolyl)-2,5-diphenyl-2H-tetrazolium bromide (MTT; SERVA Electrophoresis GmbH, #20395, Heidelberg, Germany) was added to osteogenic differentiation medium at the end of 28 days of in vitro culture. For the osseointegration setup, bowls and CERASORB® M were treated separately. After 3 h of incubation at 37 °C, conversion of yellow MTT into blue formazan was documented by taking macroscopic pictures. For quantification, samples were incubated in isopropanol for 30 min with ambient agitation to extract formazan into solution. Absorption of technical triplicates was measured at 570 nm with infinite M200 plate reader and i-control 1.11 software (Tecan Trading AG, Maennedorf, Switzerland). The resulting values refer to all cell types present in the cells-plasma-implant material composite (study A, B and C) or cells-plasma-CERSORB® M composite (study C).
2.5.2
Mineralization assay
Samples were fixed in methanol (10 min, −20 °C), washed in demineralized water and subsequently stained with Alizarin Red (MORPHISTO GmbH, #13154, Frankfurt am Main, Germany) for 5 min. For the osseointegration setup, only hMSC-M2 macrophage-plasma-implant material composites were treated because CERASORB® M yields in high background signal due to composition of β-tricalcium phosphate. Following two washing steps in demineralized water, macroscopic pictures were taken to document red staining of deposited calcium. For quantification, samples were incubated in 0.5 M HCl containing 5% (by mass) SDS for 30 min with ambient agitation to extract red staining of deposited calcium into solution. Absorption of technical triplicates was measured at 415 nm with infinite M200 plate reader and i-control 1.11 software (Tecan Trading AG, Maennedorf, Switzerland).
2.5.3
Bone bonding test
Integration between bone substitute CERASORB® M and underlying hMSC-M2 macrophage-plasma-implant material composite was evaluated using a force measuring device from SAUTER GmbH (digital force gauge FH 5; capacity 5 N; d = 0.001 N, Balingen, Germany) and a custom-built test stand. Measurements were performed with medium-filled bowls and obtained peak values were considered for analysis.
2.5.4
Statistical analysis
Statistical analysis was performed with GraphPad Prism 6.07. Following tests for outlier and Gaussian distribution, the data was either compared using an unpaired t -test (normally distributed values) or a Mann–Whitney test (not normally distributed values or n = too small). A p -value <0.05 was considered statistically significant.
3
Results
3.1
Development of the test system
The two-level human in vitro test system for the evaluation of dental implant materials ( Fig. 1 ) was developed based on the outcome of three consecutive experimental studies, each defined by a specific scientific objective. To identify the relevant components of the 3D test system (study A), combinations of human plasma, M2 macrophages and mesenchymal stromal stem cells were used to compare two implant materials – “acid-etched titanium” versus “machined titanium” – with described clinical outcome. Following the identification of the test components that mimic the wound niche, the biomineralization setup’s sensitivity and capacity to distinguish between different materials were evaluated by using different implant material formulations, namely “unmodified ceramic” versus “carbon-coated ceramic” (study B). Finally, the readout parameter of the biomineralization setup was evaluated to determine the functional integration of the implant into the recipient’s bone (study C).
In total, we have used four materials in three studies: “acid-etched titanium” (study A and C), “machined titanium” (study A), “unmodified ceramic” (study B) and “carbon-coated ceramic” (study B and C). Processed titanium was used because it is the actual gold standard for dental implants. However, there are some limitations including the coloration of peri-implant soft tissue, which can be read in the introduction. Ceramic has the potential to overcome at least some of the shortcomings, and was therefore included in the experimental setup.
As a general procedure in all three studies, implant-based constructs were cultured for 28 days in vitro and subsequently analyzed for cellular metabolic activity, intensity of biomineralization and bone bonding capacity (only study C).
3.2
Proof-of-concept study of 3-component biomineralization setup with human plasma, M2 macrophages and mesenchymal stromal stem cells: “acid-etched titanium” versus “machined titanium” (study A; reference material: standard tissue culture treated polystyrene)
Considering the biological components found in close proximity to tooth space and their postulated contribution to wound healing and osseointegration of inserted dental implants, we hypothesized that human plasma, M2 macrophages and mesenchymal stromal stem cells (hMSC) are necessary components for generating a test system able to distinguish between implant materials on the basis of mineralization capability. To prove this, we compared the amount of deposited mineralized matrix on the actual gold standard material “acid-etched titanium” against blank “machined titanium” and correlated the outcomes of three different groups comprising all 3 components or specific combinations of just 2 components thereof. The most mineralized matrix was detected on the “acid-etched titanium” in group 3, containing all three above-mentioned biological components; whereas almost no mineralized matrix could be found in group 1 and group 2 using this material, with both lacking one of these three biological components ( Fig. 2 B ). In order to establish a predictive test system for dental implant materials, it is mandatory to demonstrate a distinctive outcome by using highly different materials with known outcome in the clinical practice or in in vitro studies. Therefore, we included blank “machined titanium” as a material, for which it was expected to induce significantly smaller amounts of calcium deposition . This assumption could be confirmed by qualitative and quantitative comparisons of “machined titanium” versus “acid-etched titanium” which showed significant difference ( p = 0.0185; Fig. 2 B) in the group 3 setup that contained all three necessary biological components. Because cells cultured on both materials and all three group compositions exhibited the same level of metabolic activity ( Fig. 2 A), detected differences in mineralized matrix deposition are not caused by biological incompatibility of these implant materials. For reference purpose, a third, well-established and freely available, material was included: standard tissue culture treated polystyrene (TPP Techno Plastic Products AG, #92024, Trasadingen, Switzerland). In all three polystyrene group settings, cells exhibited the same level of metabolic activity ( Fig. 2 A), but statistically different levels of deposited mineralized matrix. In contrast to the respective titanium-based materials, the highest levels of deposited mineralized matrix were observed in group 2 – containing human plasma and hMSC, followed by group 3 – containing human plasma, hMSC and M2 macrophages ( Fig. 2 B). Despite this interesting finding using standard tissue culture treated polystyrene, the developed dental implant test system shall be used for relevant dental implant materials including titanium and modifications thereof, and therefore the group 3 setting was chosen as the standard for the biomineralization setup.
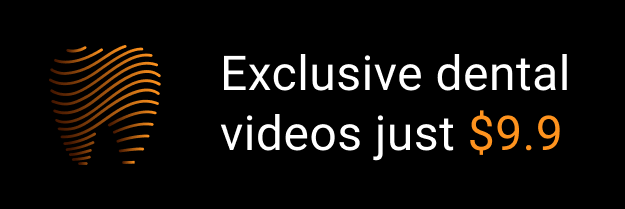