Abstract
Introduction
The hypothesis was that experimental ion-leaching bioactive composites enhance remineralization of apatite-depleted dentin.
Materials and methods
Calcium-aluminosilicate (wTC-Ba) or fluoride-containing calcium-aluminosilicate (FTC-Ba) Portland-derived mineral powders were mixed with HTP-M methacrylate HEMA/TEGDMA/PAA-based resin to prepare experimental composites. Controls were Vitrebond and Gradia Direct LoFlo.
Calcium- and fluoride-release, pH of soaking water, solubility and water uptake were evaluated in deionized water using material disks (8 mm diameter and 1.6 mm thick).
The apatite-formation ability (bioactivity) and the ability to remineralize previously demineralized dentin were assessed by ESEM-EDX and FTIR after soaking in a phosphate-containing solution.
Human dentin slices (0.8 mm thickness) were demineralized in EDTA 17% for 2 h, placed in close contact with the material disks and immersed in a phosphate-containing solution (Dulbecco’s Phosphate Buffered Saline, DPBS) to assess the ability of the materials to remineralize apatite-depleted dentin.
Results
Only the experimental materials released calcium and basified the soaking water (released hydroxyl ions). A correlation between calcium release and solubility was observed. FTC-Ba composite released more fluoride than Vitrebond and formed calcium fluoride (fluorite) precipitates. Polyacrylate calcium complexes (between COO − groups of polyacrylate and released calcium ions) formed at high pH.
The formation of apatite was noticed only on the experimental materials, due to the combination of calcium ions provided by the materials and phosphate from the DPBS. Apatite deposits (spherulites showing Ca and P EDX peaks and IR bands due to phosphate stretching and bending) were detected early on the experimental material disks after only 24 h of soaking in DPBS.
Only the experimental composites proved to have the ability to remineralize apatite-depleted dentin surfaces. After 7 days in DPBS, only the demineralized dentin treated with the experimental materials showed the appearance of carbonated apatite (IR bands at about 1400, 1020, 600 cm −1 ). EDX compositional depth profile through the fractured demineralized dentin slices showed the reappearance of Ca and P peaks (remineralization of dentin surface) to 30–50 μm depth.
Conclusions
The ion-leachable experimental composites remineralized the human apatite-depleted dentin. Ion release promotes the formation of a bone-like carbonated-apatite on demineralized dentin within 7 days of immersion in DPBS.
The use of bioactive “smart” composites containing reactive calcium-silicate Portland-derived mineral powder as tailored filler may be an innovative method for the biomimetic remineralization of apatite-depleted dentin surfaces and to prevent the demineralization of hypomineralized/carious dentin, with potentially great advantage in clinical applications.
1
Introduction
Dentin is a complex tissue, which contains apatite as mineral phase, collagen and other proteins, and water . Initial carious lesions affect the mineral phase of dentin and expose the collagen fibers creating the conditions for a fast destruction of the entire dentin network .
An important requirement for operative and preventive dentistry is the development of restorative “smart” materials able to induce the remineralization of hypomineralized carious dentin (demineralized/carious dentin). At present no restorative materials with proven capability to induce dentin remineralization are available on the market.
The remineralization of demineralized dentin ( bioremineralization ) is the process of restoring minerals through the formation of inorganic mineral-like materials .
Recently, experimental remineralizing resin-based calcium phosphate cements (ion-leaching composites) have been proposed as restorative materials to induce dentin remineralization .
Biomimetic remineralization (bioremineralization) of dentin has been investigated with different methods using ion-containing solutions or ion-leaching silicon-containing materials (mainly bioactive glasses): solutions containing Ca 2+ , SiO 4 4− , F − or PO 4 3− ions , bioactive glasses placed on dentin , remineralization solutions supplemented with a bioactive glass and remineralizing solutions containing the ions leached from ultrafine bioactive glass particles , glass-ionomer cements containing a bioactive glass in dog restorations , MTA cement layered on the dentin surface , Portland cement blocks (as a source of calcium and hydroxyl ions) immersed in a biomimetic analog consisting of simulated body fluid added with polyacrylic acid and polyphosphonic acid) or poly(vinyl phosphonic acid (PVPA) .
In most of these studies dentin was immersed in solutions containing ions leached from different silicate-based materials without dentin-material contact, and consequently long times (14 days to 1 month) are required to achieve the remineralization of dentin.
Calcium-silicate cements (conventionally termed mineral trioxide aggregate MTA cements, such as ProRoot MTA, MTA Angelus, Tech Biosealer) are Portland-derived cements that have been introduced in dentistry as materials for different endodontic clinical applications .
Calcium-silicate cements are hydrophylic materials able to tolerate moisture (hydraulic materials) and to polymerize and harden (setting) also in the presence of biological fluids (blood, plasma, saliva, dentinal fluid). They are ion-leaching materials able to release calcium and hydroxyl ions (alkalinizing activity) into the surrounding fluids, creating the conditions for apatite formation . In detail, calcium-silicate particles hydrate and decalcify after mixing with water following the formation of CSH gel (calcium-silicates hydrates) and calcium hydroxide .
Calcium-silicate cements possess bioactive behavior i.e. stimulate the formation of new apatite-containing tissues, since they are biointeractive materials able to develop apatite on their surface in a short induction period and able to elicit a positive response at the interface from the biological environment . They showed excellent clinical results possibly related to their biocompatibility and bioactivity (i.e. apatite-forming ability) properties .
The aim of this study was to develop bioactive calcium-releasing light-curable hydrophilic composites with tailored remineralizing properties, to be used as restorative base-liner materials in sandwich restorations. Moreover, to test the remineralization of dentin by the experimental composites, a new experimental set-up was proposed involving the dentin-material contact, with the aim to mimic clinical conditions.
2
Materials and methods
2.1
Materials
Two experimental composites (named wTC-Ba + HTP-M and FTC-Ba + HTP-M ) containing calcium-silicate Portland-derived hydrophilic mineral fillers (2–20 μm-sized particles) with tailored enhanced reactivity and a light-curable hydrophylic resin (1 g mineral powder/0.8 g of resin) were designed and prepared [Gandolfi, University of Bologna, Italy].
The experimental composite wTC-Ba + HTP-M was composed of a reactive calcium-aluminosilicate powder, wTC-Ba [containing tricalcium-silicate 3CaO·SiO 2 , dicalcium-silicate 2CaO·SiO 2 , tricalcium-aluminate 3CaO·Al 2 O 3 , calcium sulfate and barium sulfate], mixed with an experimental light-curable hydrophylic resin, HTP-M [containing HEMA, TEGDMA and polyacrylic-co-maleic acid, EDMAB and camphorquinone]. HEMA, TEGDMA and polyacrylic-co-maleic acid obtained from Sigma-Aldrich, Steinheim, Germany.
The experimental composite FTC-Ba + HTP-M was composed of a fluoride-containing calcium-aluminosilicate powder, FTC-Ba [i.e. wTC-Ba added to sodium fluoride], mixed with the light-curable hydrophylic resin HTP-M.
Vitrebond [3 M, St. Paul, MN, USA; lot 9NN] was used as HEMA-PAA-containing control base material. Vitrebond (resin-reinforced glass-ionomer cement) consisted of a fluoro-aluminosilicate powder [SiO 2 , AlF 3 , ZnO, SrO, Na 3 AlF 6 (criolite), NH 4 F, MgO, P 2 O 5 ] and a light-curable liquid [PAA, HEMA, water and photoinitiator] . Vitrebond was prepared following manufacturer directions [1 spoon (0.033 g)/1 drop (0.05 g)].
Gradia Direct LoFlo A3 [GC, Tokyo, Japan; lot 1001271] was used as light-cured flowable control composite/base material. Gradia contained a silica prepolymerized filler (0.85 μm size) and UDMA methacrylate monomers.
The materials were prepared by mixing the mineral powder with the resin on a glass plate to form a homogeneous paste. PVC molds (8 mm diameter and 1.6 mm thick) were used to prepare material disks. Each disk was light-cured on each side using a LED unit (Anthos, Imola, Italy). Light-curing time was 30 s for the commercial materials and 100 s for the experimental composites.
The materials were characterized for their chemical–physical properties (setting times, solubility, water absorption, alkalinizing activity, calcium and fluoride release) and bio-properties (apatite forming ability, dentin remineralization).
2.2
Chemical–physical properties
2.2.1
Setting times
Gilmore setting times (initial and final setting times) were evaluated by the penetration measurements of specific needles (initial setting Gilmore needle weight 113.4 g and diameter 2.12 mm; final setting Gilmore needle weight 453.6 g diameter 1.06 mm). Setting times corresponded to the lack of a complete circular impression (i.e. no indentation mark) on the specimen surface .
2.2.2
Solubility
According to ISO 6876, 2002 , the specimens were weighed (Initial weight) and placed in sealed cylindrical polystyrene holders (3 cm high and 4 cm in diameter) containing 15 mL of deionized water, at 37 °C. After 1 and 28 days, the samples were removed from the solutions and dried to constant weight.
The solubility (percentage weight variation, Δ W %) at each time t was calculated according to the following equation:
Δ W % = Dry weight at time t − Initial weight Initial weight × 100
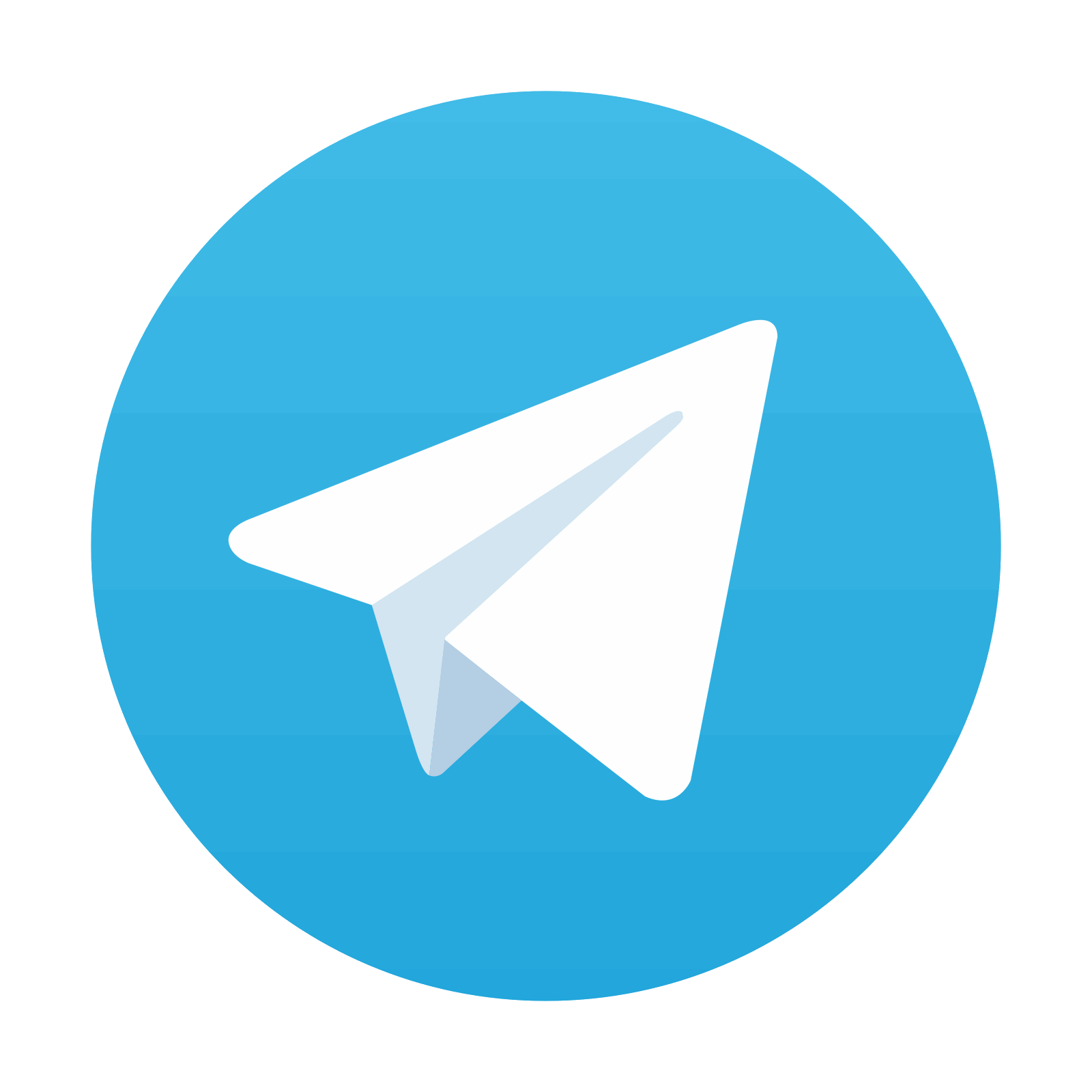
Stay updated, free dental videos. Join our Telegram channel

VIDEdental - Online dental courses
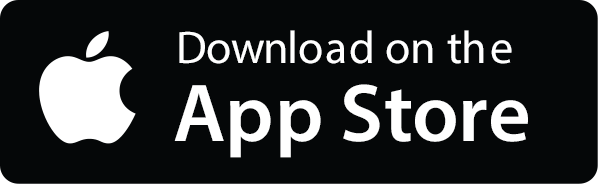
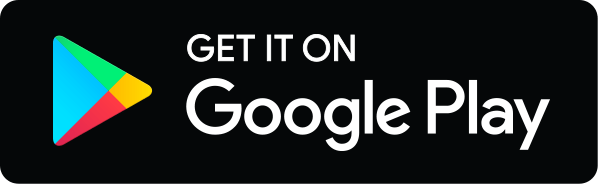