Abstract
Objectives
This study evaluated the ability of a novel sol–gel bioactive glass, in conjunction with appropriate carrier vehicles, to reduce dentinal fluid flow, with an eye toward reducing dentinal hypersensitivity.
Methods
Experiments were conducted to measure the reduction in tubule fluid flow after treatment of cut tooth surfaces with sol–gel bioactive glass particles in several carrier vehicles. Surfaces were also examined after exposure to brushing and acidic solutions. A non-bioactive particulate glass was compared.
Results
Tubular occlusion produced by the bioactive glass was observed via SEM and a sustained reduction in hydrodynamic conductance was measured after exposure to various fluids and brushing.
Conclusions
This new material may be used with the tested carriers to significantly and durably reduce tubule fluid flow, ultimately resulting in reduced dentinal hypersensitivity.
1
Introduction
It has been stated 8–35% of the population in the U.S. at any one time suffers from dentin hypersensitivity , but there are estimates as high as 74% . It also has been reported that root sensitivity increases from 9 to 15% pre-periodontal surgery, to 55% post-surgery . In vivo studies provide strong evidence that the sensitivity originates from the exposure of open dentinal tubules at the surface of the tooth and is caused by changes in the local environment, including temperature, pressure, osmolality, and chemical agents. These local environment changes cause fluids in the dentinal tubules to move quickly, stimulating the nerves surrounding the odontoblasts and giving rise to pain as postulated in the hydrodynamic theory . Typical methods to alleviate the effects of dentin hypersensitivity include occluding the open tubules with organic or inorganic chemicals and compounds, as well as applying chemical agents that suppress pain expression . The general consensus is that the most effective and durable method for achieving relief would be to seal the dentin surface through tubule occlusion, thereby eliminating or minimizing movement of fluids in the tubules .
Various agents have been packaged as both in-office, as well as over-the-counter medicaments for treating tooth hypersensitivity . However, there currently is no ideal system for performing this task. Potassium oxalate, potassium chloride, glutaraldehyde, resin monomers, and other materials have been used either in dentifrices or as single or multiple application agents to occlude dentinal tubules. While these materials have been shown to provide some relief, none have been proven to be completely effective. Recently, a bioactive glass has been added to a dentifrice for this express purpose . Tubule occlusion was examined by scanning electron microscopy, and shown to be enhanced to some extent with certain formulations. However, no attempt was made to study the ability of the agent to reduce dentin permeability. The durability of the surface layer also was not studied, though it was suggested that it is easily washed off with water.
Bioactive glasses have been shown to promote the crystallization of new mineral on their surfaces . Other studies have verified the precipitation of a calcium phosphate ceramic on the surface of a tooth after application of a bioactive glass (Bioglass ® ), providing evidence for the potential of this form of treatment . Bioactive glasses can be formulated by the sol–gel process such that they have varying rates of dissolution and mineral formation in biologic environments .
The objective of this study was to examine in vitro the dentinal tubule sealing ability of a nano-structured sol–gel bioactive glass preparation delivered on exposed dentinal tubules and its effects on hydraulic conductance of human dentin. Furthermore, we assessed the physical durability of this sealing, as measured by its resistance to aggressive solutions and toothbrushing, and identified appropriate vehicles and delivery systems for its application. It was hypothesized that this novel material may potentially be used as a dental desensitizing agent.
2
Materials and methods
2.1
Materials
We synthesized bioactive glass (BAG), using a sol–gel method. The composition of this glass was 65 mol% SiO 2 , 31 mol% CaO, and 4 mol% P 2 O 5 .
To maintain the highest homogeneity possible, all starting compounds were high-purity metal alkoxides. Tetraethyl orthosilicate (TEOS, C 8 H 20 O 4 Si) was used as the precursor for silica (SiO 2 ) in the final glass; calcium methoxyethoxide (CMOE, C 6 H 14 O 4 Ca) was the precursor for lime (CaO); and triethyl phosphate (TEP, C 6 H 15 O 3 PO) was used to yield phosphate (P 2 O 5 ). All of the alkoxide reagents were purchased commercially (Sigma–Aldrich). The methoxyethanol solution served as a mutual solvent for all of the alkoxides. The solutions were prepared in a dry nitrogen environment, and water vapor was introduced by aging them in a 100% humidity chamber at 37 °C. This served to initiate hydrolysis and glass formation. After mixing, the resulting glasses were cast into polyethylene containers and allowed to hydrolyze undisturbed for up to 3 weeks. They were aged in distilled water, air-dried and stabilized in a furnace at 600 °C, using a ramp and soak sequence over two days to completely remove residual alcohols and alkoxide components, while retaining high surface area through moderate–high porosity formation. After rapidly cooling, the glasses were coarsely crushed with mortar and pestle, and subsequently ball-milled with zirconia beads for 8 h in an ethanol solution. The resulting powders were dried at 37 °C and sieved to less than 35 μm. The particles underwent further processing in a micronizer (Sturtevant, Hanover MA), resulting in a distribution of particles with a size range from less than 1 μm to about 20 μm, as measured by laser diffraction (Malvern Mastersizer 2000, Malvern Instruments, Westborough, MA). The surface area of all powders was measured using the BET technique (Quantasorb, Quantachrome Corp., Syosset, NY).
In order to verify the bioactivity of these powders, samples (10 mg) were immersed in 5 ml of Simulated Body Fluid (SBF) for up to 14 days (see Table 1 ). Following immersion, the powders were rinsed, dried and examined using Fourier Transform Infrared Spectroscopy (FTIR).
Ionic species | Concentration in SBF (mM) |
---|---|
Mg 2+ | 1.5 |
Ca 2+ | 2.5 |
K + | 5.0 |
Na + | 142.0 |
SO 4 2− | 0.5 |
HPO 4 2− | 1.0 |
HCO 3 − | 4.2 |
Cl − | 147.8 |
2.2
Tooth preparation
Dentin/pulp chamber specimens ( n = 3–5 for each treatment) were prepared from human teeth as shown in Fig. 1 . In brief, intact human molars free from caries and restorations were sectioned perpendicular to the long axis of the tooth using a low-speed rotary saw and a diamond wafering blade. The initial cut was made apically and far enough below the dentin–enamel junction so as to leave a small amount of the original pulp chamber. The occlusal surface of the tooth was removed with a second cut parallel to the first and just superior to the pulp horns. This resulted in a thin cylinder of tooth with intact dentin on the surface of the sample. The prepared tooth was mounted on an acrylic block using cyanoacrylate adhesive (Zap-it; Dental Ventures of America) through which a 15 gauge stainless steel (SS) tube had been inserted to provide entry into the pulp chamber. The fresh dentin surface was ground using #600 SiC until perfusion of water through the dentin was visible with moderate pressure applied through the SS tube via a water-filled syringe. Care was taken to not perforate the thin dentin layer. The SS tube was used throughout the remainder of the experiments when the specimens were being aged to provide 20 cm pressure with SBF to replicate physiologic conditions.
Each tooth surface was lightly etched with 35% phosphoric acid (15 s) on a microbrush to remove the resulting smear layer and open the tubules. Following etching, the surface was rinsed for 10 s and blotted dry with tissue, and then hydraulic conductance measurements were made as detailed below.
2.3
Experimental design
All conductance measurements were made following a modified procedure described by Pashley et al. , using a FloDec Instrument (DeMarco Engineering, Switzerland) with SBF as the test fluid. In order to ensure a significant rate of conductance through the tubules, a relatively high pressure of 90 cm of SBF was maintained throughout the testing period. This pressure was achieved by arranging the SBF supply at a height of 90 cm above the dentin surface.
As an initial investigation, BAG powder, prepared as described above, was mixed for 60 s with 35% phosphoric acid gel (PA) as a carrier fluid. Samples were prepared as a 15 wt% mixture of the powder. The resulting slurry was immediately applied to the dentin surface and lightly brushed for 60 s ( n = 5). As a non-bioactive control, we also prepared the slurry using a commercially available silica nanofiller as a thickening agent (OX50; 40 nm size; 50 m 2 /g, OX-50; Degussa Inc., Germany) ( n = 3). These particles, due to their small size, may penetrate and cause some occlusion of dentin tubules, but have no demonstrated ability to promote re-mineralization on dentin surfaces. These tests were conducted to ascertain whether any reduction in conductance was due to mere physical obturation of the tubules. Additional teeth samples were brushed with the carrier alone (without added particles) to observe the effect of PA on conductance ( n = 3). Following the treatment, samples were rinsed with water and conductance measurements were made corresponding to 0, 1, 2, 96 and 168 h after treatment. Teeth were stored in sealed containers at 100% relative humidity at 25 °C under physiologic pressure (20 cm SBF) between tests. Data are reported as a percent reduction in fluid conductance.
Following these initial investigations, additional carrier fluids were selected for testing. This was necessitated by the fact that the bioactive glass began to gel in the phosphoric acid within minutes, thus providing inadequate stability. Thus, non-acidic, essentially non-toxic, and water soluble solutions were investigated. These were ethanol, propylene glycol (PPG), hydroxyethyl methacrylate (HEMA), and glycerol. Teeth were prepared as above, with conductance measurements being made after preparing the samples (with the smear layer), after etching the samples with PA to remove the smear layer and open the tubules, and after allowing the specimen to remain undisturbed in sealed containers at 100% relative humidity at 25 °C under physiologic pressure (20 cm SBF) for 1 h after etching. Following this 1-h period, the slurries were applied by microbrush for 60 s, rinsed with water and conductance measurements were again made at 1 h, 24 h, 4 days and 1 week after application ( n = 3). Teeth were again stored in sealed containers at 100% relative humidity at 25 °C under physiologic pressure between measurements. The specimen treatment conditions are summarized in Table 2 .
Treatment | n | Fluid | Particles |
---|---|---|---|
Tx 1 | 5 | Phosphoric acid gel | BAG |
Tx 2 | 3 | Phosphoric acid gel | OX-50 |
Tx 3 | 3 | Phosphoric acid gel | None |
Tx 4 | 3 | Ethanol | BAG |
Tx 5 | 1 | Ethanol | None |
Tx 6 | 3 | Propylene glycol | BAG |
Tx 7 | 1 | Propylene glycol | None |
Tx 8 | 3 | HEMA | BAG |
Tx 9 | 1 | HEMA | None |
Tx 10 | 3 | Glycerol | BAG |
Tx 11 | 1 | Glycerol | None |
Tx 12 | Ethanol | OX-50 | |
Tx 13 | HEMA | OX-50 | |
Tx 14 | Propylene glycol | OX-50 | |
Tx 15 | Glycerol | OX-50 |
2.4
Durability measurements
To assess durability of the reduction in conductance, a group of specimens ( n = 5) was treated with BAG in glycerol, and subsequently exposed to a series of toothbrush abrasion tests (see Fig. 2 ). Glycerol was selected for these investigations since it provided the highest reduction in conductance of the four solutions and was therefore deemed to be the most promising carrier vehicle. The tooth was mounted on a rotating wheel attached to a DC gear motor and subjected to abrasion from a soft bristle toothbrush (Oral B #30, Iowa City, IA) while being rotated through water/toothpaste slurry containing 1 tube (181 g) of toothpaste in 500 ml water at 60 rpm for 5 min . Fluid conductance testing was performed before and immediately after brushing (specimens were rinsed with copious water to remove the toothpaste and then blotted dry with tissue), as well as 24 h after toothbrushing (the sample was kept under physiologic pressure between events). Next, the toothpaste/water slurry was replaced sequentially with undiluted cola (pH = 2.5), undiluted grapefruit juice (pH = 3.1), and finally coffee (pH = 5.7), and the sample was cycled without brushing through these fluids for five minutes each. Conductance was again measured before and after each exposure. A set of control teeth which were etched but had nothing applied were also tested after each procedure ( n = 5).
Scanning electron microscopy (SEM) (6400, JEOL Corp., Peabody, MA) was used to examine surfaces of the samples before and after treatment. These samples were dried and sputter-coated (Hummer VII, Anatech USA, Union City, CA) with approximately 10 nm of gold/palladium.
2.5
Statistical analyses
Statistical analyses were used to examine the change in % of original conductance measured. Comparisons between carriers over time were analyzed with 2-way ANOVA/Tukey’s test ( p ≤ 0.05), while 1-way ANOVA/Tukey’s test ( p ≤ 0.05) was used to compare the effects of BAG vs. OX-50 in the same carrier (phosphoric acid) and PA.
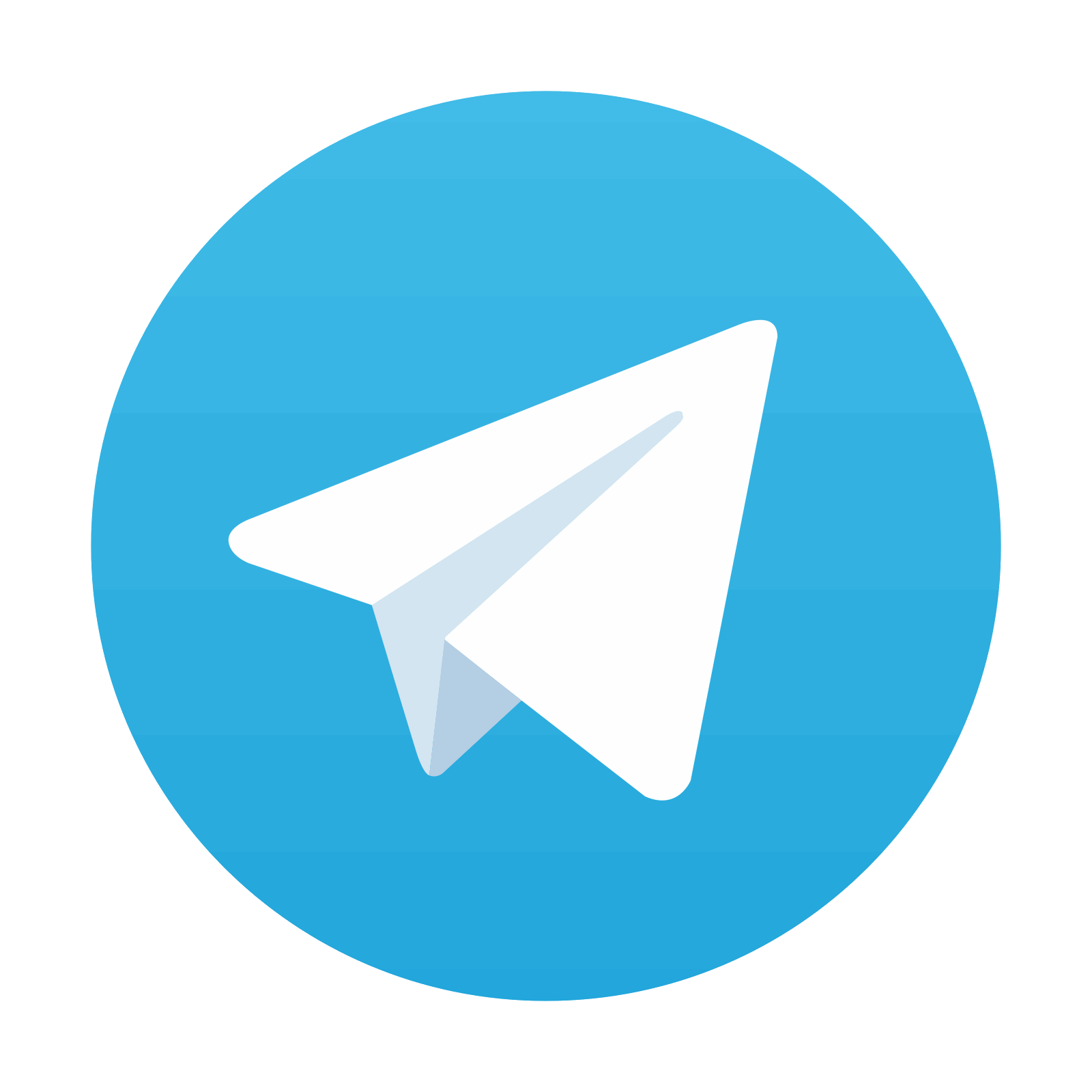
Stay updated, free dental videos. Join our Telegram channel

VIDEdental - Online dental courses
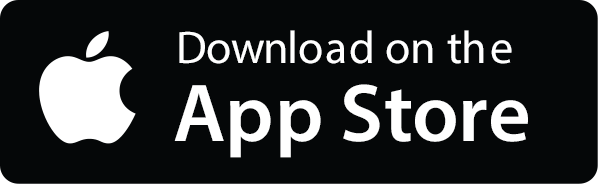
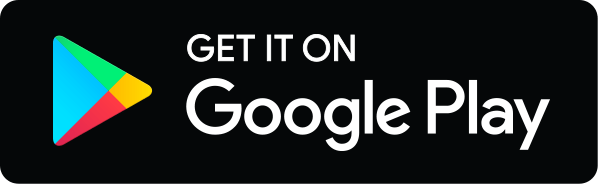