Abstract
Objectives
Composites may undergo biodegradation in the oral cavity. The objective was to investigate the effect of single- and multi-species biofilms on the surface roughness and topography of two composites.
Methods
Disk-shaped specimens of a paste-like, Bis-GMA-free (Gradia Direct Anterior, GC), and a flowable, Bis-GMA-based composite (Tetric EvoFlow, Ivoclar-Vivadent) were prepared. After ethylene-oxide sterilization (38 °C), specimens (n = 3) were incubated with Streptococcus mutans or mixed bacterial culture ( Streptococcus mutans , Streptococcus sanguinis , Actinomyces naeslundii and Fusobacterium nucleatum ). As negative controls, unexposed specimens and specimens exposed to sterile medium (BHI) were used. Specimens exposed to acidified BHI medium (pH = 5) and enzymatic solution of cholesterol esterase served as positive control. Following 6-week incubation, the attached biofilms were collected for real-time PCR assessment, after which the surface roughness and topography of the specimens were analyzed with atomic force microscopy. Surface hydrophilicity/hydrophobicity was determined by contact angle measurements. Biofilm structure was analyzed with scanning electron microscopy.
Results
Even though multi-species biofilms were thicker, with more cells attached, they did not significantly affect the surface roughness of the composites. On the other hand, S. mutans alone significantly increased the roughness of Tetric by 40.3%, while its effect on Gradia was lower (12%). The total amount of attached bacteria, however, did not differ between the composites.
Conclusions
S. mutans can increase the surface roughness of composites, depending on their composition. This ability of S. mutans is, however, mitigated in co-culture with other species. In particular, bacterial esterases seem to be responsible for the increased composite surface roughness upon biofilms exposure.
Clinical significance
Cariogenic bacteria can degrade composites, thereby increasing the surface roughness. Increased roughness and subsequent improved bacterial accumulation may facilitate the development of secondary caries around composites, which is the most common reason for the restoration failure.
1
Introduction
Composites have become the standard first-line restorative material in many countries, since they outperform amalgams in a number of clinical aspects, such as aesthetic appearance, more conservative preparation technique, clinical versatility etc . However, many researchers have reported that composites have a shorter lifespan compared to amalgams, which has mainly been attributed to their higher vulnerability to secondary caries (SC), the leading cause of restoration failure .
The shorter lifetime of composites has received increasing attention in recent years, and possible reasons, as well as solutions, for the increased susceptibility of composites to SC have extensively been investigated . Many studies have focused on plaque accumulation on the composite surface, as the presence of dental plaque (dental biofilm) is essential for the development of both primary and secondary caries. Conventional composites seem to accumulate more plaque on their surface than other restorative materials and tooth enamel do . Possible explanations for this could be the lack of antibacterial and buffering properties of composites, the release of bacteria-stimulating compounds, or the specific surface characteristics of composite materials, such as surface roughness, topography, charge, hydrophobicity etc. . The role of surface roughness in biofilm accumulation has been well established, and it is well known that increasing roughness implies higher bacterial retention . However, below a certain threshold (Ra = 0.2 μm), when the surface is considered to be smooth enough, physicochemical properties of the material (chemical composition) start playing a more important role in bacterial adhesion .
In addition to that, one of the inherent drawbacks of composites is that they undergo biodegradation in the oral cavity. Enzymes from the class of the esterases, such as cholesterol esterase (CE) and pseudocholinesterase (PCE), found in saliva, can degrade the Bis-GMA/TEGDMA-based composite polymer matrix . More importantly, cariogenic bacteria from dental plaque were also shown to be capable of degrading the resin matrix of composites and adhesives, not only by their esterase activity , but also by producing acids and creating a low-pH environment .
In consequence, many researchers suggested that the degradation of composite surfaces by the attached biofilms can result in increased surface roughness, which will further promote bacterial accumulation, leading to a vicious circle with even additional damage to the composite surface . Nevertheless, it remains unclear to which extent a composite surface can be degraded by bacteria and whether this effect is significant enough to affect bacterial accumulation. Most of the studies in this field assessed the surface changes of composites with two-dimensional imaging techniques such as scanning electron microscopy (SEM), which do not allow quantification of the surface roughness . In addition, in most of the experiments, specimens were exposed to single-species bacterial cultures (typically Streptococcus mutans ), even though it is known that bacteria express different behavior when they grow in complex bacterial communities, such as the dental biofilm . Moreover, only little is known with regard to the mechanism of degradation. It is for example not clear to which extent bacterial biodegradation is caused by acidic metabolites or enzymes excreted by oral bacteria.
Therefore, the aim of this study was to assess the influence of S. mutans single-species and multi-species biofilms on the surface of two composite materials with different composition, by atomic force microscopy (AFM). In addition, the effect of cholesterol esterase (CE) and low pH were tested to elucidate the degradation mechanism. We also investigated whether biofilm-induced changes of the composite surface affected the amount of accumulated bacteria by real-time PCR. The null hypotheses were that 1) there is no difference in the effect of single- and multi-species biofilms on the surface properties of two tested composites, and 2) there is no difference in bacterial accumulation between the two composites.
2
Materials and methods
2.1
Specimen preparation
Disk-shaped specimens (2-mm thickness, 7-mm diameter) of a hybrid, Bis-GMA-free composite (Gradia Direct Anterior, GC, Tokyo, Japan) and a flowable, Bis-GMA containing composite (Tetric EvoFlow, Ivoclar-Vivadent, Schaan, Lichtenstein) were prepared using polytetrafluoroethylene molds ( Table 1 ). The composites were inserted into molds, pressed between two glass slides and cured for 40 s at each side with a polywave LED unit as per manufacturers’ instructions (output: 1400 mW/cm 2 ) (Bluephase, Ivoclar-Vivadent, Schaan, Lichtenstein), in order to obtain very flat and clean surfaces. The surface that was not planned to be exposed to biofilms was marked with “X” with a razor blade. The specimens were then cleaned ultrasonically in distilled water for 5 min, after which they were gas-sterilized with ethylene oxide (EtO) at 38 °C.
Material | Composition | Manufacturer |
---|---|---|
Gradia Direct Anterior | Conventional hybrid composite Resin (27%w): Urethane dimethacrylate (UDMA), dimethacrylates, trimethacrylates Fillers: Silica (38%w), Pre-polymerised resin fillers (35%w) |
GC, Tokyo, Japan |
Tetric EvoFlow | Flowable nano-hybrid composite Resin (37.6%w): Bisphenol A-glycidyl methacrylate (Bis-GMA), Urethane dimethacrylate (UDMA), Decandioldimethacrylate Fillers: Barium glass filler, Ytterbium trifluoride, Mixed oxide, Highly dispersed silica (41.1%w) Prepolymers (20.4%w) |
Ivoclar Vivadent, Schaan, Lichtenstein |
2.2
Bacteria and culturing conditions
Overnight cultures of Streptococcus mutans (ATCC 25175), Streptococcus sanguinis (LMG 14657), Actinomyces naeslundii (ATCC 51655) and Fusobacterium nucleatum (ATCC 10953) were centrifuged and resuspended in fresh Brain Heart Infusion broth (BHI, Becton Dickinson and Company, Franklin Lakes, NJ, USA). The concentration of each bacterial suspension was adjusted spectrophotometrically (600 nm) (GeneQuant 100, GE Healthcare, UK) to 2 × 10 7 CFU/ml. Sterile material specimens were placed in 24-well plates (one specimen per well), with the “X”-sides facing the bottom of the wells, and the smooth upper surfaces available for biofilm formation. In order to form single-species biofilms, we added 2 ml of S. mutans suspension into the wells with specimens, while 500 μl of suspensions of each of the four species ( S. mutans , S. sanguinis , A. naeslundii and F. nucleatum ) were added to the wells to form multi-species biofilms. As negative controls, specimens incubated with 2 ml of sterile BHI broth, as well as unexposed (baseline) specimens, were used. Specimens with S. mutans cultures and with sterile BHI broth were incubated (37 °C, 5% CO 2 ) under aerobic conditions, while the specimens exposed to multi-species suspension were cultured anaerobically (85% N 2 , 10% CO 2 , 5% H 2 ) for a period of 6 weeks. Bacterial growth medium was refreshed every 48 h, without disturbing the formed biofilm. As positive controls, we used composite samples exposed to acidified sterile BHI broth (acidic control) and to an enzyme solution of cholesterol esterase for the same period of time. The pH of BHI broth was adjusted to 5 by adding lactic acid (Sigma Aldrich, St. Louis, MO, USA), whereas the enzyme solution was prepared by dissolving cholesterol esterase enzyme (CE, Sigma Aldrich, St. Louis, MO, USA) in phosphate buffered saline (PBS) at the concentration of 0.5 U/ml, and was replaced every 48 h by fresh stock solution kept at −20 °C. The experiments were carried out in triplicate, and were repeated two independent times on different days, with new bacterial cultures and specimens.
2.3
Scanning electron microscopy of biofilms
Additional composite disk-shaped specimens with a deep notch going through the middle of the disks, were prepared and exposed to bacterial cultures as described in §2.2. After an incubation of 6 weeks, the specimens with attached biofilms were carefully rinsed twice with sterile PBS and fixed with 2.5% glutaraldehyde in 0.1 M sodium-cacodylate buffer, followed by a postfixation treatment with 1% osmium-tetroxide. After the specimens were dehydrated in ethanol solutions with increasing concentration (50, 70, 90 and 100%) and dried with hexamethyldisilazane (Acros Organics, Geel, Belgium), they were carefully broken into halves at the notch in order allow the observation of the longitudinal sections of the biofilms. The specimens were then mounted on specimen stubs, sputter-coated with gold, and analyzed with SEM (JSM-6610LV, JEOL, Tokyo, Japan).
2.4
Real-time PCR quantification
After six weeks of incubation, specimens with biofilms were carefully taken out of the wells with sterile tweezers, without disturbing the overlying biofilms, and rinsed twice in sterile PBS to remove loosely bound cells. Subsequently, specimens were incubated for 30 min (37 °C, 5% CO 2 ) with 0.05% trypsin-EDTA solution (Life Technologies, Paisley, UK) to loosen up the attached biofilm, after which they were sonicated for 5 min in 2 ml of PBS to completely detach bacterial cells from the specimens. Finally, 1 ml of the PBS containing bacterial cells from biofilms was collected in micro-centrifuge tubes and frozen until DNA extraction and real-time PCR quantification. Control samples were also incubated with 0.05% trypsin-EDTA solution, to exclude any possible effect of this solution on the specimen’s surface.
Bacterial DNA was extracted and purified using the QIAamp DNA Mini Kit (Qiagen, Hilden, Germany) following the protocol for gram-positive bacteria. Real-time PCR was performed using specific primers and probes (Eurogentec, Liège, Belgium) in a CFX96 Real-Time PCR System (BioRad, Hercules, CA, USA) ( Table 2 ). Standard curves for absolute quantification were constructed using serial dilutions of plasmid DNA. Real-time PCR assays were carried out in a total volume of 25 μl, consisting of 12.5 μl of 2x Master Mix (Eurogentec), 1 μl each of the forward primer, the reverse primer and the probe, and 5 μl of the DNA template. The thermal cycling protocol was as follows: 1 cycle at 50 °C for 2 min, 1 cycle at 95 °C for 10 min, followed by 45 cycles at 95 °C for 15 s and at 60 °C for 1 min.
Bacterial strain | Primers and probes (5′ → 3′) | Target gene | Fragment length |
---|---|---|---|
Streptococcus mutans (ATCC 25175) |
FP: GCC TAC AGC TCA GAG ATG CTA TTC T RP: GCC ATA CAC CAC TCA TGA ATT GA P: FAM-TGG AAA TGA CGG TCG CCG TTA TGA A-TAMRA |
gtfB | 114 bp |
Streptococcus sanguinis (LMG 14657) | FP: CAA AAT TGT TGC AAA TCC AAA GG RP: GCT ATC GCT CCC TGT CTT TGA P: FAM-AAA GAA AGA TCG CTT GCC AGA ACC GG-TAMRA |
tnpA | 75 bp |
Actinomyces naeslundii (ATCC 51655) | FP: TCG AAA CTC AGC AAG TAG CCG RP: AGA GGA GGG CCA CAA AAG AAA P: FAM-GGG TAC TCT AGT CCA AAC TGG CGG ATA GCG-TAMRA |
Gene encoding unknown protein | 96 bp |
Fusobacterium nucleatum (ATCC 10953) | FP: GGA TTT ATT GGG CGT AAA GC RP: GGC ATT CCT ACA AAT ATC TAC GAA P: FAM-CTC TAC ACT TGT AGT TCC G-TAMRA |
16S rRNA gene | 162 bp |
2.5
Atomic force microscopy
After the collection of bacteria from biofilms for real-time PCR analysis, all the specimens, including the controls, were cleaned ultra-sonically, first in ultra-pure water for 15 min (Milli-Q ® , Merck Millipore, Billerica, MA, USA), then in 3% sodium-hypochlorite for 5 min (Orphi Pharma, Belgium) and finally again in ultra-pure water for 5 min, to make sure that all the remaining bacteria and extracellular matrix were removed. AFM imaging was performed in AC mode (intermittent-contact mode) in air, with NanoWizard ® 3 BioScience AFM (JPK, Berlin, Germany) and silicon probes (PPP-NCSTR-10, NANOSENSORS™, Neuchatel, Switzerland). In total, four samples from each group (two from each independent experiment) were analyzed in three different areas. In each area of 100 by 100 μm (maximum scanning area of the AFM scanner), images were taken at three different spots (at least 36 images per group). In addition, in each of the three spots, we made lower-resolution (10 × 10 μm) and higher-resolution (1 × 1 μm) images, in order to analyze roughness changes at micron- and sub-micron levels. The AFM images were further processed and RMS (root mean squared) roughness values for each image were calculated with the JPK Data Processing software (version spm-5.1.8., JPK).
2.6
Contact angle measurements
Surface hydrophobicity/hydrophilicity of the specimens was assessed by contact angle measurements. Low contact angle values correspond to high surface hydrophilicity, and vice versa. For this purpose, we used the sessile drop method measured by the CAM 200 instrument (KSV NIMA-Biolin Scientific, Stockholm, Sweden) at room temperature. One droplet of ultrapure water (Milli-Q ® ) was applied on each of the six specimens in every group (n = 6). For each droplet, the average value of ten successive contact angle measurements (1 s intervals) was used.
2.7
Statistical analysis
All statistical analyses were performed by R (version 3.1.1; R Foundation for Statistical Computing). Two-way ANOVA compared the total amount of bacteria and the amount of S. mutans among different groups, whereas the composition of mixed biofilms between two composites was compared with one-way ANOVA. For the comparison of RMS roughness values among the different groups and between the composites, two-way ANOVA and Tukey’s post-hoc test were used. Finally, contact angle data were analyzed by the non-parametric Kruskal-Wallis and post-hoc Nemenyi multiple comparisons test. Normality of the data was tested with Shapiro-Wilk normality test, and all the tests were performed at a significance level of p = 0.05.
2
Materials and methods
2.1
Specimen preparation
Disk-shaped specimens (2-mm thickness, 7-mm diameter) of a hybrid, Bis-GMA-free composite (Gradia Direct Anterior, GC, Tokyo, Japan) and a flowable, Bis-GMA containing composite (Tetric EvoFlow, Ivoclar-Vivadent, Schaan, Lichtenstein) were prepared using polytetrafluoroethylene molds ( Table 1 ). The composites were inserted into molds, pressed between two glass slides and cured for 40 s at each side with a polywave LED unit as per manufacturers’ instructions (output: 1400 mW/cm 2 ) (Bluephase, Ivoclar-Vivadent, Schaan, Lichtenstein), in order to obtain very flat and clean surfaces. The surface that was not planned to be exposed to biofilms was marked with “X” with a razor blade. The specimens were then cleaned ultrasonically in distilled water for 5 min, after which they were gas-sterilized with ethylene oxide (EtO) at 38 °C.
Material | Composition | Manufacturer |
---|---|---|
Gradia Direct Anterior | Conventional hybrid composite Resin (27%w): Urethane dimethacrylate (UDMA), dimethacrylates, trimethacrylates Fillers: Silica (38%w), Pre-polymerised resin fillers (35%w) |
GC, Tokyo, Japan |
Tetric EvoFlow | Flowable nano-hybrid composite Resin (37.6%w): Bisphenol A-glycidyl methacrylate (Bis-GMA), Urethane dimethacrylate (UDMA), Decandioldimethacrylate Fillers: Barium glass filler, Ytterbium trifluoride, Mixed oxide, Highly dispersed silica (41.1%w) Prepolymers (20.4%w) |
Ivoclar Vivadent, Schaan, Lichtenstein |
2.2
Bacteria and culturing conditions
Overnight cultures of Streptococcus mutans (ATCC 25175), Streptococcus sanguinis (LMG 14657), Actinomyces naeslundii (ATCC 51655) and Fusobacterium nucleatum (ATCC 10953) were centrifuged and resuspended in fresh Brain Heart Infusion broth (BHI, Becton Dickinson and Company, Franklin Lakes, NJ, USA). The concentration of each bacterial suspension was adjusted spectrophotometrically (600 nm) (GeneQuant 100, GE Healthcare, UK) to 2 × 10 7 CFU/ml. Sterile material specimens were placed in 24-well plates (one specimen per well), with the “X”-sides facing the bottom of the wells, and the smooth upper surfaces available for biofilm formation. In order to form single-species biofilms, we added 2 ml of S. mutans suspension into the wells with specimens, while 500 μl of suspensions of each of the four species ( S. mutans , S. sanguinis , A. naeslundii and F. nucleatum ) were added to the wells to form multi-species biofilms. As negative controls, specimens incubated with 2 ml of sterile BHI broth, as well as unexposed (baseline) specimens, were used. Specimens with S. mutans cultures and with sterile BHI broth were incubated (37 °C, 5% CO 2 ) under aerobic conditions, while the specimens exposed to multi-species suspension were cultured anaerobically (85% N 2 , 10% CO 2 , 5% H 2 ) for a period of 6 weeks. Bacterial growth medium was refreshed every 48 h, without disturbing the formed biofilm. As positive controls, we used composite samples exposed to acidified sterile BHI broth (acidic control) and to an enzyme solution of cholesterol esterase for the same period of time. The pH of BHI broth was adjusted to 5 by adding lactic acid (Sigma Aldrich, St. Louis, MO, USA), whereas the enzyme solution was prepared by dissolving cholesterol esterase enzyme (CE, Sigma Aldrich, St. Louis, MO, USA) in phosphate buffered saline (PBS) at the concentration of 0.5 U/ml, and was replaced every 48 h by fresh stock solution kept at −20 °C. The experiments were carried out in triplicate, and were repeated two independent times on different days, with new bacterial cultures and specimens.
2.3
Scanning electron microscopy of biofilms
Additional composite disk-shaped specimens with a deep notch going through the middle of the disks, were prepared and exposed to bacterial cultures as described in §2.2. After an incubation of 6 weeks, the specimens with attached biofilms were carefully rinsed twice with sterile PBS and fixed with 2.5% glutaraldehyde in 0.1 M sodium-cacodylate buffer, followed by a postfixation treatment with 1% osmium-tetroxide. After the specimens were dehydrated in ethanol solutions with increasing concentration (50, 70, 90 and 100%) and dried with hexamethyldisilazane (Acros Organics, Geel, Belgium), they were carefully broken into halves at the notch in order allow the observation of the longitudinal sections of the biofilms. The specimens were then mounted on specimen stubs, sputter-coated with gold, and analyzed with SEM (JSM-6610LV, JEOL, Tokyo, Japan).
2.4
Real-time PCR quantification
After six weeks of incubation, specimens with biofilms were carefully taken out of the wells with sterile tweezers, without disturbing the overlying biofilms, and rinsed twice in sterile PBS to remove loosely bound cells. Subsequently, specimens were incubated for 30 min (37 °C, 5% CO 2 ) with 0.05% trypsin-EDTA solution (Life Technologies, Paisley, UK) to loosen up the attached biofilm, after which they were sonicated for 5 min in 2 ml of PBS to completely detach bacterial cells from the specimens. Finally, 1 ml of the PBS containing bacterial cells from biofilms was collected in micro-centrifuge tubes and frozen until DNA extraction and real-time PCR quantification. Control samples were also incubated with 0.05% trypsin-EDTA solution, to exclude any possible effect of this solution on the specimen’s surface.
Bacterial DNA was extracted and purified using the QIAamp DNA Mini Kit (Qiagen, Hilden, Germany) following the protocol for gram-positive bacteria. Real-time PCR was performed using specific primers and probes (Eurogentec, Liège, Belgium) in a CFX96 Real-Time PCR System (BioRad, Hercules, CA, USA) ( Table 2 ). Standard curves for absolute quantification were constructed using serial dilutions of plasmid DNA. Real-time PCR assays were carried out in a total volume of 25 μl, consisting of 12.5 μl of 2x Master Mix (Eurogentec), 1 μl each of the forward primer, the reverse primer and the probe, and 5 μl of the DNA template. The thermal cycling protocol was as follows: 1 cycle at 50 °C for 2 min, 1 cycle at 95 °C for 10 min, followed by 45 cycles at 95 °C for 15 s and at 60 °C for 1 min.
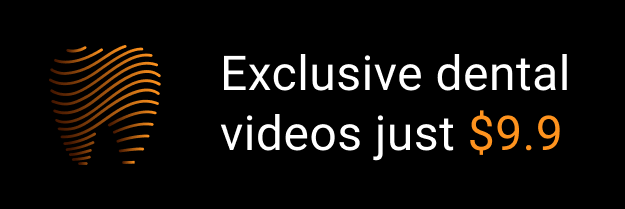