Abstract
Objectives
To elucidate the impact of surface properties and the release of fluoride from different glass ionomer cements on biofilm formation.
Methods
Standardized specimens manufactured from various classes of glass ionomer cements (GICs), a resin-based composite (RBC), and human enamel were subjected to surface analyses. Subsequent to simulation of salivary pellicle formation, Streptococcus mutans biofilm formation was initiated using a drip flow reactor for 48 h and 96 h. Biofilms were characterized by determining viable bacterial biomass and 3D biofilm architecture using SEM and CLSM; the release of fluoride from the specimens was measured using the ion selective micro method in dependence on various experimental conditions (incubation with sterile broth/bacteria/acid).
Results
Surface properties and biofilm formation correlated poorly, while the release of fluoride correlated well with viable streptococcal biomass and SEM/CLSM analyses. For all investigated materials, biofilm formation was lower than on enamel. The release of fluoride showed a significant dependency on the experimental conditions applied; the presence of biofilms reduced fluoride release in comparison to sterile incubation conditions.
Conclusions
Within the limitations of a laboratory study, the results suggest that biofilm formation on GICs cannot be easily predicted as a function of substratum surface parameters. The release of fluoride from glass ionomer cements contributes to control biofilm formation particularly in its early phases.
Clinical significance
Glass ionomer cements can actively control microbial biofilm formation, while biofilms modulate the release of fluoride from GIC materials.
1
Introduction
Glass ionomer cements (GICs) have been introduced in dentistry in the mid-seventies , featuring some favourable properties such as a chemical bond to enamel and dentin, a coefficient of thermal expansion almost equal to natural tooth tissues, and the ability to release fluoride over a significant amount of time . As a result, GICs are considered as biomaterials that may prevent and inactivate dental caries and its progression. While early glass ionomer cement formulations featured poor mechanical properties, the recently introduced high-viscosity GICs (HV-GICs) featured significantly improved hardness and stress resistance in comparison to early and conventional GICs. In addition to their application as restorative materials, GICs can successfully be used for cementation of indirect restorations .
As secondary caries remains one of the most frequent reasons for failure of dental restorations , it has often been highlighted that biofilm formation on the surface of dental restorative materials may contribute to the establishment of secondary caries lesions. In GIC restorations, the fluoride released by GICs may serve as a buffer that neutralizes acids secreted by oral bacteria and may inhibit the growth of cariogenic microorganisms . Recent studies have highlighted a correlation between the release of fluoride from GICs and the properties of Streptococcus mutans biofilms on their surface, suggesting that acidogenicity, dry weight, the amount of extracellular polysaccharides (EPS), as well as biovolumes and EPS thickness are significantly reduced in biofilms on the surface of GICs which feature a high release of fluoride . Employing a clinical approach for the analysis of biofilm formation, other researchers pointed out that despite of lacking significant differences in biofilm formation between various materials such as GICs, amalgam, resin-based composite, and ceramic, there was a tendency towards a lower number of viable bacterial cells in biofilms grown on restorations made of GICs and amalgam . Similar results have been published by other groups , while some researchers suggested that biofilm formation is not necessarily reduced on fluoride-releasing GICs . Thus, the aim of the present laboratory study was to elucidate the impact of surface properties and the release of fluoride from various classes of GICs on Streptococcus mutans biofilm formation in dependency on incubation times. The study hypotheses were that (I) Streptococcus mutans biofilm formation is not affected by the release of fluoride, (II) the release of fluoride is not influenced by the incubation time, and (III) the release of fluoride is not influenced by exposure of GIC surfaces to biofilms.
2
Materials and method
2.1
Specimen preparation
Standardized specimens were prepared from an experimental light-curing glass ionomer cement (resin-modified GIC; A), a compomer ( Glasiosite ; B), a luting GIC ( Meron ; C), a high viscosity GIC ( Ionostar plus ; D), and from a reference nanohybrid resin-based composite (RBC, Grandioso ; E, all by VOCO GmbH, Cuxhaven, G). For preparation of a single specimen, a standardized amount of each material was placed into a custom made steel mould with a diameter of 6.0 mm and a height of 2.0 mm, condensed against a glass plate and covered with a cellulose acetate strip (Mylar ® ) until cured. Compomer, resin-modified GIC, and RBC specimens were light-cured in direct contact for 40 s using a hand-held light curing unit (LCU; SDI Radii plus, SDI, Bayswater, AUS; 1500 mW/cm 2 ). A total of 83 specimens for each material were produced. The specimens were then subjected to a standardized polishing protocol, including polishing with 1000/4000-grit grinding paper (Buehler, Lake Bluff, IL, USA) using a polishing machine (Motopol 8; Buehler, Düsseldorf, G).
Anterior human teeth extracted for clinical reasons were obtained from the Oral Surgery Unit at the Department of Biomedical, Surgical and Dental Sciences (Milan, Italy). A total of 83 round enamel-dentin slabs with a diameter of 6.0 mm and a thickness of 2.0 mm were cut from the labial surfaces using a water-cooled trephine diamond bur (INDIAM, Carrara, I). Dentin bottoms were removed, and the enamel surfaces (enamel, E) were polished as described for the dental materials above. All specimens were subsequently stored under light-proof conditions in artificial saliva for six days at 37 ± 1 °C prior to the further experiments to allow for maturation of the cement, to eliminate potential impacts of a fluoride burst and, for the resin-modified GIC, the compomer, and RBC, to minimize the impact of residual monomer leakage on cell viability. The artificial saliva used in the present study allows a reproduction of the average electrolytic composition of human whole saliva and was prepared by mixing 100 mL of 150 mM KHCO 3 , 100 mL of 100 mM NaCl, 100 mL of 25 mM K 2 HPO 4 , 100 mL of 24 mM Na 2 HPO 4 , 100 mL of 15 mM CaCl 2 , 100 mL of 1,5 mM MgCl 2 , and 6 mL of 25 mM citric acid. The volume was made up to 1 L and the pH was adjusted to 7.0 by pipetting NaOH 4 M or HCl 4 M solutions under vigorous stirring. All specimens undergoing surface analysis were subsequently cleaned using distilled water and applicator brush tips (3 M ESPE, Seefeld, G).
2.2
Surface analysis
2.2.1
Surface roughness
Peak-to-valley surface roughness (R a ) was determined on five randomly selected specimens for each group material using a profilometric contact surface measurement device (Perthen S6P, Feinprüf-Perthen, Göttingen, G). A distance of 1.75 mm was measured in three randomly selected line scans perpendicular to the expected grinding grooves using a standard diamond tip (tip radius 2 μm, tip angle 90°) and a cut off level of 0.25.
2.2.2
Surface free energy
Contact angles between the surface of the various materials and three liquids differing in hydrophobicity (bidistilled water, diiodomethane, ethylene glycol) were determined using the sessile drop method and a computer-aided contact angle measurement device (OCA 15plus, DataPhysics Instruments GmbH, Filderstadt, G). A total of eight drops for each liquid (drop volume 0.2 μL) were analyzed on each of four randomly selected specimens for each material. Left and right contact angles were averaged and the surface free energy was calculated according to the approach introduced by Owens and Wendt .
2.2.3
Energy-dispersive X-ray spectroscopy (EDS)
Two randomly selected specimens for each material were subjected to EDS surface analysis. A scanning electron microscope coupled with an EDS probe (EDAX Genesis 2000, Ametek GmbH, Meerbusch, G) was used to acquire full frames of the surfaces of the specimens employing an accelerating voltage of 20 kV, a magnification of 2000× and an acquisition time of 200 s.
2.3
Microbiological procedures
2.3.1
Saliva preparation
Stimulated whole saliva was collected by expectoration from three healthy donors in accordance with the protocol published by Guggenheim et al. . Saliva was collected in chilled tubes, pooled, heated to 60 °C for 30 min to inactivate endogenous enzymes, and was then centrifuged (12.000 × g ) for 15 min at 4 °C. The supernatant was transferred into sterile tubes, stored at −20 °C, and thawed at 37 °C for 1 h directly prior to the experiments.
2.3.2
Bacteria
Streptococcus mutans ATCC 35668 was cultured according to a previously published protocol . Briefly, Mitis Salivarius Bacitracin agar inoculated plates were incubated for 48 h at 37 °C in a 5% supplemented CO 2 environment. A total of 1% sucrose was added to a pure suspension of the microorganism in Brain Heart Infusion obtained from these plates after an incubation of 12 h at 37 °C in a 5% supplemented CO 2 environment. S. mutans cells were harvested by centrifugation (2200 rpm, 19 °C, 5 min), washed twice with phosphate-buffered saline (PBS), and resuspended. The suspension was subsequently subjected to low intensity ultrasonic energy (Sonifier model B-150; Branson, Danbury, CT, USA; operating at 7-W energy output for 30 s) in order to disperse bacterial chains. The suspension was then adjusted to a value of 1.0 on the McFarland scale, corresponding to a microbial concentration of approximately 3.0 × 10 8 cells/mL.
2.3.3
Biofilm formation
The drip-flow reactor (M-DFR) employed in the present study was a modification of a commercially available Drip Flow Reactor (DFR 110; BioSurface Technologies, Bozeman, MT, USA). The modified design allowed the placement of customized specimen-trays on the bottom of the flow cells and the complete immersion of the surfaces of the specimens into the surrounding flowing medium . A total of 72 randomly selected specimens for each material were placed in Teflon trays, which fixed the specimens tightly and exposed their surfaces to the surrounding medium; the trays were fixed on the bottom of each flow cell of the M-DFR. All tubing and specimen-containing trays were sterilized prior to the experiments using a chemiclave with hydrogen peroxide gas plasma technology (Sterrad; ASP, Irvine, CA, USA). By limiting the maximum temperature to 45 °C, heat-related damage of the specimens was avoided. The whole M-DFR was assembled inside a sterile hood and transferred into a thermostat in order to operate under a standardized temperature of 37 °C.
For simulation of salivary pellicle formation, the surfaces of the specimens in each flow cell were exposed to thawed sterile saliva for 24 h. Subsequently, excess saliva was discarded. S. mutans monospecific biofilm development was obtained on the surfaces of the specimens by inoculating 10 mL of S. mutans suspension into each flow cell.
A total of 40 specimens for each material were inoculated with S. mutans suspension, 16 specimens were incubated under sterile conditions and 16 additional specimens were incubated under acidic conditions (pH = 4.0) generated by a flow of buffered lactic acid/potassium lactate solution. After 4 h, a multichannel, computer-controlled peristaltic pump (RP-1; Rainin, Emeryville, CA, USA) was turned on to provide a constant flow of nutrient broth through the flow cells. The sterile nutrient broth was enriched with 10.0 g/L sucrose and consisted of 2.5 g/L mucin (type II, porcine gastric), 2.0 g/L bacteriological peptone, 2.0 g/L tryptone, 1.0 g/L yeast extract, 0.35 g/L NaCl, 0.2 g/L KCl, 0.2 g/L CaCl2, 0.1 g/L cysteine hydrochloride, 0.001 g/L hemin, and 0.0002 g/L vitamin K1. The flow rate was set to 9.6 mL/h .
2.3.4
MTT assay
Viable biomass adherent to the surfaces was assessed after either 48 h (n = 8) or 96 h (n = 8) incubation period using a MTT-based assay . In brief, MTT stock solution was prepared by dissolving 5 mg/mL 3-(4,5)-dimethylthiazol-2-yl-2,5-diphenyltetrazolium bromide in sterile PBS; PMS stock solution was prepared by dissolving 0.3 mg/mL of N-methylphenazinium methyl sulphate in sterile PBS. The solutions were stored at 2 °C in light-proof vials until the day of the experiment, when a fresh measurement solution (FMS) was prepared by diluting 1:10 v/v of MTT stock solution and 1:10 v/v of PMS stock solution in sterile PBS. A lysing solution (LS) was prepared by dissolving 10% v/v of sodium dodecyl sulphate and 50% v/v dimethylformamide in deionized water.
After either 48 h or 96 h of incubation in the M-DFR, the flow of nutrient broth was stopped, the flow cells were opened, and the trays containing the specimens were carefully removed and immediately placed in dishes containing sterile PBS at 37 °C. The specimens were subsequently gently removed from the tray, passed into another dish with sterile PBS at 37 °C in order to remove non-adhered cells, and finally transferred into 48-well plates. 300 μL of FMS solution were added to each well, and the plates were incubated for 3 h at 37 °C under light-proof conditions.
During incubation, electron transport across the microbial plasma membrane and, to a lesser extent, microbial redox systems convert the yellow salt to insoluble purple formazan crystals. The conversion at the cell membrane level was facilitated by the intermediate electron acceptor (PMS). The unreacted FMS solution was gently removed and the formazan crystals were dissolved by adding 300 μL of LS to each well. The plates were stored for an additional 1 h under light-proof conditions at room temperature; 100 μL of the solution were then transferred into the wells of 96-well plates. The absorbance of the solution was measured using a spectrophotometer (Genesys 10-S, Thermo Spectronic, Rochester, NY, USA) at a wavelength of 550 nm; results were expressed as relative absorbance in optical density (OD) units corresponding to the amount of adherent, viable and metabolically active biomass.
2.3.5
Confocal laser-scanning microscopy
A total of four specimens (48 h incubation, n = 2; 96 h incubation, n = 2) were gently removed from the flow cells, rinsed twice with sterile PBS, and stained using the FilmTracer™ LIVE/DEAD ® Biofilm Viability Kit (Invitrogen Ltd., Paisley, UK). Stained biofilms were analyzed using confocal laser-scanning microscopy (Leica TCS SP2, Leica Microsystems, Wetzlar, G) immediately after the staining procedures. Four randomly selected image stack sections were recorded for each specimen and incubation time. Confocal images were obtained using a dry 20× (NA = 0.7) objective and digitalized using the Leica Application Suite Advanced Fluorescence Software (LAS-AF, Leica microsystems) at a resolution of 2048 × 2048 pixels with a zoom factor of 1.0 at a scan speed of 400 Hz. Three channels were acquired: one was excited at 405 nm and observed at 420–470 nm in order to digitally subtract in post-processing the specimenś autofluorescence signal whenever present; the other two channels were excited at a wavelength of 488 nm. Emission was acquired at 500–570 nm (green channel, live bacteria) and 610–760 nm (red channel, dead bacteria). For each image stack section, 3D-rendering reconstructions were obtained using ImageJ (National Institutes of Health, Bethesda, MD, USA), and Drishti (Ajay Limaye, Australian National University, CAN, AUS http://sf.anu.edu.au/Vizlab/drishti/ ) was used for 3D reconstructions.
2.3.6
Scanning electron microscopy
A total of four specimens (48 h incubation, n = 2; 96 h incubation, n = 2) were gently removed from the flow cells, rinsed twice with sterile PBS, and placed into a cacodylate-buffered 2% glutaraldehyde–fixative solution (pH = 7.4) for 48 h. The specimens were then passed through a graded ethanol series (50, 70, 80, 85, 90, 95, and 100%, v/v). Finally, the specimens were subjected to critical point drying (Critical-Point Dryer, EMS 850, Hatfield, PA, USA), mounted on stubs with conductive glue, sputter coated (JEOL FFC-1100, Japan), and analyzed with a scanning electron microscope (JEOL JSM-5300, Japan) at a magnification of 500x–5000x. Four randomly selected fields (2000×) were recorded for each specimen.
2.4
Determination of the release of fluoride
The release of fluoride from each material was determined for specimens subjected to biofilm formation (G1; 48 h (n = 8) or 96 h (n = 8)) for specimens kept under sterile flow conditions (G2; 48 h (n = 8) or 96 h (n = 8)), and for specimens kept under the acidic buffered solution flow (G3; 48 h (n = 8) or 96 h (n = 8)). The latter condition simulated a continuous acidic environment as expected in biofilms with low pH value. Specimens were removed from the flow cells and adherent biomass (for group G1 only) was detached by sonication in distilled water in an ultrasonic bath for 5 min. The specimens (G1, G2, and G3) were immersed into 400 μL of fresh artificial saliva and the cumulative concentration of fluoride in the supernatant was determined after a period of seven days using the ion-selective electrode micro method as described previously . In brief, a stock solution with a fluoride concentration of 1000 ppm was appropriately diluted with artificial saliva to obtain fluoride standards with fluoride concentrations ranging from 0.0019 to 64 parts per million (ppm). A calibration curve was obtained by measuring fluoride standards using a digital pH/mV meter (SA-720, Orion Research Inc, Boston, MA, USA). Sodium acetate buffer 20% v/v with EDTA as ionic strength adjustor was added to each standard prior to analysis. A negative standard (0 ppm fluoride) was prepared by adding 20% v/v of sodium acetate buffer with EDTA to artificial saliva; this solution was also used to rinse the electrodes between the single measurements.
2.5
Statistical analysis
Statistical analyses were performed using JMP 10.0 software (SAS Institute, Cary, NC, USA). Normal distribution of data was checked using Shapiro-Wilk’s test and homogeneity of variances was verified using Levene’s test. Means and standard errors were calculated from the raw data. One-way analysis of variance (ANOVA) was employed to analyze data gathered for surface characterization. Biofilm formation (MTT assay) was analysed using two-way ANOVA setting material and incubation time as fixed factors. Release of fluoride was statistically examined using three-way ANOVA setting material, incubation time, and incubation mode as fixed factors. Tukey HSD test was employed for post-hoc analysis (p < 0.05).
2
Materials and method
2.1
Specimen preparation
Standardized specimens were prepared from an experimental light-curing glass ionomer cement (resin-modified GIC; A), a compomer ( Glasiosite ; B), a luting GIC ( Meron ; C), a high viscosity GIC ( Ionostar plus ; D), and from a reference nanohybrid resin-based composite (RBC, Grandioso ; E, all by VOCO GmbH, Cuxhaven, G). For preparation of a single specimen, a standardized amount of each material was placed into a custom made steel mould with a diameter of 6.0 mm and a height of 2.0 mm, condensed against a glass plate and covered with a cellulose acetate strip (Mylar ® ) until cured. Compomer, resin-modified GIC, and RBC specimens were light-cured in direct contact for 40 s using a hand-held light curing unit (LCU; SDI Radii plus, SDI, Bayswater, AUS; 1500 mW/cm 2 ). A total of 83 specimens for each material were produced. The specimens were then subjected to a standardized polishing protocol, including polishing with 1000/4000-grit grinding paper (Buehler, Lake Bluff, IL, USA) using a polishing machine (Motopol 8; Buehler, Düsseldorf, G).
Anterior human teeth extracted for clinical reasons were obtained from the Oral Surgery Unit at the Department of Biomedical, Surgical and Dental Sciences (Milan, Italy). A total of 83 round enamel-dentin slabs with a diameter of 6.0 mm and a thickness of 2.0 mm were cut from the labial surfaces using a water-cooled trephine diamond bur (INDIAM, Carrara, I). Dentin bottoms were removed, and the enamel surfaces (enamel, E) were polished as described for the dental materials above. All specimens were subsequently stored under light-proof conditions in artificial saliva for six days at 37 ± 1 °C prior to the further experiments to allow for maturation of the cement, to eliminate potential impacts of a fluoride burst and, for the resin-modified GIC, the compomer, and RBC, to minimize the impact of residual monomer leakage on cell viability. The artificial saliva used in the present study allows a reproduction of the average electrolytic composition of human whole saliva and was prepared by mixing 100 mL of 150 mM KHCO 3 , 100 mL of 100 mM NaCl, 100 mL of 25 mM K 2 HPO 4 , 100 mL of 24 mM Na 2 HPO 4 , 100 mL of 15 mM CaCl 2 , 100 mL of 1,5 mM MgCl 2 , and 6 mL of 25 mM citric acid. The volume was made up to 1 L and the pH was adjusted to 7.0 by pipetting NaOH 4 M or HCl 4 M solutions under vigorous stirring. All specimens undergoing surface analysis were subsequently cleaned using distilled water and applicator brush tips (3 M ESPE, Seefeld, G).
2.2
Surface analysis
2.2.1
Surface roughness
Peak-to-valley surface roughness (R a ) was determined on five randomly selected specimens for each group material using a profilometric contact surface measurement device (Perthen S6P, Feinprüf-Perthen, Göttingen, G). A distance of 1.75 mm was measured in three randomly selected line scans perpendicular to the expected grinding grooves using a standard diamond tip (tip radius 2 μm, tip angle 90°) and a cut off level of 0.25.
2.2.2
Surface free energy
Contact angles between the surface of the various materials and three liquids differing in hydrophobicity (bidistilled water, diiodomethane, ethylene glycol) were determined using the sessile drop method and a computer-aided contact angle measurement device (OCA 15plus, DataPhysics Instruments GmbH, Filderstadt, G). A total of eight drops for each liquid (drop volume 0.2 μL) were analyzed on each of four randomly selected specimens for each material. Left and right contact angles were averaged and the surface free energy was calculated according to the approach introduced by Owens and Wendt .
2.2.3
Energy-dispersive X-ray spectroscopy (EDS)
Two randomly selected specimens for each material were subjected to EDS surface analysis. A scanning electron microscope coupled with an EDS probe (EDAX Genesis 2000, Ametek GmbH, Meerbusch, G) was used to acquire full frames of the surfaces of the specimens employing an accelerating voltage of 20 kV, a magnification of 2000× and an acquisition time of 200 s.
2.3
Microbiological procedures
2.3.1
Saliva preparation
Stimulated whole saliva was collected by expectoration from three healthy donors in accordance with the protocol published by Guggenheim et al. . Saliva was collected in chilled tubes, pooled, heated to 60 °C for 30 min to inactivate endogenous enzymes, and was then centrifuged (12.000 × g ) for 15 min at 4 °C. The supernatant was transferred into sterile tubes, stored at −20 °C, and thawed at 37 °C for 1 h directly prior to the experiments.
2.3.2
Bacteria
Streptococcus mutans ATCC 35668 was cultured according to a previously published protocol . Briefly, Mitis Salivarius Bacitracin agar inoculated plates were incubated for 48 h at 37 °C in a 5% supplemented CO 2 environment. A total of 1% sucrose was added to a pure suspension of the microorganism in Brain Heart Infusion obtained from these plates after an incubation of 12 h at 37 °C in a 5% supplemented CO 2 environment. S. mutans cells were harvested by centrifugation (2200 rpm, 19 °C, 5 min), washed twice with phosphate-buffered saline (PBS), and resuspended. The suspension was subsequently subjected to low intensity ultrasonic energy (Sonifier model B-150; Branson, Danbury, CT, USA; operating at 7-W energy output for 30 s) in order to disperse bacterial chains. The suspension was then adjusted to a value of 1.0 on the McFarland scale, corresponding to a microbial concentration of approximately 3.0 × 10 8 cells/mL.
2.3.3
Biofilm formation
The drip-flow reactor (M-DFR) employed in the present study was a modification of a commercially available Drip Flow Reactor (DFR 110; BioSurface Technologies, Bozeman, MT, USA). The modified design allowed the placement of customized specimen-trays on the bottom of the flow cells and the complete immersion of the surfaces of the specimens into the surrounding flowing medium . A total of 72 randomly selected specimens for each material were placed in Teflon trays, which fixed the specimens tightly and exposed their surfaces to the surrounding medium; the trays were fixed on the bottom of each flow cell of the M-DFR. All tubing and specimen-containing trays were sterilized prior to the experiments using a chemiclave with hydrogen peroxide gas plasma technology (Sterrad; ASP, Irvine, CA, USA). By limiting the maximum temperature to 45 °C, heat-related damage of the specimens was avoided. The whole M-DFR was assembled inside a sterile hood and transferred into a thermostat in order to operate under a standardized temperature of 37 °C.
For simulation of salivary pellicle formation, the surfaces of the specimens in each flow cell were exposed to thawed sterile saliva for 24 h. Subsequently, excess saliva was discarded. S. mutans monospecific biofilm development was obtained on the surfaces of the specimens by inoculating 10 mL of S. mutans suspension into each flow cell.
A total of 40 specimens for each material were inoculated with S. mutans suspension, 16 specimens were incubated under sterile conditions and 16 additional specimens were incubated under acidic conditions (pH = 4.0) generated by a flow of buffered lactic acid/potassium lactate solution. After 4 h, a multichannel, computer-controlled peristaltic pump (RP-1; Rainin, Emeryville, CA, USA) was turned on to provide a constant flow of nutrient broth through the flow cells. The sterile nutrient broth was enriched with 10.0 g/L sucrose and consisted of 2.5 g/L mucin (type II, porcine gastric), 2.0 g/L bacteriological peptone, 2.0 g/L tryptone, 1.0 g/L yeast extract, 0.35 g/L NaCl, 0.2 g/L KCl, 0.2 g/L CaCl2, 0.1 g/L cysteine hydrochloride, 0.001 g/L hemin, and 0.0002 g/L vitamin K1. The flow rate was set to 9.6 mL/h .
2.3.4
MTT assay
Viable biomass adherent to the surfaces was assessed after either 48 h (n = 8) or 96 h (n = 8) incubation period using a MTT-based assay . In brief, MTT stock solution was prepared by dissolving 5 mg/mL 3-(4,5)-dimethylthiazol-2-yl-2,5-diphenyltetrazolium bromide in sterile PBS; PMS stock solution was prepared by dissolving 0.3 mg/mL of N-methylphenazinium methyl sulphate in sterile PBS. The solutions were stored at 2 °C in light-proof vials until the day of the experiment, when a fresh measurement solution (FMS) was prepared by diluting 1:10 v/v of MTT stock solution and 1:10 v/v of PMS stock solution in sterile PBS. A lysing solution (LS) was prepared by dissolving 10% v/v of sodium dodecyl sulphate and 50% v/v dimethylformamide in deionized water.
After either 48 h or 96 h of incubation in the M-DFR, the flow of nutrient broth was stopped, the flow cells were opened, and the trays containing the specimens were carefully removed and immediately placed in dishes containing sterile PBS at 37 °C. The specimens were subsequently gently removed from the tray, passed into another dish with sterile PBS at 37 °C in order to remove non-adhered cells, and finally transferred into 48-well plates. 300 μL of FMS solution were added to each well, and the plates were incubated for 3 h at 37 °C under light-proof conditions.
During incubation, electron transport across the microbial plasma membrane and, to a lesser extent, microbial redox systems convert the yellow salt to insoluble purple formazan crystals. The conversion at the cell membrane level was facilitated by the intermediate electron acceptor (PMS). The unreacted FMS solution was gently removed and the formazan crystals were dissolved by adding 300 μL of LS to each well. The plates were stored for an additional 1 h under light-proof conditions at room temperature; 100 μL of the solution were then transferred into the wells of 96-well plates. The absorbance of the solution was measured using a spectrophotometer (Genesys 10-S, Thermo Spectronic, Rochester, NY, USA) at a wavelength of 550 nm; results were expressed as relative absorbance in optical density (OD) units corresponding to the amount of adherent, viable and metabolically active biomass.
2.3.5
Confocal laser-scanning microscopy
A total of four specimens (48 h incubation, n = 2; 96 h incubation, n = 2) were gently removed from the flow cells, rinsed twice with sterile PBS, and stained using the FilmTracer™ LIVE/DEAD ® Biofilm Viability Kit (Invitrogen Ltd., Paisley, UK). Stained biofilms were analyzed using confocal laser-scanning microscopy (Leica TCS SP2, Leica Microsystems, Wetzlar, G) immediately after the staining procedures. Four randomly selected image stack sections were recorded for each specimen and incubation time. Confocal images were obtained using a dry 20× (NA = 0.7) objective and digitalized using the Leica Application Suite Advanced Fluorescence Software (LAS-AF, Leica microsystems) at a resolution of 2048 × 2048 pixels with a zoom factor of 1.0 at a scan speed of 400 Hz. Three channels were acquired: one was excited at 405 nm and observed at 420–470 nm in order to digitally subtract in post-processing the specimenś autofluorescence signal whenever present; the other two channels were excited at a wavelength of 488 nm. Emission was acquired at 500–570 nm (green channel, live bacteria) and 610–760 nm (red channel, dead bacteria). For each image stack section, 3D-rendering reconstructions were obtained using ImageJ (National Institutes of Health, Bethesda, MD, USA), and Drishti (Ajay Limaye, Australian National University, CAN, AUS http://sf.anu.edu.au/Vizlab/drishti/ ) was used for 3D reconstructions.
2.3.6
Scanning electron microscopy
A total of four specimens (48 h incubation, n = 2; 96 h incubation, n = 2) were gently removed from the flow cells, rinsed twice with sterile PBS, and placed into a cacodylate-buffered 2% glutaraldehyde–fixative solution (pH = 7.4) for 48 h. The specimens were then passed through a graded ethanol series (50, 70, 80, 85, 90, 95, and 100%, v/v). Finally, the specimens were subjected to critical point drying (Critical-Point Dryer, EMS 850, Hatfield, PA, USA), mounted on stubs with conductive glue, sputter coated (JEOL FFC-1100, Japan), and analyzed with a scanning electron microscope (JEOL JSM-5300, Japan) at a magnification of 500x–5000x. Four randomly selected fields (2000×) were recorded for each specimen.
2.4
Determination of the release of fluoride
The release of fluoride from each material was determined for specimens subjected to biofilm formation (G1; 48 h (n = 8) or 96 h (n = 8)) for specimens kept under sterile flow conditions (G2; 48 h (n = 8) or 96 h (n = 8)), and for specimens kept under the acidic buffered solution flow (G3; 48 h (n = 8) or 96 h (n = 8)). The latter condition simulated a continuous acidic environment as expected in biofilms with low pH value. Specimens were removed from the flow cells and adherent biomass (for group G1 only) was detached by sonication in distilled water in an ultrasonic bath for 5 min. The specimens (G1, G2, and G3) were immersed into 400 μL of fresh artificial saliva and the cumulative concentration of fluoride in the supernatant was determined after a period of seven days using the ion-selective electrode micro method as described previously . In brief, a stock solution with a fluoride concentration of 1000 ppm was appropriately diluted with artificial saliva to obtain fluoride standards with fluoride concentrations ranging from 0.0019 to 64 parts per million (ppm). A calibration curve was obtained by measuring fluoride standards using a digital pH/mV meter (SA-720, Orion Research Inc, Boston, MA, USA). Sodium acetate buffer 20% v/v with EDTA as ionic strength adjustor was added to each standard prior to analysis. A negative standard (0 ppm fluoride) was prepared by adding 20% v/v of sodium acetate buffer with EDTA to artificial saliva; this solution was also used to rinse the electrodes between the single measurements.
2.5
Statistical analysis
Statistical analyses were performed using JMP 10.0 software (SAS Institute, Cary, NC, USA). Normal distribution of data was checked using Shapiro-Wilk’s test and homogeneity of variances was verified using Levene’s test. Means and standard errors were calculated from the raw data. One-way analysis of variance (ANOVA) was employed to analyze data gathered for surface characterization. Biofilm formation (MTT assay) was analysed using two-way ANOVA setting material and incubation time as fixed factors. Release of fluoride was statistically examined using three-way ANOVA setting material, incubation time, and incubation mode as fixed factors. Tukey HSD test was employed for post-hoc analysis (p < 0.05).
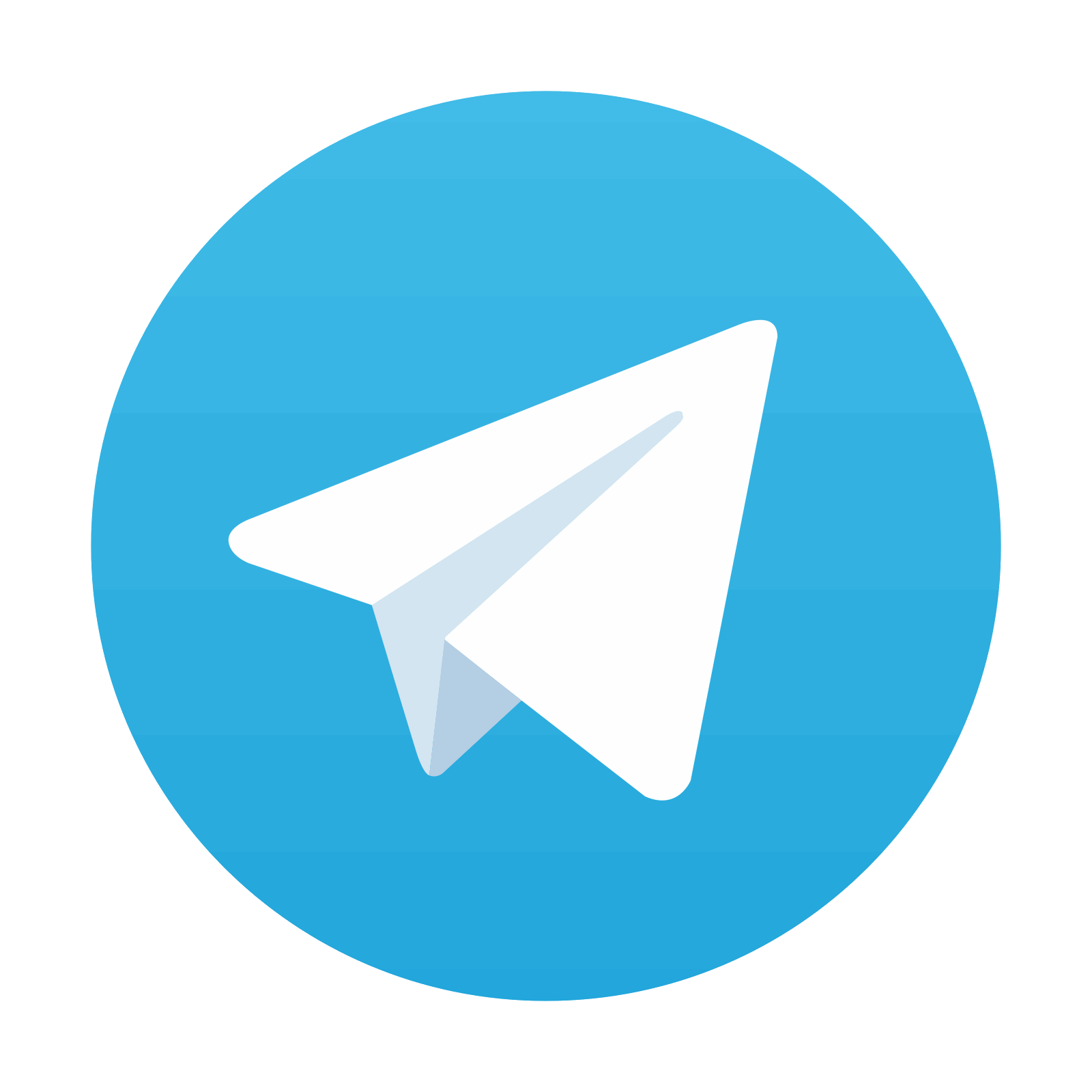
Stay updated, free dental videos. Join our Telegram channel

VIDEdental - Online dental courses
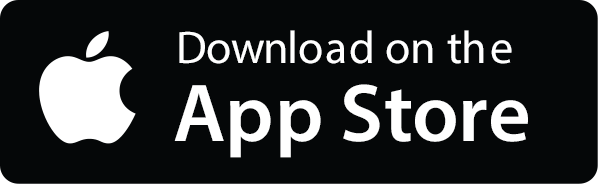
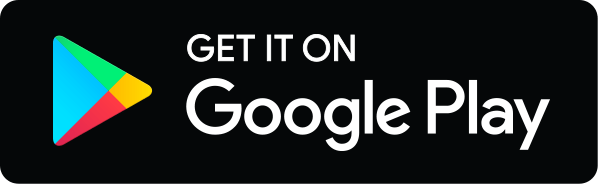