Abstract
Objectives
Dental personnel are more at risk to develop asthmatic disease, but the exact reason is so far unknown. During abrasive procedures, dental personnel are exposed to nano-sized dust particles released from dental composite. The aim of this study was to investigate whether respirable composite dust may also release monomers.
Methods
Respirable (<5 μm) composite dust was collected and the release of methacrylate monomers and Bisphenol A (BPA) in water and ethanol was evaluated by liquid chromatography/mass spectroscopy (LC–MS/MS). The dust was ultra-morphologically and chemically analyzed by transmission electron microscopy and energy-dispersive X-ray spectroscopy (TEM-EDS).
Results
LC–MS/MS analysis revealed that, irrespective of the type of composite, the respirable fraction of composite dust may release relatively high concentrations of unpolymerized methacrylate monomers, both in water and ethanol. Higher release was observed in ethanol. The endocrine disruptor BPA also emanated from the composite dust particles. TEM showed that most particles were nano-sized, although particle size ranged between 6 nm and 5 μm with a mode value between 12 and 39 nm. Most particles consisted of several filler particles in resin matrix, although single nano-filler particles could also be observed. Elemental analysis by TEM-EDS proved that the particles collected on the filters originated from the dental composites.
Conclusion
Theoretically, composite dust may function as a vehicle to transport monomers deeply into the respiratory system. The results of this study may shed another light on the increasing incidence of respiratory disease among dental personnel, and more care should be taken to prevent inhalation of composite dust.
Clinical significance
Special care should be taken to prevent inhalation of composite dust, as the dust particles may release methacrylate monomers.
1
Introduction
Engineered nanoparticles used for biomedical purposes are often coated (or functionalized) for different reasons. By changing the surface properties of the particles, the inherent surface reactivity of the particles can be reduced, toxicity can be mitigated, agglomeration can be prevented and uptake, both cellular and gastro-intestinal can be improved . Synthetic polymers, such as polyethylene glycol or polyacrylic acid are often used to coat particles thanks to their stability and good biocompatibility .
An example of unintentional exposure to polymer-coated (nano-) particles is the exposure of dental personnel and patients to airborne dust particles from dental composite, which typically occurs during the placement of this type of fillings. Previous research revealed that during these finishing steps, high amounts (particle concentrations between 10 5 and 10 6 #/cm 3 ) of nano-sized particles (<100 nm) were released in the breathing zone of both dental personnel and patient . Transmission electron microscopic analysis showed that composite dust consisted mostly of nano-sized pieces of filler particles coated in resin, but also single nano-sized filler particles and pieces of resin could be observed .
First toxicological research showed that composite dust does not exhibit important surface reactivity or intrinsic oxidative potential , which is in correspondence with previous research showing that metal oxides nanoparticles such as silica and amorphous silica exhibit rather low toxicity. Also the fact that the dust particles are often still covered by methacrylate resin may play a role. However, knowing that the methacrylate resin in situ never polymerizes to a full extent (polymerization degree is typically between 50 and 60%), and that unpolymerized monomers, are released in the oral cavity from composite restorations, it could be hypothesized that composite dust also could release residual monomers . There have already been reports on increasing occupational hypersensitivity in dental personnel , but so far it has not been entirely clear which components may be responsible for this increase. It has been well established that methacrylate monomers are allergenic and can provoke allergic reactions , but it has never been investigated whether airborne composite dust, which is small enough to enter deeply into the lungs, plays a role in respiratory hypersensitization in dental personnel. In this study, the potential of composite dust to release unpolymerized monomers was investigated. The null hypothesis to be tested was that composite dust does not emanate methacrylate monomers.
2
Materials and methods
2.1
Composites used
Four commercial resin-based composites, including so-called nano(-hybrid) composites and conventional hybrid composites were included in this study. Their composition can be found in Table 1 . For each composite, five sticks with size of 17.4 mm × 5.4 mm × 1.6 mm were prepared in a metal mold following a clinically relevant protocol as per instructions of the manufacturer. The composite was covered with a glass plate, and then light-cured for 20 s with a light curing unit (Bluephase 20i, Ivoclar-Vivadent, Schaan, Liechtenstein) with an output between 1000 and 1100 mW/cm 2 and wavelength 385–515 nm.
Composite | Manufacturer | Classification | Resin matrix | Filler | Filler loading |
---|---|---|---|---|---|
Filtek Supreme XTE | 3 M ESPE, Seefeld, Germany | Nano-filled composite | BisGMA, BisEMA, UDMA, TEGDMA |
|
78.5 wt% 59.5 vol% |
Z100 MP | 3 M ESPE, St. Paul, MN, USA | Hybrid composite | BisGMA, TEGDMA |
|
84.5 wt% 66 vol% |
Grandio | VOCO, Cuxhaven, Germany | Nano-hybrid composite | BisGMA, TEGDMA |
|
87 wt% 71.4 vol% |
Gradia Direct | GC, Tokyo, Japan | Micro-filled hybrid composite | UDMA |
|
73 wt% 64 vol% |
2.2
Collection of respirable dust
Similar to clinical circumstances, entire blocks of composite (n = 5) were ground in an enclosed chamber using a dental bur, and airborne respirable dust was collected using a cyclone. The cyclone (225-62, SKC, Eighty Four, PA, USA) was fixed to the wall of the chamber, and attached to a personal sampling pump (SP 350 sidepack personal sampling pump, TSI, Shoreview, MN, USA) ( Fig. 1 ) . The air flow of the pump (2.2 L/min) was calibrated with the Defender 510 (BIOS International Corp., Butler, NJ, USA). The cassette inside the cyclone contained a polytetrafluoroethylene (PTFE) filter (Mitex membrane filters, pore size 5.0 μm, Millipore LSWP02500, Billerica, MA, USA).
The experiment was carried out in an experimental chamber made of plexiglass (270 × 270 × 420 mm) with two openings at the sides sealed with disposable gloves, enabling manipulation of the specimens and the bur ( Fig. 1 ). The composite blocks were held with a forceps during grinding with a rough diamond bur (842314014 Komet, Lemgo, Germany, grain size 100 μm) in a Kavo Intracompact handpiece (200,000 rpm) connected to an electric micromotor (EWL K9, Kavo, Biberach, Germany). The motor was placed outside of the chamber to prevent particle emissions from the motor to mix with the sample aerosol. The pump was started immediately before grinding the composite, and left on for 10 min after grinding the composite (or until the pump blocked).
The amount of respirable dust collected on the filter was quantified gravimetrically measuring the weight of the Teflon filter before and after dust collection via micro balance (Sartorius 1712 MP 8, Sartorius AG, Goettingen, Germany).
2.3
Characterization of composite dust
The dust collected on the filters in the cyclone was characterized by transmission electron microscopy (TEM) and by energy dispersive spectroscopy (TEM-EDS). A formvar-coated copper grid was attached in the cassette in which the filter is normally placed and the procedure for respirable dust collection was carried out as described in paragraph 1. The size and shape of the particles was determined by TEM (Jeol, JEM-1200 EX II, Tokyo, Japan) and the chemical composition was evaluated by a TEM-EDS (CM-200FEG, Philips, Eindhoven, The Netherlands), equipped with energy-dispersive x-ray analysis and with a field-emission gun and a super-twin lens operational up to 200 kV acceleration voltage yielding a point to point resolution of 0.24 nm and a smallest probe size of 1 nm.
In order to determine the particle size distribution of the tested materials, 200 counted particles from TEM images were statistically analyzed for each tested composite .
More specifically, the size of one hundred particles smaller than 100 nm and larger than 100 nm was measured in TEM images at a magnification of 60,000 and 12,000 respectively (with respective scale of 1 and 5.8 nm/pixel). The particle size distribution was based on the minimal Ferret diameter of the particles.
2.4
Extraction procedure
Immediately after collecting the dust, the filters were removed from the cassettes and were placed in glass vials (ref 548-0582, VWR, Haasrode, Belgium) with PFTE crimp cap that were filled with 1 mL extraction solvent. Special care was taken not to contaminate the filters. As extraction solvent, either ethanol (99.99%; CAS: 64-17-5, VWR, Haasrode, Belgium) or sterile water (Water LC/MS-p, Biosolve BV, Valkenswaard, Nederlands) was used, and the vials were rotated for 24 h. Then, the extraction solvent was transferred to LC–MS vials for analysis. Teflon filters without particles were tested as control.
2.5
LC–MS analysis
Liquid chromatography in combination with mass spectroscopy was used to evaluate the release of monomers from composite dust. First, liquid-chromatographic separation was carried out on an Acquity UPLC BEH C18 column (2.1 × 50 mm, 1.7 μm particle size) using an Acquity UPLC system from Waters. Mass analysis (tandem) was carried on Micromass Quattro Premier™ Mass Spectrometer (Waters, Manchester, UK). The compounds were determined using multiple reactions monitoring, with argon as the collision gas.
Good detection and quantification of the monomers was ensured by optimal chromatographic conditions to obtain a chromatogram with optimal resolution, peak shape, and enhanced response. This was done by varying the solvents flow rate (i.e. from 0.30 to 0.50 mL/min) as well as the separation gradient. Formic acid and ammonium hydroxide, known to enhance the MS signal, were used in order to reach low limits of quantification. In brief, the methacrylate monomers were separated in a mobile phase consisting of water (eluent A) and acetonitrile (eluent B) containing 0.1% formic acid at 40 °C using a flow rate of 0.35 mL/min. This is a commonly used solvent with low pH for LC–MS, which ensures good detection and quantification of the tracked compounds. The chromatographic separation program started at 10% eluent B, then increased linearly to 100% in 2 min, this composition was held for 0.5 min before returning to the initial conditions. The analysis was performed using electrospray ionization (ES) in positive mode and the quantification specific MS/MS parameters are shown in Table 2 .
TEGDMA | UDMA | BisGMA | BisEMA | BPA | |
---|---|---|---|---|---|
Retention Time | 1.71 | 1.95 | 2.04 | 2.44 | 2.28 |
MS/MS parameters | |||||
Ionization mode | ES+ | ES+ | ES+ | ES+ | ES- |
Quantifier Transition | 309.6 → 309.6 | 493.7 → 493.7 | 535.3 → 535.3 | 520.5 → 520.5 | 226.9 → 132.8 |
Cone (V) | 35 | 50 | 50 | 50 | 40 |
Collision Energy (V) | 10 | 10 | 15 | 10 | 25 |
Limits of Quantification | |||||
LoQ water (ng/mL) | 78 | 739 | 284 | 212 | 7 |
LoQ ethanol (ng/mL) | 78 | 185 | 284 | 212 | 7 |
Calibration curves in water | |||||
Domain (ng/mL) | 78–3110 | 739–5910 | 284–1137 | 212–1698 | 7–27 |
R 2 value | 0.9843 | 0.9691 | 0.9813 | 0.9834 | 0.9885 |
Calibration curves in ethanol | |||||
Domain (ng/mL) | 78–24880 | 185–11820 | 284–4548 | 212–6792 | 7–107.7 |
R 2 value | 0.9856 | 0.9866 | 0.9827 | 0.9758 | 0.9882 |
The endocrine disruptor, Bisphenol A (BPA) was analyzed independently in order to reach lower limits of quantification (LoQ). BPA was separated at 40 °C using a flow rate of 0.5 mL/min and the mobile phase consisted of water (eluent A’) and methanol (eluent B’) containing 0.5% ammonium hydroxide. The chromatographic separation program started at 5% eluent B’, than increased linearly to 95% in 3 min and this composition was held for 0.7 min before returning to the initial conditions. The analysis was performed using electrospray ionization (ESI) in negative mode and the specific MS/MS parameters are also presented in Table 2 .
The UPLC–MS/MS methods were validated according to the international rules . Calibration curves were generated using standard solutions prepared from commercial solid reference standards in ultrapure water or ethanol for the quantification of samples extracted with water or ethanol, respectively. Stable-isotope-labeled derivatives (D4-diethylphtalate (CAS: 93952-12-6; Cambridge isotope Laboratories Inc., Tewksbury, MA, USA) and D16-bisphenol A (CAS: 96210-87-6, Sigma–Aldrich, St. Louis, MO, USA)) were used to overcome any potential sample manipulation losses. The correlation coefficients, R 2 , of the regression equations exceeded the value 0.9691, demonstrating a good correlation between the measured response (peak area) and the concentration of the target compounds. The limits of quantification were determined based on the lowest calibration levels analyzed in pentaplicates and corresponding to the following performance criteria: accuracy within the interval 80–120% of the target level and repeatability with a relative standard deviation lower than 25% ( Table 2 ).
The eluted amount of monomers and BPA in water and ethanol was statistically compared with two-way ANOVA with each ‘monomer’ and type of ‘solvent’ as factors. To analyze the effect of solvent further, planned comparisons were calculated comparing extraction in ethanol versus water for each monomer. All tests were performed at a significance level of α = 0.05.
2
Materials and methods
2.1
Composites used
Four commercial resin-based composites, including so-called nano(-hybrid) composites and conventional hybrid composites were included in this study. Their composition can be found in Table 1 . For each composite, five sticks with size of 17.4 mm × 5.4 mm × 1.6 mm were prepared in a metal mold following a clinically relevant protocol as per instructions of the manufacturer. The composite was covered with a glass plate, and then light-cured for 20 s with a light curing unit (Bluephase 20i, Ivoclar-Vivadent, Schaan, Liechtenstein) with an output between 1000 and 1100 mW/cm 2 and wavelength 385–515 nm.
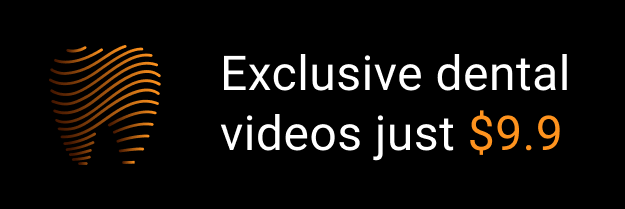