Highlights
- •
Novel bioactive glass (BAG) and fluoride-containing BAG composites were prepared.
- •
We investigated the effects of biofilm on the surface properties of these composites.
- •
Both the biofilm and the media-alone can negatively affect the surface properties.
- •
Biofilm reduced the change of surface roughness and gloss compared to the media-only.
Abstract
Objectives
This study investigated the effects of bacterial biofilm on the surface properties of novel bioactive glass (BAG)-containing composites of different initial surface roughness.
Methods
BAG (65 mol% Si; 4% P; 31% Ca) and BAG-F (61% Si; 31% Ca; 4% P; 3% F; 1% B) were synthesized by the sol–gel method and micronized (size ∼0.1–10 μm). Composites with 72 wt% total filler load were prepared by replacing 15% of the silanized Sr glass with BAG, BAG-F, or silanized silica. Specimens ( n = 10/group) were light-cured and divided into 4 subgroups of different surface roughness by wet polishing with 600 and then up to 1200, 2400, or 4000 grit SiC. Surface roughness (SR), gloss, and Knoop microhardness were measured before and after incubating in media with or without a Streptococcus mutans (UA 159) biofilm for 2 weeks. Results were analyzed with ANOVA/Tukey’s test ( α = 0.05).
Results
The SR of the BAG-containing composites with the smoothest surfaces (2400/4000 grit) increased in media or bacteria; the SR of the roughest composites (600 grit) decreased. The gloss of the smoothest BAG-containing composites decreased in bacteria and media-only, but more in media-alone. The microhardness of all of the composites decreased with exposure to media or bacteria, with BAG-containing composites affected more than the control.
Significance
Exposure to bacterial biofilm and its media produced enhanced roughness and reduced gloss and surface microhardness of highly polished dental composites containing a bioactive glass additive, which could affect further biofilm formation, as well as the esthetics, of restorations made from such a material.
1
Introduction
Bioactive glasses (BAGs) are represented by a range of dense, amorphous calcium, sodium phosphosilicate materials that can develop strong chemical bonds with the collagen in living tissues . This characteristic is known to be related to their ability to form a biologically active hydroxycarbonate apatite layer on their surfaces by releasing ions rapidly when exposed to an aqueous medium, such as body fluids . Thus, BAGs are considered as promising materials for dental use because of their biocompatibility and capability for stimulating the regeneration of hard tissues. Recently, BAG has been considered as a strategic additive for dental restorative materials because it has been shown to possess antimicrobial properties as well as the capability for releasing ions needed for tooth remineralization . While it has been shown that BAG has a high elastic modulus and a low fracture toughness compared to more conventional fillers, such as a strontium glass , a recent study showed that BAG containing composites demonstrate adequate and stable mechanical properties, comparable to three successful commercial composites . Also, when fluoride-containing BAG (BAG-F) was added to a composite formulation, the composite had the potential to release both calcium and fluoride ions in aqueous solution and could also be readily recharged with fluoride .
It is desirable for dental composite materials to have high surface hardness, and to be polishable to a very low surface roughness and to maintain these characteristics during service. Recent studies of resin composite showed that the surface roughness was negatively correlated with the surface microhardness, and that finishing/polishing procedures resulted in harder and smoother surfaces . A study using water and acidic solutions as storage media showed that both the surface roughness and microhardness of resin composites decreased after five weeks . It has also been reported that Streptococcus mutans biofilms grown on the surface of resin composite increased surface roughness without influencing surface microhardness, but that the change in surface integrity could cause a further accumulation of biofilm . These studies suggest that the surface properties of dental composites may be affected by storage media and bacterial exposure, but that more systematic studies are needed. In particular, it is important to systematically study the effect that surface roughness has on biofilm formation on composites, as it has been suggested that biofilm formation is enhanced when average surface roughness (Ra) exceeds 0.2 μm .
In the present study, novel composites containing bioactive glass fillers, with and without fluoride, were synthesized and characterized in terms of surface roughness, composition, and effects of S. mutans colonization. The aim of this study was to systematically evaluate the effect of surface roughness (less than and greater than 0.2 μm) of the BAG and BAG-F composites on biofilm formation and to investigate how biofilm accumulation can affect the surface properties of the composites.
2
Materials and methods
2.1
Preparation of BAG
BAGs used in this study were synthesized in our laboratory by sol–gel methods as previously described . After the solution had gelled and was cooled rapidly, the synthetic glasses were ball milled in ethanol and sieved to a gross particle dimension of less than 38 μm. The particles were then further processed using a Micronizer Jet Mill (Sturtevant Inc., Hanover, MA, USA), produce a fine particle size (0.04–3.0 μm) as determined by laser particle size measurements (Beckman Coulter LS13 320, Brea, CA, USA).
2.2
Formulation of experimental composites
The three experimental composites all contained 57 wt% of silane treated strontium glass filler (1–3 μm average size, Bisco, Inc.), and were further modified as follows: the control group included a silane-treated aerosil-silica (OX-50, Degussa) as a control, while micronized BAG (BAG65) and fluorine-contained micronized BAG (BAG61) replaced the silica in the BAG and BAG-F groups ( Table 1 ). The composites were produced using a centrifugal mixing device (Speed-Mixer DAC 150 FVZ, Hauschild, Germany) for 2 min at 2400 rpm and stored in sealed containers at room temperature before testing.
Group | Composition |
---|---|
Control | SG 57 wt%, OX-50 15 wt% and matrix resin 28 wt% |
BAG | SG 57 wt%, BAG65 15 wt% and matrix resin 28 wt% |
BAG-F | SG 57 wt%, BAG61 15 wt% and matrix resin 28 wt% |
2.3
Specimen preparation
Forty-eight disk-shaped specimens of each group (10 mm diameter by 2 mm thickness) were prepared in rubber molds with both the top and bottom surface covered by microscope slide glasses to extrude excess resin. All materials were light-cured using a curing unit with a 12 mm diameter light guide (Demi™, Kerr, Orange, CA, USA) for 40 s at 520–580 mW/cm 2 on each side. Specimens were separated from the mold, any flash on the lateral border was carefully removed, and the specimens were aged for 24 h in the dark at ambient humidity. To produce a reproducible and consistent surface roughness, a specimen holder was created to hold the specimen firmly while delivering a gentle axial force perpendicular to the surface of the silicon carbide paper rotating on a polishing wheel (Struers inc., Cleveland, OH, USA) under cooling water. Every group was divided into four polishing subgroups of twelve specimens designed to produce four distinct ranges of surface roughness. The roughness targets were established by a pilot test using 44 composite specimens; Subgroup P600 was polished against SiC paper of 600-grit; P1200 against 600- and 1200-grit sequentially; P2400 against 600-, 1200-, and 2400-grit sequentially; and P4000 against 600-, 1200-, 2400-, and 4000-grit sequentially. Every polishing step was performed by one investigator checking repeatedly during the polishing to ensure that the correct range of target average surface roughness (Ra) values were obtained.
2.4
Baseline properties testing
The baseline surface roughness (Ra, μm) of each specimen was measured using a surface roughness tester TR200 (TIME Group, Pittsburgh, PA, USA) with a tracing length of 5 mm and a cutoff value of 0.25 μm to maximize filtration of surface waviness. Gloss was measured using a small-area glossmeter (Novo-Curve, Rhopoint Instrumentation, East Sussex, UK), with a square measurement area of 2 mm × 2 mm and 60° geometry. Gloss measurements were expressed in gloss units (GU). Both were measured at four different positions on the specimen, rotated clockwise, at a right angle. Three Knoop indentations were made in each specimen using a universal hardness tester (Duramin, Struers, Ballerup, Denmark) with a load of 0.98 N applied for 5 s (Knoop hardness number, KHN). One specimen of each subgroup ( n = 12) was randomly assigned to be examined using a scanning electron microscope (SEM). The specimen was sputter coated with gold-palladium in a Denton Vacuum Desk II (Denton Vacuum Inc., Moorestown, NJ, USA) and the surface was observed at 15.0 kV in an SEM under 2000x magnification (Quanta 200, FEI Company, Hillsboro, OR, USA).
2.5
Biofilm procedure
Overnight cultures of S. mutans (strain UA159) grown in brain heart infusion (BHI) at 37 °C in an incubator with 5% CO 2 were measured for optical density at 600 nm (OD 600 ) and then diluted to an OD 600 of 0.4–0.6. A 1:10 dilution of the stock solution in new BHI medium was then incubated for 3 h to obtain OD 600 = 0.3, which represents a bacterial concentration of 9 × 10 7 CFU/mL based on previous calibration studies. Culture media was trypticase soy broth (BBL™ Trypticase™ Soy Broth, BD diagnostics, MD, USA) with 3 wt% sucrose (Fischer Science Education, Hanover Park, IL, USA). Six specimens from each group were chosen for the biofilm group and were inoculated with S. mutans . Five specimens were used for the group aged in media only. All specimens ( n = 132) were sterilized using 70% ethyl alcohol in an ultrasonic bath for 15 min followed by absolute ethyl alcohol for 15 min. The sample disks were placed at the bottom of the wells of six well culture plates. For the S. mutans group, a 1:100 subculture of S. mutans was added to the culture media and 5 mL was placed in each well using a sterile pipette. For the control group, only 5 mL of the culture media free of S. mutans was placed in each well. All specimens were incubated in 5% CO 2 at 37 °C for 14 days, with the culture media being removed every 24 h and replaced with 5 mL of fresh culture media using a sterile pipette. On the 13th day of the experiment, the samples were checked for contamination by culturing in BBL™ Trypticase™ Soy Agar with 5% Sheep Blood (BD diagnostics, Hunt Valley, MD, USA).
One of the samples in each S. mutans group ( n = 12) was chosen for SEM examination of the biofilm by fixing with 4% glutaraldehyde in distilled water at room temperature. The biofilms were removed from the other samples by gently wiping with tissues (Kimwipes™, Kimberly-Clark, Dallas, TX, USA) and sterile water in order to prepare the specimens’ surface for surface roughness, gloss, microhardness, and SEM imaging.
2.6
Post incubation properties
The samples on which biofilm was formed were carefully removed from the fixative solution and were dried at room temperature for 24 h. They were sputter coated with gold-palladium, and their surfaces were examined under SEM in the same way as the pretreatment examination. In all the other samples of which biofilm was removed from the specimen surface, Ra, GU, and KHN measurements were repeated in the same way as the baseline measurements. They were also coated and studied using SEM.
2.7
Statistical analysis
Statistical comparisons between pre- and post-treatment were made among each polishing level of P600, P1200, P2400, and P4000 for all three materials. The normality of the studied parameters was checked using the Kolmogorov–Smirnov test. If all followed normal distribution, the values of the studied parameters in each group were compared by paired t -test. Otherwise, they were analyzed using Wilcoxon Signed Ranks Test. For within-group comparison, if they satisfied the assumptions of normality and homogeneity of variance (Levene’s test), they were compared by analysis of variance (ANOVA). According to the results of Levene statistic, post hoc comparisons were performed using Tukey or Dunnett’s T3. If any of the parameters did not follow a normal distribution, they were analyzed using the Kruskal Wallis test. All statistical analyses were performed with a significance level of p ≤ 0.05 using SPSS 21 (IBM Corp., Somers, NY, USA).
2
Materials and methods
2.1
Preparation of BAG
BAGs used in this study were synthesized in our laboratory by sol–gel methods as previously described . After the solution had gelled and was cooled rapidly, the synthetic glasses were ball milled in ethanol and sieved to a gross particle dimension of less than 38 μm. The particles were then further processed using a Micronizer Jet Mill (Sturtevant Inc., Hanover, MA, USA), produce a fine particle size (0.04–3.0 μm) as determined by laser particle size measurements (Beckman Coulter LS13 320, Brea, CA, USA).
2.2
Formulation of experimental composites
The three experimental composites all contained 57 wt% of silane treated strontium glass filler (1–3 μm average size, Bisco, Inc.), and were further modified as follows: the control group included a silane-treated aerosil-silica (OX-50, Degussa) as a control, while micronized BAG (BAG65) and fluorine-contained micronized BAG (BAG61) replaced the silica in the BAG and BAG-F groups ( Table 1 ). The composites were produced using a centrifugal mixing device (Speed-Mixer DAC 150 FVZ, Hauschild, Germany) for 2 min at 2400 rpm and stored in sealed containers at room temperature before testing.
Group | Composition |
---|---|
Control | SG 57 wt%, OX-50 15 wt% and matrix resin 28 wt% |
BAG | SG 57 wt%, BAG65 15 wt% and matrix resin 28 wt% |
BAG-F | SG 57 wt%, BAG61 15 wt% and matrix resin 28 wt% |
2.3
Specimen preparation
Forty-eight disk-shaped specimens of each group (10 mm diameter by 2 mm thickness) were prepared in rubber molds with both the top and bottom surface covered by microscope slide glasses to extrude excess resin. All materials were light-cured using a curing unit with a 12 mm diameter light guide (Demi™, Kerr, Orange, CA, USA) for 40 s at 520–580 mW/cm 2 on each side. Specimens were separated from the mold, any flash on the lateral border was carefully removed, and the specimens were aged for 24 h in the dark at ambient humidity. To produce a reproducible and consistent surface roughness, a specimen holder was created to hold the specimen firmly while delivering a gentle axial force perpendicular to the surface of the silicon carbide paper rotating on a polishing wheel (Struers inc., Cleveland, OH, USA) under cooling water. Every group was divided into four polishing subgroups of twelve specimens designed to produce four distinct ranges of surface roughness. The roughness targets were established by a pilot test using 44 composite specimens; Subgroup P600 was polished against SiC paper of 600-grit; P1200 against 600- and 1200-grit sequentially; P2400 against 600-, 1200-, and 2400-grit sequentially; and P4000 against 600-, 1200-, 2400-, and 4000-grit sequentially. Every polishing step was performed by one investigator checking repeatedly during the polishing to ensure that the correct range of target average surface roughness (Ra) values were obtained.
2.4
Baseline properties testing
The baseline surface roughness (Ra, μm) of each specimen was measured using a surface roughness tester TR200 (TIME Group, Pittsburgh, PA, USA) with a tracing length of 5 mm and a cutoff value of 0.25 μm to maximize filtration of surface waviness. Gloss was measured using a small-area glossmeter (Novo-Curve, Rhopoint Instrumentation, East Sussex, UK), with a square measurement area of 2 mm × 2 mm and 60° geometry. Gloss measurements were expressed in gloss units (GU). Both were measured at four different positions on the specimen, rotated clockwise, at a right angle. Three Knoop indentations were made in each specimen using a universal hardness tester (Duramin, Struers, Ballerup, Denmark) with a load of 0.98 N applied for 5 s (Knoop hardness number, KHN). One specimen of each subgroup ( n = 12) was randomly assigned to be examined using a scanning electron microscope (SEM). The specimen was sputter coated with gold-palladium in a Denton Vacuum Desk II (Denton Vacuum Inc., Moorestown, NJ, USA) and the surface was observed at 15.0 kV in an SEM under 2000x magnification (Quanta 200, FEI Company, Hillsboro, OR, USA).
2.5
Biofilm procedure
Overnight cultures of S. mutans (strain UA159) grown in brain heart infusion (BHI) at 37 °C in an incubator with 5% CO 2 were measured for optical density at 600 nm (OD 600 ) and then diluted to an OD 600 of 0.4–0.6. A 1:10 dilution of the stock solution in new BHI medium was then incubated for 3 h to obtain OD 600 = 0.3, which represents a bacterial concentration of 9 × 10 7 CFU/mL based on previous calibration studies. Culture media was trypticase soy broth (BBL™ Trypticase™ Soy Broth, BD diagnostics, MD, USA) with 3 wt% sucrose (Fischer Science Education, Hanover Park, IL, USA). Six specimens from each group were chosen for the biofilm group and were inoculated with S. mutans . Five specimens were used for the group aged in media only. All specimens ( n = 132) were sterilized using 70% ethyl alcohol in an ultrasonic bath for 15 min followed by absolute ethyl alcohol for 15 min. The sample disks were placed at the bottom of the wells of six well culture plates. For the S. mutans group, a 1:100 subculture of S. mutans was added to the culture media and 5 mL was placed in each well using a sterile pipette. For the control group, only 5 mL of the culture media free of S. mutans was placed in each well. All specimens were incubated in 5% CO 2 at 37 °C for 14 days, with the culture media being removed every 24 h and replaced with 5 mL of fresh culture media using a sterile pipette. On the 13th day of the experiment, the samples were checked for contamination by culturing in BBL™ Trypticase™ Soy Agar with 5% Sheep Blood (BD diagnostics, Hunt Valley, MD, USA).
One of the samples in each S. mutans group ( n = 12) was chosen for SEM examination of the biofilm by fixing with 4% glutaraldehyde in distilled water at room temperature. The biofilms were removed from the other samples by gently wiping with tissues (Kimwipes™, Kimberly-Clark, Dallas, TX, USA) and sterile water in order to prepare the specimens’ surface for surface roughness, gloss, microhardness, and SEM imaging.
2.6
Post incubation properties
The samples on which biofilm was formed were carefully removed from the fixative solution and were dried at room temperature for 24 h. They were sputter coated with gold-palladium, and their surfaces were examined under SEM in the same way as the pretreatment examination. In all the other samples of which biofilm was removed from the specimen surface, Ra, GU, and KHN measurements were repeated in the same way as the baseline measurements. They were also coated and studied using SEM.
2.7
Statistical analysis
Statistical comparisons between pre- and post-treatment were made among each polishing level of P600, P1200, P2400, and P4000 for all three materials. The normality of the studied parameters was checked using the Kolmogorov–Smirnov test. If all followed normal distribution, the values of the studied parameters in each group were compared by paired t -test. Otherwise, they were analyzed using Wilcoxon Signed Ranks Test. For within-group comparison, if they satisfied the assumptions of normality and homogeneity of variance (Levene’s test), they were compared by analysis of variance (ANOVA). According to the results of Levene statistic, post hoc comparisons were performed using Tukey or Dunnett’s T3. If any of the parameters did not follow a normal distribution, they were analyzed using the Kruskal Wallis test. All statistical analyses were performed with a significance level of p ≤ 0.05 using SPSS 21 (IBM Corp., Somers, NY, USA).
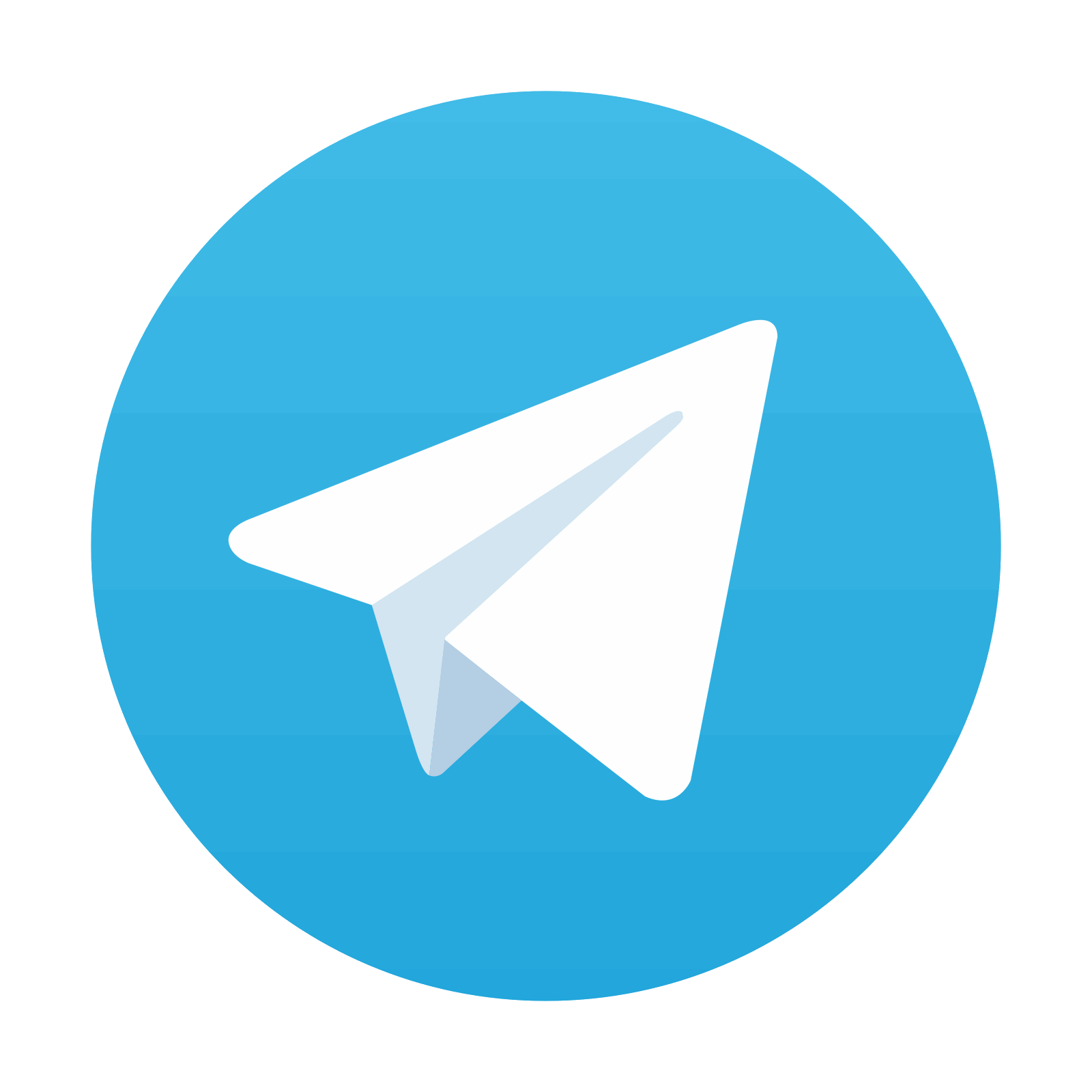
Stay updated, free dental videos. Join our Telegram channel

VIDEdental - Online dental courses
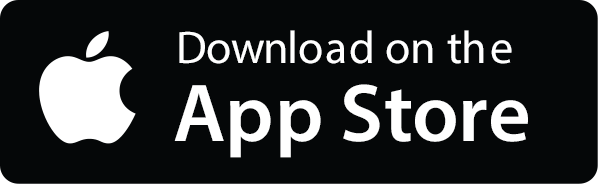
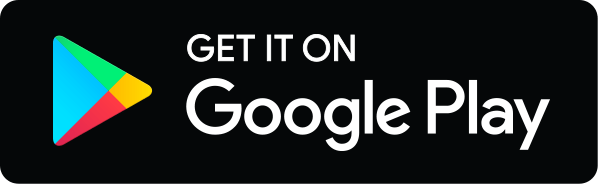