Highlights
- •
Different kinds of filler particles were compared in a composite resins.
- •
Low particle-matrix coupling leads to low mechanical strength.
- •
However, strength of composites with tetrapodal zinc oxide fillers is increased.
- •
Functionalization with lauric acid decreases strength of composite resins.
Abstract
Objective
The aim of this study was to evaluate whether the mechanical properties of the modern dental composites can be improved by using tetrapodal ZnO particles as fillers in a Bis-GMA/TEGDMA matrix. Another aim was to test whether the mechanical properties of the composite are influenced by functionalization of the ZnO particles with lauric acid to achieve antibacterial activity.
Methods
Different filler materials and particle shapes (spherical zirconia, spherical zinc oxide, tetrapodal zinc oxide) were used to produce Bis-GMA/TEGDMA based composites with a filler content of 40 wt.-% and 60 wt.-%, respectively. In addition, functionalization with lauric acid was investigated. For the biaxial flexural strength testing 104 test disks ( N = 8) with a diameter of 15 mm and a thickness of 1.5 mm were produced.
Results
Functionalization with lauric acid resulted in a decrease in biaxial flexural strength for all filler materials. The biaxial flexural strength decreased when using a higher filler content with spherical particles but increased when using tetrapodal zinc oxide particles.
Significance
A higher durability of the composites using tetrapodal zinc oxide particles. An antibacterial functionalization with lauric acid cannot be recommended as the mechanical stability of the composite will be reduced.
1
Introduction
Nowadays composite resin materials are mainly used for dental fillings, because amalgam, the material preferred in earlier times, has many disadvantages as the possibility of toxic reactions , lack of adhesion to the tooth, limited mechanical strength and lack of esthetics . Composite resins should provide similar structural, physical and mechanical properties as the natural tooth structures . Therefore composite resin materials are mainly made of inorganic components that are embedded in an organic matrix. This is imitating the natural tooth, as the dentin and the enamel also have a large content of inorganic material . However, a clinical study has shown that the survival rate of the composite fillings after seven years was lower (85.5%) than the survival rate of the amalgam (94.4%) .
Often zirconia fillers are used to improve the mechanical properties of composite resins materials . However not only filler materials properties but also the shape of the filler particles have a considerable influence on the mechanical properties of the final composite resin. It has been shown that the mechanical properties of the composite can be improved by changing the particle shape from spherical to tetrapodal . While it is quite simple and cost effective to produce tetrapodal shaped particles, if the chosen material is zinc oxide , it is quite difficult to produce zirconia filler particles of tetrapodal shape.
The main reason for failure of the dental fillings is secondary caries caused by bacteria . It has been shown that the colonization of caries-producing bacteria is enhanced by the higher surface energy and surface roughness of the composite fillings . Several approaches to prevent the bacterial growth have been made. Antibacterial agents can be added to the filling materials, but the supply will be exhausted after a short time . Additives may also change the mechanical properties of the composites . However, it has been shown that lauric acid is an antibacterial agent against bacteria in various regions of the human body . In another study it has been shown that lauric acid inside the feeding stuff of rats reduces their amount of caries .
Therefore, it was the purpose of this study to evaluate whether the biaxial flexural strength of composite resins can be enhanced by using tetrapodal zinc oxide filler particles instead of spherical filler particles of zirconia or zinc oxide. Another purpose of this study was to evaluate if the functionalization of the particles with lauric acid will affect the biaxial flexural strength of the composite. The first hypothesis of this study is that the functionalization with lauric acid will have no influence on the biaxial flexural strength. The second hypothesis of the study is that tetrapodal zinc oxide filler particles will lead to a higher biaxial flexural strength of the BisGMA/TEGDMA based composite resins than spherical zinc oxide and zirconia fillers.
2
Materials and methods
2.1
Sample preparation
In this study a monomer mixture of BisGMA (Sigma–Aldrich) and TEGDMA (Sigma–Aldrich) with a weight ratio of 60:40 has been used. For the polymerization 0.5 wt-% camphorquinone (97%, Sigma–Aldrich) as photo initiator and 0.5 wt-% ethyl 4-(dimethylamino) benzoate (99%, Sigma–Aldrich) as accelerator were added. After a complete mixture of these components the filling particles were mixed mechanically into the matrix material. Besides the groups of composites with functionalized and not functionalized spherical zirconia and zinc oxide and tetrapodal zinc oxide particles with a filler content of 40 wt-% and 60 wt-% another group of the pure polymer was investigated to serve as a control group. The investigated test groups with their corresponding group codes are listed in Table 1 .
Group code | Shape | Material | Size | Filler content | Functionalization |
---|---|---|---|---|---|
Matrix | – | None | – | 0 wt-% | – |
sZr40-N | Spherical | ZrO 2 | <400 nm | 40 wt-% | None |
sZr40-L | Spherical | ZrO 2 | <400 nm | 40 wt-% | Lauric acid |
sZr60-N | Spherical | ZrO 2 | <400 nm | 60 wt-% | None |
sZr60-L | Spherical | ZrO 2 | <400 nm | 60 wt-% | Lauric acid |
sZn40-N | Spherical | ZnO | <1 μm | 40 wt-% | None |
sZn40-L | Spherical | ZnO | <1 μm | 40 wt-% | Lauric acid |
sZn60-N | Spherical | ZnO | <1 μm | 60 wt-% | None |
sZn60-L | Spherical | ZnO | <1 μm | 60 wt-% | Lauric acid |
tZn40-N | Tetrapodal | ZnO | 5–40 μm | 40 wt-% | None |
tZn40-L | Tetrapodal | ZnO | 5–40 μm | 40 wt-% | Lauric acid |
tZn60-N | Tetrapodal | ZnO | 5–40 μm | 60 wt-% | None |
tZn60-L | Tetrapodal | ZnO | 5–40 μm | 60 wt-% | Lauric acid |
The spherical zirconia (TZ-10YS, TOSOH) and zinc oxide particles (Sigma–Aldrich) have been purchased, while the tetrapodal particles have been synthesized in our laboratory. A scanning electron microscopic image of the tetrapodal zinc oxide particles that were produced as described by Mishra et al. is shown in Fig. 1 . In order to achieve a functionalization with lauric acid, the particles were mixed into a solution of lauric acid (≥98%, Sigma–Aldrich) and ethanol. The ratio of the amount of lauric acid to particles to ethanol was 1–20 to 600. After 2 h at 60 °C with continuous stirring the particles were filtered out of the solution and were dried for 24 h at 80 °C. The hydrophobicity was evaluated by adding a water drop to the particles and assessment of the resulting reaction. If the particles had been immersed into the drop, it indicated hydrophilicity of the particles, while the observation that the particles were pushed away by the water drop was used to confirm that the functionalization was successful. In order to achieve a homogeneous mixture, agglomerates were destroyed by an ultrasonic bath. Using a Teflon mold, 8 disks per group with a diameter of 15 mm and a thickness of 1.5 mm were produced by layering technique in order to provide a complete hardening of the specimens. Light curing was performed for 60 s with a LED array with a power of 1.35 W providing a wave length of 466 nm. After light curing of the composite, the surfaces were ground with 600 grit size SiC abrasive paper.
2.2
Biaxial flexural strength
The biaxial flexural strength test was performed by using the piston-on-three ball technique in a universal testing machine (Z010, Zwick, Ulm, Germany). Three 3.2 mm diameter stainless steel balls that were equidistant from each other were placed on a circle with a diameter of 10 mm. The disks were placed centrally on the steel balls and load was applied by a piston of 1.2 mm diameter with crosshead speed of 0.5 mm/min. The fracture load for each specimen was recorded and the biaxial flexural strength was calculated using the following equation:
S = − 0.2387 P ( X − Y ) d 2
where S : biaxial flexural strength (MPa); P : fracture load (N); d : specimen disk thickness at fracture origin (mm). X and Y were determined as follows:
X : ( 1 + u ) ln r 2 r 3 2 + 1 − u 2 r 2 r 3 2
Y : ( 1 + u ) [ 1 + ln r 1 r 3 2 ] + ( 1 − u ) r 1 r 3 2
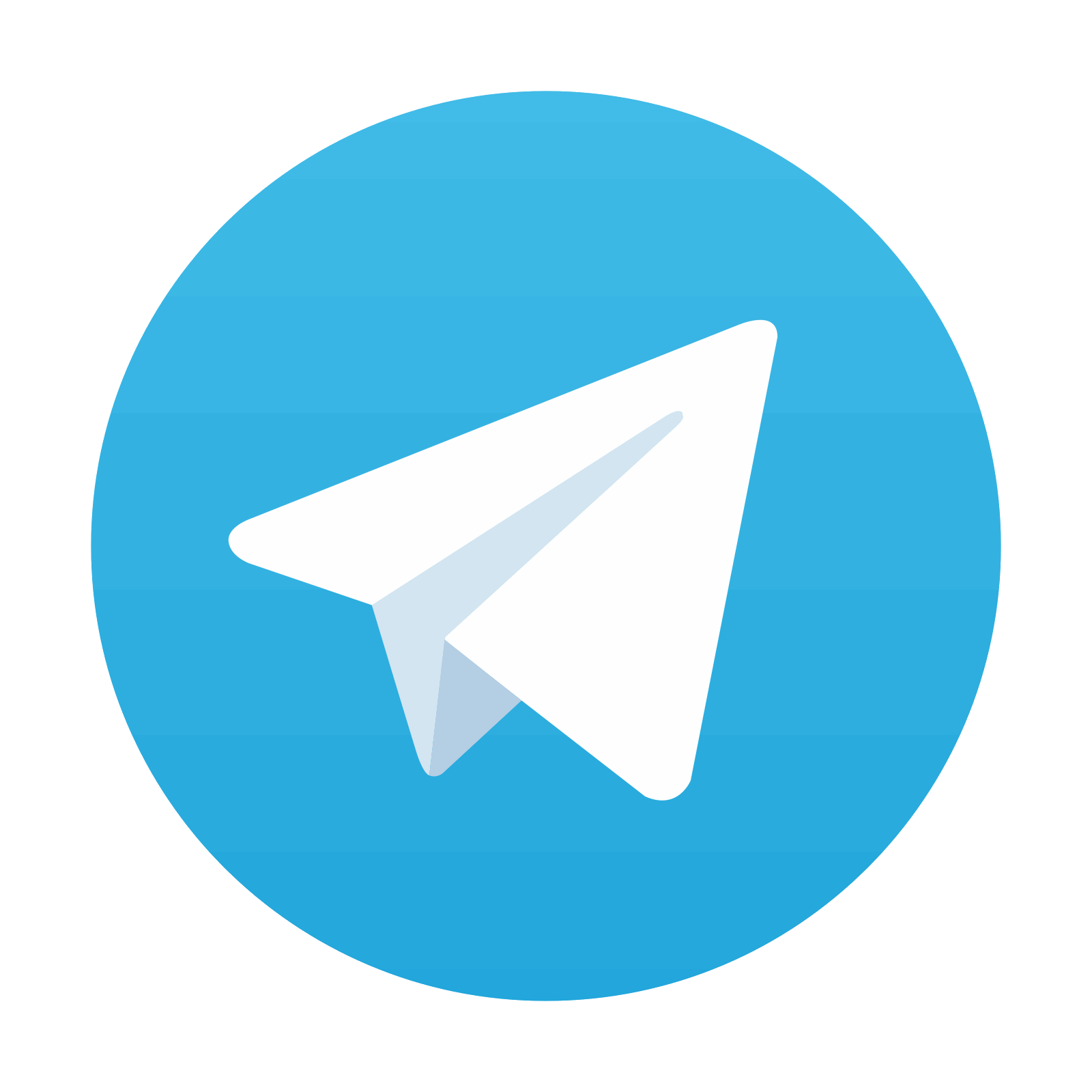
Stay updated, free dental videos. Join our Telegram channel

VIDEdental - Online dental courses
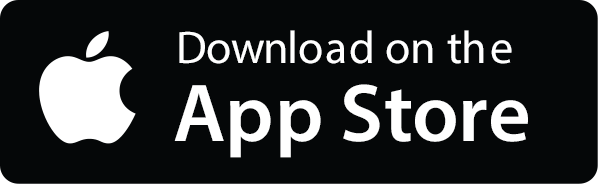
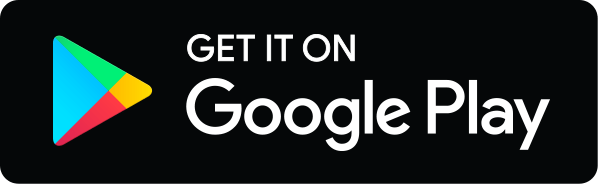