Sleep can be defined as a complex reversible state characterized by behavioral quiescence, diminished responsiveness to external stimuli, and a stereotypical species-specific posture. Both components of sleep, non–rapid eye movement and rapid eye movement, are generated and maintained by central nervous system networks that use specific neurotransmitters located in specific areas of the brain. Widespread changes in physiologic processes occur during sleep, and these changes may influence the presentation and severity of specific medical disorders.
Sleep can be defined as a complex reversible state characterized by behavioral quiescence, diminished responsiveness to external stimuli, and a stereotypical species-specific posture. Unlike other states of reduced awareness and receptivity to environmental influences, such as coma, delirium, or encephalopathy, both components of sleep, non–rapid eye movement (NREM) and rapid eye movement (REM), are generated and maintained by central nervous system (CNS) networks that use specific neurotransmitters located in specific areas of the brain.
Control of sleep and waking
Three physiologic processes control sleep latency (ie, time taken to fall asleep), duration and quality, and alertness, namely, (1) sleep homeostasis, (2) circadian rhythm, and (3) sleep inertia. In addition, the timing of sleep and waking is also determined by behavioral influences, such as social activities, meals, and work responsibilities.
Sleep homeostasis is the increase in sleep pressure related to the duration of immediate prior wakefulness. This sleep pressure declines with sustained sleep. Slow wave (delta) activity (SWA) is often used as a marker of homeostatic sleep pressure among adults. Like sleep homeostasis, SWA increases during sustained wakefulness and diminishes after sustained NREM sleep. Thus, it is more difficult to arouse a person when SWA is high. The neurotransmitter adenosine is believed to regulate this homeostatic sleep drive.
The circadian rhythm, on the other hand, is independent of the sleep-wake cycle. Its main function is promoting wakefulness. Most individuals have 2 circadian rhythm–related peaks in alertness, during the late morning and early evening, and 2 periods of circadian troughs in alertness, in the early morning and early midafternoon. Increase in circadian alertness resists the increase in homeostatic sleep pressure during the waking period, and a decreasing circadian alertness opposes the decreasing homeostatic sleep throughout the sleep period, thereby maintaining constant alertness during the biological day and consolidated sleep during the biological night.
Sleep inertia, or a period of relative confusion and disorientation during the transition between sleep and waking, is responsible for the sensation of sleepiness at the start of the biological day when sleep homeostasis is at its lowest level.
Neuroanatomy of sleep and waking
The states of wake, NREM sleep, and REM sleep are each generated by discrete but interconnected neural networks that use specific neurotransmitters. There are several key sleep-wake neurotransmitters, including glutamate (main CNS excitatory neurotransmitter), γ-aminobutyric acid (GABA, main NREM neurotransmitter), acetylcholine (main REM sleep neurotransmitter), and glycine (responsible for inhibition of spinal motor neurons that cause muscle atonia during REM sleep).
Main neurotransmitters (and their neurons) involved in generating waking include acetylcholine (basal forebrain and pedunculopontine tegmentum/laterodorsal tegmentum in the brainstem), dopamine (substantia nigra), glutamate (reticular formation, thalamus, and hypothalamus), histamine (tuberomammillary nucleus), hypocretin (lateral hypothalamic perifornical region), norepinephrine (locus coeruleus), and serotonin (dorsal raphe). Main neurotransmitters (and their neurons) involved in the generation of sleep include acetylcholine (for REM sleep), adenosine (basal forebrain), GABA (ventrolateral preoptic region), and melatonin (pineal gland).
Acetylcholine is both a wake and a REM sleep neurotransmitter and is responsible for cortical electroencephalographic (EEG) desynchronization seen during these 2 stages. Cholinergic agonists (eg, physostigmine) increase REM sleep, whereas cholinergic antagonists (eg, tricyclic antidepressants) decrease REM sleep.
Adenosine, a sleep neurotransmitter, is, as stated earlier, responsible for the homeostatically driven sleep pressure. A byproduct of the breakdown of adenosine triphosphate (ATP) released by glutamate-stimulated astrocytes, levels of adenosine progressively increase during prolonged wakefulness and decrease during sleep. Adenosine acts by inhibiting wake-promoting regions of the brain (via A 1 receptors) and activating ventrolateral preoptic nucleus (VLPO) neurons (via A 2A receptors). Adenosine receptor blockers (eg, caffeine) increase wakefulness and decrease EEG SWA during sleep.
Dopamine is a wake neurotransmitter; D 1 receptor agonists (eg, amphetamines) that enhance dopamine release increase wakefulness, and dopamine antagonists (haloperidol and chlorpromazine) promote sleep. Glutamate is another wake and REM neurotransmitter, levels of which increase during waking and REM sleep and decrease during NREM sleep. Glutamate stimulates the release of ATP by astrocytes. Histamine, a wake neurotransmitter, is blocked by first-generation histamine-1 receptor blockers (eg, diphenhydramine) and by low-dose doxepin, leading to sedation. GABA agonists (eg, barbiturates, benzodiazepines receptor agonists, and sodium oxybate) cause sedation and sleepiness.
Hypocretin (orexin) promotes wakefulness and suppresses REM sleep, and dysfunction of this neurotransmitter is associated with narcolepsy-cataplexy. Agonists of norepinephrine (eg, isoproterenol), a wake neurotransmitter, increase arousal and wakefulness. Precursors and reuptake inhibitors of serotonin, a wake neurotransmitter, promote wakefulness.
Through their effects on one or more of these neurotransmitters, drugs and substances can be sedating, alerting, or both, because of direct action on the neuronal receptors, adverse reaction, or withdrawal effect. For example, stimulants can prolong sleep onset latency and decrease total sleep time by increasing levels of dopamine and norepinephrine (seen with amphetamine, cocaine, and methylphenidate), by augmenting the actions of hypocretin and dopamine (believed to account for the effects of modafinil and armodafinil), by decreasing adenosine levels (caffeine), or by enhancing acetylcholine release (nicotine). Stimulants, as a class, decrease sleepiness and fatigue, increase alertness, and enhance daytime performance and memory. On the other hand, sedating medications (eg, benzodiazepine receptor agonists expressing affinity for GABA A receptors and ramelteon, a melatonin receptor agonist) generally shorten sleep onset latency; long acting formulations of sedating agents may also increase both total sleep time and sleep efficiency (ratio of total sleep time to total time in bed).
Aminergic neurons, activity of which decreases during NREM and REM sleep relative to waking, and cholinergic neurons, which are active during wake and REM sleep, interact to switch between wakefulness and sleep (NREM and REM).
Neuroanatomy of sleep and waking
The states of wake, NREM sleep, and REM sleep are each generated by discrete but interconnected neural networks that use specific neurotransmitters. There are several key sleep-wake neurotransmitters, including glutamate (main CNS excitatory neurotransmitter), γ-aminobutyric acid (GABA, main NREM neurotransmitter), acetylcholine (main REM sleep neurotransmitter), and glycine (responsible for inhibition of spinal motor neurons that cause muscle atonia during REM sleep).
Main neurotransmitters (and their neurons) involved in generating waking include acetylcholine (basal forebrain and pedunculopontine tegmentum/laterodorsal tegmentum in the brainstem), dopamine (substantia nigra), glutamate (reticular formation, thalamus, and hypothalamus), histamine (tuberomammillary nucleus), hypocretin (lateral hypothalamic perifornical region), norepinephrine (locus coeruleus), and serotonin (dorsal raphe). Main neurotransmitters (and their neurons) involved in the generation of sleep include acetylcholine (for REM sleep), adenosine (basal forebrain), GABA (ventrolateral preoptic region), and melatonin (pineal gland).
Acetylcholine is both a wake and a REM sleep neurotransmitter and is responsible for cortical electroencephalographic (EEG) desynchronization seen during these 2 stages. Cholinergic agonists (eg, physostigmine) increase REM sleep, whereas cholinergic antagonists (eg, tricyclic antidepressants) decrease REM sleep.
Adenosine, a sleep neurotransmitter, is, as stated earlier, responsible for the homeostatically driven sleep pressure. A byproduct of the breakdown of adenosine triphosphate (ATP) released by glutamate-stimulated astrocytes, levels of adenosine progressively increase during prolonged wakefulness and decrease during sleep. Adenosine acts by inhibiting wake-promoting regions of the brain (via A 1 receptors) and activating ventrolateral preoptic nucleus (VLPO) neurons (via A 2A receptors). Adenosine receptor blockers (eg, caffeine) increase wakefulness and decrease EEG SWA during sleep.
Dopamine is a wake neurotransmitter; D 1 receptor agonists (eg, amphetamines) that enhance dopamine release increase wakefulness, and dopamine antagonists (haloperidol and chlorpromazine) promote sleep. Glutamate is another wake and REM neurotransmitter, levels of which increase during waking and REM sleep and decrease during NREM sleep. Glutamate stimulates the release of ATP by astrocytes. Histamine, a wake neurotransmitter, is blocked by first-generation histamine-1 receptor blockers (eg, diphenhydramine) and by low-dose doxepin, leading to sedation. GABA agonists (eg, barbiturates, benzodiazepines receptor agonists, and sodium oxybate) cause sedation and sleepiness.
Hypocretin (orexin) promotes wakefulness and suppresses REM sleep, and dysfunction of this neurotransmitter is associated with narcolepsy-cataplexy. Agonists of norepinephrine (eg, isoproterenol), a wake neurotransmitter, increase arousal and wakefulness. Precursors and reuptake inhibitors of serotonin, a wake neurotransmitter, promote wakefulness.
Through their effects on one or more of these neurotransmitters, drugs and substances can be sedating, alerting, or both, because of direct action on the neuronal receptors, adverse reaction, or withdrawal effect. For example, stimulants can prolong sleep onset latency and decrease total sleep time by increasing levels of dopamine and norepinephrine (seen with amphetamine, cocaine, and methylphenidate), by augmenting the actions of hypocretin and dopamine (believed to account for the effects of modafinil and armodafinil), by decreasing adenosine levels (caffeine), or by enhancing acetylcholine release (nicotine). Stimulants, as a class, decrease sleepiness and fatigue, increase alertness, and enhance daytime performance and memory. On the other hand, sedating medications (eg, benzodiazepine receptor agonists expressing affinity for GABA A receptors and ramelteon, a melatonin receptor agonist) generally shorten sleep onset latency; long acting formulations of sedating agents may also increase both total sleep time and sleep efficiency (ratio of total sleep time to total time in bed).
Aminergic neurons, activity of which decreases during NREM and REM sleep relative to waking, and cholinergic neurons, which are active during wake and REM sleep, interact to switch between wakefulness and sleep (NREM and REM).
Circadian rhythms
Biological rhythms are ubiquitous, being present in prokaryotic and eukaryotic microbes, plants, insects, and animals, including humans. These rhythms are characterized by specific amplitude (maximal excursion from peak to nadir), peak, nadir, phase, and frequency (number of oscillations per unit time). The term circadian refers to 1 oscillation approximately every 24 hours. Phase is the temporal position of the endogenous rhythm in relation to an external reference, such as the 24-hour light-dark cycle. A phase is considered advanced if it is shifted to an earlier time in the 24-hour cycle or delayed if it occurs later during the cycle. Most intrinsic human circadian rhythms are not exactly 24 hours (commonly about 24.2 hours) and tend to free run at this longer frequency in the absence of environmental time cues. The process by which external cues adjust the phase (forward or backward) of the intrinsic circadian rhythms is called entrainment, and the environmental cues that are capable of entraining intrinsic circadian rhythms are referred to as zeitgebers. Light is the dominant zeitgeber, but other factors such as exogenous melatonin and timing of activities are capable of phase shifting intrinsic circadian rhythms. Light provided at night before minimum core body temperature (CTmin) can cause phase delay of the sleep-wake rhythm, and morning light provided after CTmin can cause a phase advance.
The suprachiasmatic nucleus (SCN) is the master circadian rhythm generator in mammals, promoting wakefulness during the day and consolidating sleep during the night. The main afferent pathway involves photic stimuli reaching the retina and from the retina to the SCN via the retinohypothalamic tract. Retinal ganglion cells contain melanopsin and are most sensitive to shorter wavelength light (blue to blue-green). Other photic (GABAergic, histaminergic, and cholinergic) and nonphotic (serotonergic) pathways also exist. The SCN has efferent projections to several CNS areas, including the hypothalamus and pineal gland, where melatonin is synthesized and released. Like light exposure, melatonin can influence the sleep-wake rhythm, phase delaying and advancing the rhythm when given in the morning and evening, respectively.
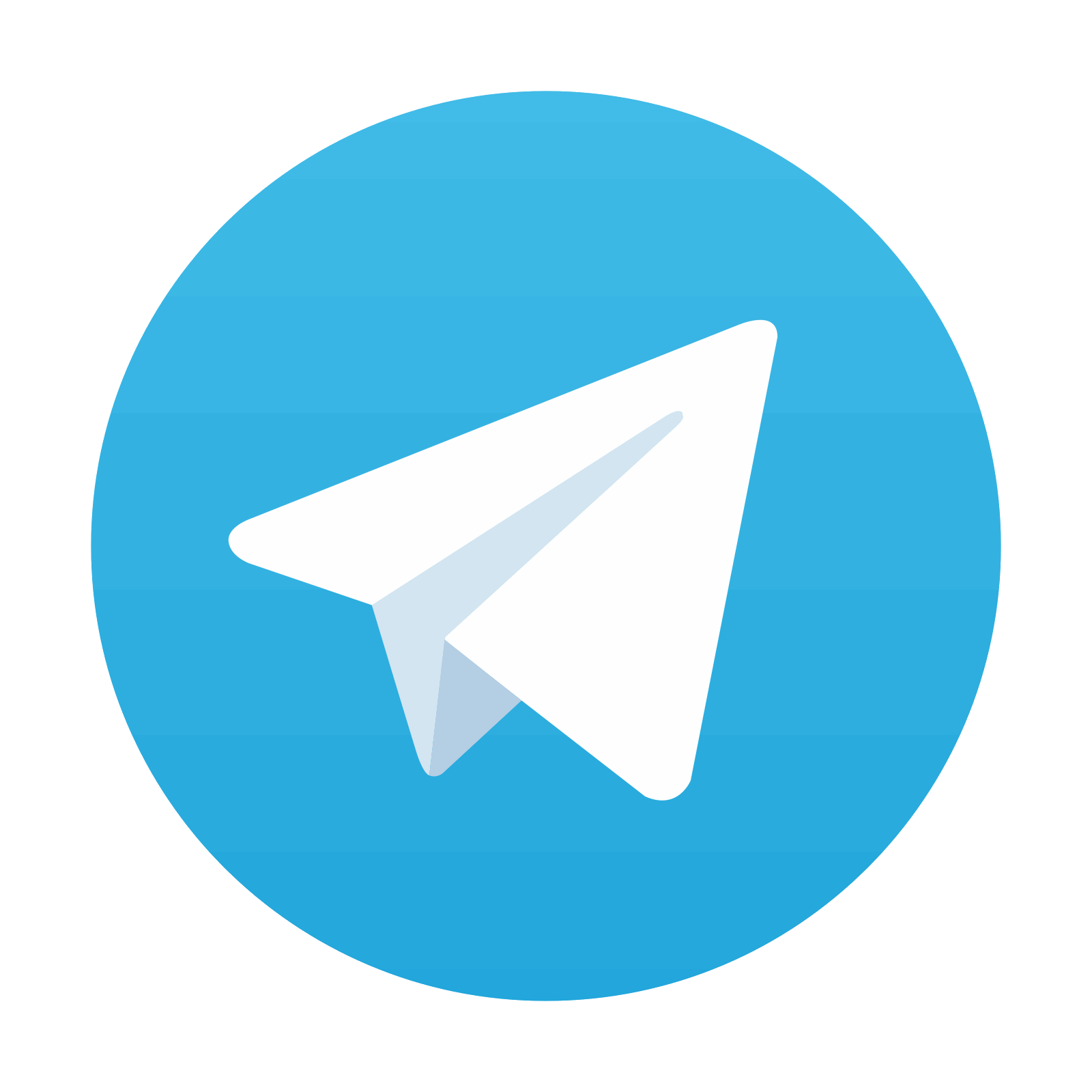
Stay updated, free dental videos. Join our Telegram channel

VIDEdental - Online dental courses
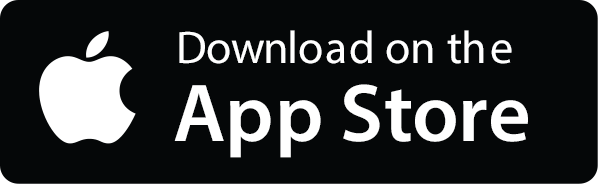
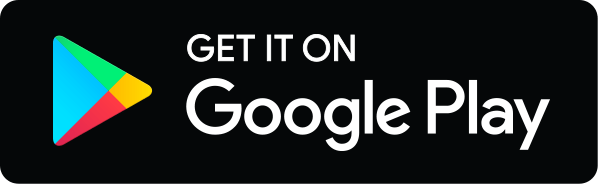