Key Terms
Impression materials
Base paste (base putty) The component that forms the main three-dimensional structure of a final impression.
Catalyst paste (catalyst putty) A component of a polymerization reaction that decreases the energy required for the reaction and usually does not become part of the final product; however, the term catalyst has been used for the structural component of dental materials that initiates the polymerization reaction.
Colloid A solid, liquid, or gaseous substance made up of large molecules or masses of smaller molecules that remain in suspension in a surrounding continuous medium of different matter.
Elastomer Any of various polymers having the elastic properties of natural rubber.
Gel A network of fibrils forming a weak, slightly elastic brush-heap structure of hydrocolloid; also, the solid network structure of a crosslinked polymer.
Gelation The process of transforming a hydrocolloid from a sol to a gel.
Imbibition The displacement of one fluid by another immiscible fluid in a hydrocolloid. In the context of impression materials, imbibition is the uptake by agar or alginate when immersed in water.
Inelastic Incapable of sustaining significant elastic deformation without fracturing under stress.
Model A positive full-scale replica of teeth, soft tissues, and restored structures used as a diagnostic aid for the construction of orthodontic and prosthetic appliances; a facsimile used for display purposes.
Pseudoplastic A non-Newtonian behavior of fluids whose viscosity decreases under shear strain until it reaches a nearly constant value. Thus, the more rapidly pseudoplastic fluids are stirred or forced through a syringe, the less viscous (thinner) and more easily they flow.
Shear thinning The tendency for viscosity to decrease as the shearing rate increases (see pseudoplastic and thixotropy ).
Static mixing A technique of transforming two paste-like materials (or fluids) into a homogeneous mixture without mechanical stirring; two streams of material are forced into a mixer cylinder, and the stationary elements in the mixer divide and recombine the feed materials and keep the material streams spiraling through the cylinder simultaneously.
Syneresis The expression of fluid onto the surface of gel structures.
Thixotropy The property of certain gels or fluids to become less viscous when sufficient energy in the form of impact force or vibration is applied; at rest, they require a specific duration to return to the previous viscous state. Both pseudoplasticity and thixotropy are shear-thinning processes; the difference is that changes in pseudoplastic viscosity do not exhibit the time dependency characteristic of thixotropy.
Viscoelastic/viscoelasticity The ability of a material to strain instantaneously like an elastic solid during rapid stretching or to resist shear flow and to strain linearly over time (like honey) when a stress is applied continuously.
Gypsum Products
Dental plaster (plaster of Paris) The beta form of calcium sulfate hemihydrate (CaSO 4 • ½H 2 O).
Dental stone The alpha form of calcium sulfate hemihydrate (CaSO 4 • ½H 2 O).
Hygroscopic setting expansion The expansion that occurs when gypsum or a gypsum-bonded investment sets while immersed in water (usually heated to approximately 38 °C [100 °F]).
Normal setting expansion The expansion that occurs when gypsum or a gypsum-bonded investment sets in ambient air.
Waxes
Baseplate wax Dental wax provided in sheet form to establish the initial arch form in the construction of complete dentures.
Bite wax A wax form used to record the occlusal surfaces of teeth as an aid in establishing maxillo-mandibular relationships.
Boxing wax A wax sheet form used as a border at the perimeter of an impression to provide an enclosed boundary for the base of the cast to be made from a poured material such as gypsum or resin.
Corrective wax (dental impression wax) A thermoplastic wax that is used to make a type of dental impression.
Dental wax (1) A low-molecular-weight ester of fatty acids derived from natural or synthetic components, such as petroleum derivatives, that soften to a plastic state at a relatively low temperature. (2) A mixture of two or more waxes and additives used as an aid for the production of gypsum casts, the production of nonmetallic denture bases, the registering of jaw relations, and laboratory work.
Flow Relative ability of wax to plastically deform when heated slightly above body temperature.
Inlay wax A specialized dental wax that can be applied to dies to form direct or indirect patterns for the lost wax technique, which is used for the casting of metals or hot pressing of ceramics.
Refractory Capable of sustaining exposure to a high temperature without significant degradation.
Sprue The mold channel through which molten metal or ceramic flows into a mold cavity.
Sticky wax A type of dental wax that exhibits high adhesion to dry, clean surfaces when heated to a plastic condition.
Prosthetic devices and restorations are made to adapt to the anatomy of the oral tissue receiving the device. If the device is to be fabricated by the lost wax technique, a pattern with the exact dimensions of the device must be made with a material that is stable at room temperature but that can be removed by melting or burning out. The pattern usually is made of wax. To ensure close adaptation or fit for cementation of the fabricated device, the pattern must be fabricated on a model or die. A model (cast) or die can be made from gypsum products using an impression mold (or negative likeness) of a dental structure ( Figure 13-1 ). Gypsum is a powder that, when mixed with water, becomes flowable and capable of being poured into an impression mold and capturing and reproducing the fine detail of the impression. Gypsum models are dimensionally stable during the entire process of pattern making and are resistant to scratching during pattern fabrication. To duplicate the anatomical form of the oral tissue, an impression material that is initially moldable is forced against the tissue and then sets. After removal, the impression should remain stable while models or dies are being made. These materials are required for making prostheses but do not become part of a final prosthesis. They are thus known as auxiliary materials . Impression materials, gypsum products, and waxes have multiple applications in addition to making prostheses. This chapter focuses on the use and relevant properties for general applications.

How are dental impression materials classified?
Classification of Impression Materials
To produce accurate replicas of intra- and extraoral tissues, the impression materials should be (1) sufficiently fluid to adapt to the oral tissues, (2) viscous enough to be contained in a tray, (3) able to transform (set) into a rubbery or rigid solid in the mouth in a reasonable time (<7 minutes), (4) resistant to distortion or tearing when removed from the mouth, (5) dimensionally stable long enough to allow one or more casts to be poured, (6) biocompatible, and (7) cost-effective in terms of the required processing time and the expense of the materials and associated processing equipment.
By their composition, the impression materials are divided into three major groups: nonaqueous elastomers, hydrocolloids, and inelastic materials. Elastomers include polysulfide, condensation and addition silicone, and polyether. Hydrocolloids are alginate and agar. Inelastic materials comprise impression compound, plaster of Paris, and zinc oxide–eugenol (ZOE).
They are also classified by mechanism of setting, mechanical properties, and clinical applications. The setting mechanisms of impression materials can be reversible and irreversible. Reversible materials, such as agar and impression compound, soften upon heating and retain the shape when cooled in the oral cavity, with no chemical changes taking place. Irreversible materials imply that chemical reactions have occurred during impression making. The set impression materials can be inelastic (rigid) or elastic. Set rigid materials are highly resistant to flexure but fracture when deformed. They include ZOE impression paste, impression plaster, and impression compound. The term elastic in impression materials means that the material can be flexed easily without fracture and returns to its original form when unstressed. Hydrocolloids and elastomers fall into this category. The ability of elastic impression materials to rebound after removal from the mouth makes them suitable for reproducing both the hard and soft structures of the mouth, including the undercuts and interproximal spaces. Inelastic impression materials, such as ZOE and plaster, are ideal for making impressions of edentulous jaw structures or soft tissue because they do not compress the tissue during seating of the impression tray. Impression compound is often used to make trays for the construction of full dentures. Table 13-1 shows the classification based on the setting mechanism and mechanical characteristics.
Setting Mechanism | MECHANICAL CHARACTERISTICS | |
---|---|---|
Inelastic | Elastic | |
Chemical reaction (irreversible) | Plaster of Paris Zinc oxide–eugenol | AlginatePolysulfidePolyetherCondensation siliconeAddition silicone |
Thermally induced physical reaction (reversible) | Impression compound | Agar |
Environmental conditions and the type of tissue dictate the choice of materials, the quality of the impression, and the quality of the cast. The next three sections discuss the unique properties of three categories of impression materials by composition and describe how they affect the quality of the impressions and the casts.
Elastomeric Impression Materials
Elastomeric impression materials are crosslinked polymers when set that can be stretched and yet rapidly recover to their original dimensions when stress is released. Chemically, there are three elastomers based on polysulfide, silicone (polysiloxane), and polyether. A background covering the underlying fundamentals of polymeric materials is presented in Chapter 2, Polymers .
Chemistry of Elastomeric Impression Materials
Elastomeric impression materials are supplied in two components, a base and a catalyst (or accelerator), that are mixed before making impressions. The term catalyst or accelerator used here may be a misnomer with certain material systems. Reactor is a more appropriate term for the polysulfide and condensation silicone because they participate in the reaction. In the case of polyether, the catalyst contains initiators. The materials are often formulated in several consistencies, including extra low, low, medium, heavy, and putty, in increasing order of filler content. Extra-low and putty forms are available only for condensation and addition silicones. Polysulfide is provided only in light-body and heavy-body consistencies. Pigments are added to give each material a distinct color. As a group of impression materials, elastomers are classified by consistency measured immediately after complete mixing as type 0: putty, type 1: heavy-bodied, type 2: medium-bodied, and type 3: light-bodied (International Organization of Standard ISO 4823:2015).
Polysulfide
The base paste contains a liquid prepolymer with multifunctional mercaptan (–SH) groups, a suitable filler to provide the consistency for mixing and the required strength when set, a plasticizer to confer the appropriate viscosity for workability of the paste, and a small quantity of sulfur (~0.5%) to accelerate the reaction. The catalyst paste contains lead dioxide, filler, and plasticizer, as in the base paste, and oleic or stearic acid as a retarder to control the rate of the setting reaction. Each paste is supplied in a dispensing tube with appropriately sized bore diameters at the tip so that equal lengths of each paste are extruded from each tube to provide the correct ratio of base to crosslinking agent.
The reaction starts as soon as mixing begins, and a resilient network has started to form ( Figure 13-2 ). During the final set, a material of adequate elasticity and strength is formed so that the impression can be removed past undercuts quite readily. Elevated temperature and humid conditions will accelerate the setting of polysulfide impression material. The reaction yields water as a by-product. Loss of water from the set material has a significant effect on the dimensional stability of the impression.

Condensation Silicone
The materials are supplied as a base paste and a liquid catalyst, a two-paste system, or a two-putty system. The putty can be used as the tray material in conjunction with a low-viscosity silicone, which is referred to as the putty-wash technique.
The base paste consists of α-ω-hydroxyl-terminated polydimethyl siloxane ( Figure 13-3 ). The curing of this material involves a reaction of tri- and tetra-functional alkyl silicates in the presence of stannous octoate as a catalyst. The material sets by condensation reaction between terminal groups of the silicone polymers and the alkyl silicate to form a three-dimensional network ( Figure 13-3 ). Ethyl alcohol is a by-product of the condensation reaction that evaporates, and the evaporation accounts for much of the contraction that occurs during setting.

Addition Silicone
This material is often called a polyvinyl siloxane (PVS) or vinyl polysiloxane (VPS) impression material. The base paste contains polymethylhydrosiloxane and other siloxane prepolymers. The catalyst paste contains divinylpolysiloxane and a platinum salt. Both pastes contain fillers. The addition silicone is based on addition polymerization between divinylpolysiloxane and polymethylhydrosiloxane with a platinum salt as the catalyst ( Figure 13-4 ). No reaction by-products are formed if the correct proportions of base and catalyst pastes are used and there are no impurities. However, the residual polymethylhydrosiloxane in the material can lead to a secondary reaction with each other or with moisture, producing hydrogen gas. Technically, hydrogen gas is a reaction by-product that does not affect the dimensional stability of the impression. Nonetheless, the hydrogen gas evolved can result in pinpoint voids in the gypsum casts poured soon after the removal of the impression from the mouth. A noble metal, such as palladium, can be added as a scavenger for the released hydrogen gas. The impression should be left overnight if epoxy is used for pouring models.

Sulfur contamination from natural latex gloves inhibits the setting of addition silicone. Some vinyl gloves may have the same effect because of the sulfur-containing stabilizer used in the manufacturing process. The contamination is so pervasive that touching the tooth with latex gloves before seating the impression can inhibit the setting of the critical surface next to the tooth.
Medium-body addition silicone has also been formulated for making impressions for diagnostic purposes, as a substitute for alginate impression material, an irreversible hydrocolloid to be discussed later. The advantage of these so-called alginate substitutes is the ability to make multiple, accurate diagnostic casts from one impression. Laboratory studies have reported that they exhibited better detail reproduction and less variability in linear dimensional change than alginates.
Polyether
There are two types of polyether impression materials. The first is based on the ring-opening polymerization of aziridine rings, which are at the end of branched polyether molecules ( Figure 13-5 ). The main chain is probably a copolymer of ethylene oxide and tetrahydrofuran. Crosslinking and setting are promoted by an initiator and an aromatic sulfonate ester, where R is an alkyl group. This produces crosslinking by cationic polymerization via the imine end groups. The base paste contains the polyether prepolymer, colloidal silica as filler, and a plasticizer such as glycol ether or phthalate. The accelerator paste contains an alkyl-aromatic sulfonate in addition to the filler and plasticizer. The ether-dominated polymer backbones make this group of materials the most hydrophilic of all elastomeric impression materials.

What are the steps needed to produce casts and dies for prosthetic procedures?
The second type is based on an acid-catalyzed condensation polymerization ( Figure 13-3 ) of polyether prepolymer with alkoxysilane terminal groups. The mechanism is like that observed in condensation silicones having low-molecular-weight alcohols as by-products. This material is often called a hybrid. Because the ether linkages constitute the main component of the polymer chains, these materials behave very much like the first type of polyether impression material.
Making Impressions With Elastomeric Materials
The use of elastomeric impression material to fabricate gypsum models, casts, and dies involves six major steps: (1) preparing a tray, (2) managing tissue, (3) preparing the material, (4) making an impression, (5) removing the impression, and (6) preparing for stone casts and dies.
Impression Trays
The use of a custom tray ( Figure 13-6 , left ) requires less impression material than a stock tray to make impressions, thus minimizing dimensional changes attributable to the materials. A custom tray allows a uniform distribution of impression material between the tray and the tissue, which also improves accuracy. This is especially true for polysulfide impression material. The use of custom trays for polyether and addition silicone impressions is not critical because these materials are stiffer and have less polymerization shrinkage than polysulfide. In addition, disposable stock trays ( Figure 13-6 , right ) work satisfactorily. Note that the use of less material in a custom tray reduces the compressibility of the impression, which can make removal of the impression tray more difficult. When severe undercuts are present, the use of a custom tray should be avoided. The stock tray should be rigid, thereby minimizing flexure of the tray during impression removal. Prior to making an impression, a uniform thickness of tray adhesive is applied on the tray surface, extending over its edge, and allowed to dry (through evaporation of solvent). Tray adhesives are not interchangeable among different types of materials.

Tissue Management
The margins of tooth preparations for fixed prostheses often extend to or below the free margin of the gingiva. To ensure access for the tooth preparation and for making the impression, displacing the gingival tissues is necessary to control gingival hemorrhage and sulcular fluids from distorting the margins of the crown preparation. Among the most popular methods of gingival displacement is the use of gingival retraction cord. An electrosurgical unit or a soft tissue laser can also be used.
Placing a retraction cord displaces the gingival tissue laterally away from the margin of the preparation. One or two gingival retraction cords are placed under the margin around the tooth for at least 5 minutes before making impressions. The double-cord technique is used when the margin is very close to the gingival attachment. A fine cord is placed at the base of the crevice to facilitate moisture control, with a larger cord placed on top of the first and near the coronal extent to displace the gingiva laterally. The outermost cord is removed, leaving the fine cord within the crevice while the impression is made. A single cord is sufficient to deflect the soft tissue around the margin when the preparation margin is at or slightly above the gingival crest.
Retraction cords may be impregnated with a hemostatic agent by dipping the cord in a hemostatic solution prior to placement. These agents can have unintended side effects and should be used with caution. For example, epinephrine, which is used widely, is of concern in patients with cardiovascular disease. In addition, agents with a low pH can remove the smear layer and superficial dentin apical to the margins of the preparation, possibly leading to postoperative sensitivity of some teeth.
How does static mixing achieve a uniform mixture? What is the main difference between static mixing and dynamic mixing?
Manipulation of Impression Materials
Currently, elastomeric impression materials are offered for three modes of mixing: hand mixing, static mixing, and dynamic mechanical mixing ( Figure 13-7 ).

Hand Mixing
The user dispenses the same lengths of materials onto a mixing pad ( Figure 13-7, A ). The catalyst paste is first collected on a stainless-steel spatula and then spread over the base paste. The mixture is spread over the mixing pad. The mass is then scraped up with the spatula blade and spread uniformly back and forth on the mixing pad. This process continues until the mixed paste is uniform in color, with no streaks of the base or catalyst appearing in the mixture. If one of the components is in liquid form, such as the catalyst for condensation silicones, a length of the base is dispensed from the tube onto a graduated mixing pad, and drops of the liquid catalyst corresponding to the length of the base are added.
The two-putty systems available for condensation and addition silicone are dispensed by volume using an equal number of scoops of each material. The best mixing technique is to knead the material with one’s gloved fingers until a uniform color is obtained.
Static Mixing
This technique transforms two flowable (or paste-like) materials into a homogeneous mixture without mechanical mixing. The device comprises a gun for pushing materials in a two-cylinder cartridge (base and catalyst), along with a mixing tip ( Figure 13-7, B ). The mixing tip is made of helical mixer elements in a cylindrical housing ( Figure 13-8 ). The mixer elements are a series of alternating right- and left-turn 180° helixes positioned so that the leading edge of one element is perpendicular to the trailing edge of the other one ( Figure 13-8, B ).

The base and catalyst are pressed from the cartridge into the mixing tip as one stream of a two-layer material. The leading edge of the first element splits the material entering the mixer into two streams. The streams that flow on either side of the helix will make a 180° turn ( Figure 13-8, A ) when they reach the second element. Both streams are split by the leading edge of the second element and become two streams of four-layer material (two layers from each original stream) entering the third element. This process is known as flow division. Even if there is no intermixing between layers as they merge to a new stream, there will be 2 n layers in either stream as they come out of the n th element. For a mixing tip with 11 elements, the stream that exits the mixing tip will have a 2048-striation structure, which is practically a uniformly mixed stream of material. In addition, as the materials make turns along the helix, the rotational circulation causes a radial mixing of the materials. Thus mixing between layers occurs before the subsequent flow division that further increases the uniformity of the mixture. Because there is no mechanical mixing, porosity caused by mixing with air is avoided.
The mixed impression material is injected directly into the adhesive-coated tray or, if the “syringe tip” is in place, onto the prepared teeth. One can also use an intraoral syringe ( Figure 13-7, C ) to apply light-body material onto prepared teeth. One precaution that should be taken is to make sure that the openings of the cartridges that dispense the pastes remain unclogged. Problems can be avoided if one expresses a small amount of material from the cartridge before attaching the mixing tip and discards the first centimeter of mixture that emerges from the mixing tip. This process is called bleeding . This type of device has also been adapted to mix and dispense acrylic-based materials for fabricating temporary crown and bridge, and luting cements that are used for luting prostheses ( Chapter 7, Clinical Manipulation and Evaluation ).
Dynamic Mechanical Mixing
The device shown in Figure 13-7, D uses a motor to drive parallel plungers, forcing the materials into a mixing tip and out into an impression tray or syringe; meanwhile, the motor-driven impeller, which is inside the mixing tip, mixes the materials as they are extruded through the tip. The function of the impeller is only to mix the materials as they are passing through; it does not propel the material. The materials are supplied in collapsible plastic bags housed in a cartridge. The amount of material retained in the mixing tip is slightly greater than that used in static mixing. In using this device, thorough mixing of higher-viscosity materials can be achieved with little effort. Both polyether and addition silicone impression materials of various viscosities are available with this dispensing system.
How does each impression-making technique take advantage of the unique properties of the impression materials?
Techniques of Making Impressions
Elastomeric impression materials are typically supplied in several viscosities to accommodate the different techniques for impression making. Three techniques for making impressions are discussed in this section.
Multiple-Mix Technique
A syringe material (light body) and a tray material (heavy body) are used in this technique. When they are hand mixed, they are mixed simultaneously, each by a different person because one must load the syringe with the light-body material. With mechanical devices, the materials can be mixed as needed by one individual. The lighter material is dispensed from the filled syringe or directly from a static mixing gun within and around the tooth preparation. The filled tray is then inserted in the mouth and seated over the syringe material. The tray material will force the syringe material to adapt to the prepared tissues. The two materials should bond together upon setting. If either material has progressed past the working time when brought together, the bond between them will be compromised. If a partially set material is seated, the material will be compressed elastically. Once removed from the mouth, the impression will “spring back” or relax, and the dies made from this impression will be too narrow and too short, as illustrated in Figure 13-9 .

In rare cases a clinician may attempt to repair an impression that has small defects or that lacks sufficient detail. This is usually performed by cutting away the interproximal and gingival areas of the impression. Reseating the tray precisely will be difficult even with proper relief of the initial impression. Entrapment of a minute fragment of impression material or debris will eliminate any chance of a successful repair. The impression material’s surface must be roughened to ensure that the new material bonds to the set impression. The safest method is to make a new impression when bubbles or similar defects are detected in critical areas.
Monophase Technique
Medium-body polyether and addition silicone are often used for the monophase or single-viscosity technique. The procedure is like that of the multiple-mix technique except that only one mixture is made, and part of the material is placed in the tray, and another portion is placed in the syringe for injection in the cavity preparation, prepared teeth, or soft tissue. The success of this technique depends on the pseudoplastic (shear-thinning) properties of the materials. When a medium-viscosity material is forced through the syringe tip, the viscosity is reduced, facilitating material adaptation to the preparation. Meanwhile, the material in the tray retains a medium viscosity, and when seated, the tray material can force the syringe material to flow past critical areas of the tooth preparation. Table 13-2 shows the effect of shear rate and elapsed time on some monophase addition silicones.
VISCOSITY AT 1 min | VISCOSITY AT 1.5 min | |||
---|---|---|---|---|
Material | 0.5 rpm | 2.5 rpm | 0.5 rpm | 2.5 rpm |
Baysilex (Miles) | 122.1 (2.8) * | 68.9 (2.5) | 211.2 (14.7) | 148.8 (1.2) |
Green-Mouse (Parkell) | 133.7 (8.9) | 56.7 (2.9) | 247.9 (14.9) | 78.0 (2.8) |
Hydrosil (Caulk) | 194.2 (8.5) | 129.4 (4.1) | 398.1 (7.8) | 153.5 † |
Imprint (3M) | 106.5 (12.2) | 79.7 (2.2) | 245.1 (8.9) | 146.2 (5.9) |
Omnisil (Coe) | 156.8 (11.5) | 102.5 (1.9) | 347.1 (5.2) | 153.5 ‡ |
* Numbers within parentheses represent the standard deviation of the mean.
† Value at 75 seconds after mixing.
‡ Value at 77 seconds after mixing. From Kim K-N, Craig RG, Koran A: Viscosity of monophase addition silicones as a function of shear rate, J Prosthet Dent 67:794, 1992.
What are the consequences of placing an impression material in the mouth after the working time has expired?
Putty-Wash Technique
The thick putty material is placed in a stock tray, and a preliminary impression is made. This procedure results in what is essentially an intraoral custom-made tray formed by the putty. Space for the light-body “wash” material is provided either by cutting away some of the “tray” putty or by using a thin polyethylene sheet as a spacer between the putty and the prepared teeth during preliminary impression making. A mixture of the thin-consistency wash material is placed into the putty impression and on the preparation; then the tray is reseated in the mouth to make the final impression.
Removal of the Impression
Under no circumstances should the impression be removed until the curing has progressed sufficiently to provide adequate elasticity. One method for determining the time of removal is to inject some of the syringe material onto a space that is not in the field of operation before inserting the impression tray. This material can be probed with a blunt instrument from time to time; when the material is firm and returns completely to the original contour, the impression is sufficiently elastic to be removed. Typically, the impression should be ready for removal within at least 10 minutes from the time of mixing, allowing 6 to 8 minutes for the impression to remain in the mouth. Manufacturers usually provide instructions as to the optimal time for removal after mixing.
The mechanics of removing the impression involve separation at the impression–tissue interface and stretching of the impression. The first step is to break the physical adhesion between the tissue and the impression; therefore an impression material, such as polyether, that wets the tissue well will require extra effort to break the adhesion (seal) for the removal. The second step is stretching the impression enough to pass under the height of contour of the hard tissue to remove the impression; therefore using a material of higher rigidity will require a greater force to stretch the impression to facilitate removal. Polysulfide has the lowest viscosity and ranks as the least stiff of the elastomeric impression materials of a similar consistency. This flexibility allows the set material to be easily removed from undercut areas and from the mouth with a minimum of stress.
In addition, all elastomeric impression materials are viscoelastic, and it is necessary to use a quick snap to minimize plastic deformation of the impression during the final step of the removal process. The phenomenon of viscoelastic behavior is discussed in subsequent sections.
Preparation of Stone Casts and Dies
The hydrophobic characteristics of silicone impression make pouring with gypsum products challenging because it increases the potential of forming voids in gypsum dies and casts. One can spray a thin layer of surfactant, also known as debubblizer, on the impression to improve the surface wettability for the stone mixture. A dilute solution of soap is also an effective surfactant. One can also select a hydrophilized addition silicone (discussed later) to make impressions. Pouring of a stone cast in a polyether or polysulfide impression does not require the aid of a surfactant.
The excellent dimensional stability of addition silicone and polyether impression materials makes the construction of two or three casts or dies from one impression possible. It is also possible to construct successive stone dies or casts from polysulfide impressions when duplicate stone dies are needed. It should be noted that each successive die will be less accurate than the first die constructed from the impression. One should wait before the next pour after the removal of the cast but not greater than 30 minutes. To minimize tearing and gross distortion after the first pour, the clinician should remove the excess gypsum-forming mass from undercut areas along the periphery of the tray. Be aware that the stiffness of the impression material makes it difficult to remove the stone cast from the impression. A weak stone cast may fracture during removal.
Properties of Elastomeric Impression Materials
The ability to make a cast or die that reproduces the surface details and precise shape of the original tissue depends on various properties of the impression material. In this section, the properties relevant to impression making with elastomers are described. The comparative properties of elastomeric impression materials are listed in Table 13-3 .
Property | Polysulfide | Condensation Silicone | Addition Silicone | Polyether | ||||
---|---|---|---|---|---|---|---|---|
Working time (min) | 4–7 | 2.5–4 | 2–4 | 3 | ||||
Setting time (min) | 7–10 | 6–8 | 4–6.5 | 6 | ||||
Tear strength (N/m) | 2500–7000 | 2300–2600 | 1500–4300 | 1800–4800 | ||||
Percent contraction (at 24 h) | 0.40–0.45 | 0.38–0.60 | 0.14–0.17 | 0.19–0.24 | ||||
Contact angle between set material and water (°) | 82 | 98 | 98/53 * | 49 | ||||
Hydrogen gas evolution (Y/N) | N | N | Y † | N | ||||
Automatic mixing (Y/N) | N | N | Y | Y | ||||
Custom tray (Y/N) | Y | N | N | N | ||||
Unpleasant odor (Y/N) | Y | N | N | N | ||||
Multiple casts (Y/N) | N | N | Y | Y | ||||
Stiffness (value of 1 indicates greatest stiffness) ‡ | 3 | 2 (1) | 2 (1) | 1 (2) | ||||
Distortion on removal (value of 1 indicates the greatest and 4 the least potential distortion) | 1 | 2 | 4 | 3 |
* The lower contact angle resulted from testing of a hydrophilized polyvinyl siloxane (PVS).
† A hydrogen absorber is often included to eliminate hydrogen gas evolution.
‡ The numbers in the parentheses reflect the ranking when a soft formulation of polyether impression material is considered.
Why is it not advisable to alter the base/catalyst (accelerator) ratio as a means of controlling working or setting time?
Working and Setting Times
The working time begins at the start of mixing and ends just before the elastic properties develop. The setting time is the time elapsed from the beginning of mixing until the material has enough strength to be removed from the mouth without distortion. Remember, however, that polymerization may continue for a considerable time after. An increase in temperature accelerates the rate of polymerization of all elastomeric impression materials; therefore the effect of temperature on working and setting time should be taken into consideration.
Altering the base/catalyst ratio will change the curing rate of these materials. Normally, having more base materials in the mixture tends to increase the working and setting times. Keep in mind that altering the base/catalyst ratio may not produce a predictable change in the polymerization rate.
Why is rapid seating of an impression tray not advisable for a pseudoplastic impression material?
Rheological Properties
Ideally, the impression material should flow freely and wet the tissue as it is being injected to achieve adaptation, then become immobilized immediately, which keeps the material from flowing away from the intended surface areas. The same property will facilitate spreading of heavy-body material on the impression tray and retain it in the tray. This phenomenon is called shear thinning, which was discussed in Chapter 3, Rheology . All elastomeric impression materials exhibit shear-thinning characteristics before setting.
There are two categories of shear thinning: pseudoplasticity and thixotropy. A pseudoplastic material displays decreasing viscosity with increasing shear stress and recovers its viscosity immediately upon termination of shear stress. A thixotropic material does not exhibit a reduction of viscosity until sufficient agitation (shear stress) is applied to overcome the yield stress of the material. After the termination of shear stress, it takes a specific time to regain its previous viscous state. The latter is important because it takes time for the impression material to adapt to the surface. Manufacturers often emphasize their materials as being thixotropic and also note that the materials exhibit no slump when injected on a vertical surface. They usually do not mention the duration of the time delay.
The benefit of thixotropy in impression making has been disputed because the time duration needed for the impression material to recover the necessary viscosity may be unacceptable. However, reports suggest that a thixotropic material should facilitate handling and make better impressions. One should be aware that these studies are often conducted with unmixed base and catalyst pastes. From the point of impression making, the viscosity of a uniformly mixed impression also increases with polymerization, regardless of the effect of thixotropy. Therefore the impact of thixotropy during impression making may not be critical. In addition, confirming the thixotropic nature of a material without reporting the time lag required before regaining the intrinsic viscosity is not meaningful.
How does the viscoelastic property of impression materials dictate the way an impression must be removed from a patient’s mouth?
Elasticity and Viscoelasticity
An impression material sustains some deformation as it is removed from the mouth, but it must rebound to its preremoval dimensions to preserve the dimensions of the preparation. All elastomeric impression materials are viscoelastic and exhibit both viscous and elastic characteristics. Recall that viscous materials, such as honey, resist shear flow but continue to flow as long as stress is applied. Elastic materials strain (deform) when a stress is applied, but the strain does not increase with time if no additional stress is applied, and they immediately return to their original state once the stress is removed. Essentially, viscoelastic materials exhibit time-dependent strain. Thus, under a constant stress (load), the straining process takes time to complete, and after the load is lifted, the recovery process also takes time and allows a certain degree of plastic (nonrecoverable) deformation to take place. In addition, the amount of deformation is related to the duration of loading. Therefore an impression may sustain deformation after being subjected to various forces during removal and then, in time, recovers most of the deformation after it is allowed to rest. This explains why removal of the impression should be done quickly (in a “snap”) if possible, and prolonged teasing or rocking should be avoided.
Polyvinyl siloxane impression materials exhibit the most elastic recovery of the currently available materials. Distortion on removal from undercuts is virtually nonexistent. However, the excellent elastic properties present a problem in that the heavy-body putty material begins to acquire elastic properties while it is still in the working-time stage. If the material is at an advanced stage of elasticity and is compressed excessively during the seating of the impression, distortion can occur when the material elastically rebounds ( Figure 13-9 ).
The difficulty of removing earlier generations of polyether impressions from undercut areas is attributed to their high modulus of elasticity. The new generations of polyether impression materials, with a stiffness lower than that of hydrophilic PVS, were formulated specifically to address stiffness concerns. However, the inherent hydrophilicity of these polyethers has resulted in greater adhesion between the tissue and the material, which still makes impression removal difficult. It is important to note that increased precision also implies greater adaptation and resultant adhesion between the impression and the tissue, especially the teeth, and increased difficulty of removing the impression. Because of the reduced rigidity, these new generations of polyether impression material may not be the material of choice for the “triple-tray” technique, where the impression material’s stiffness during removal compensates for the flexibility of the tray. Although these new polyether impression materials are flexible during removal, they have been shown to increase their elastic moduli for some time after removal. As the formulation of impression materials continues to evolve to accommodate specific needs, grouping the stiffness of impression materials based on the backbone of polymer chains is becoming less meaningful.
What is the difference between tear strength and tear energy? Why is tear energy more meaningful for impression making?
Tear Strength
The subgingival regions of the impression are often very thin and can tear during impression removal, leaving a portion embedded within the gingival sulcus ( Figure 13-10 ). The tear strength is influenced by consistency and the manner of removing the materials. A heavier consistency usually increases the tear strength of the material. The addition of a thinning agent to the mixture reduces the tear strength slightly but increases the flexibility substantially. A quick snap in removing the impression usually increases the tear strength because the action increases the resistance of the material to deformation (strain).

Tear strength measures the resistance of an elastomeric material to fracture when subjected to a tensile force acting perpendicular to a surface flaw. Figure 13-11 shows two specimen designs that have been used by the dental community for testing impression materials. The amount of force needed to tear a specified test specimen, divided by the thickness of the specimen, is called the tear strength . Note that the calculation of tear strength disregards the dimensional change for the test specimen. For the same tooth preparation, a material that can be stretched a great deal elastically before fracture will likely remain intact, whereas a rigid material can fracture at a much lower tensile strain at a higher level of force. The latter will appear to have higher tear strength but is more likely to fracture than the former. Thus the use of tear strength to distinguish the resistance of an impression material to tearing is not very meaningful. The property that can delineate the combined behavior of stress and strain is energy ( Chapter 4, Resilience and Toughness ), which includes the dimensional change of the material in the calculation. The tear energy is determined by the force (F) needed to keep the crack propagating, the thickness of the specimen (t) and the extension ratio (λ). The extension ratio is the extended portion of the length (Δl) at the end of the test divided by the original length of the specimen (l) ( Figure 13-11 ). The calculated value also has the units of N/m, which can be converted to N⋅m/m 2 and then J/m 2 , an expression of energy per unit area.

Polysulfide materials have the highest resistance to tearing. Therefore thin sections of polysulfide impression material are less likely to tear than polyether or silicone impression materials of a similar thickness. However, because of their susceptibility to permanent distortion, polysulfide impressions may distort rather than tear. This presents a problem because tearing can be seen immediately by carefully checking the impression, whereas distortion is difficult to detect by visual inspection. Because the strain rate influences tear resistance and permanent deformation, the impression should be strained rapidly for as short a time as possible to minimize adverse effects.
What are the main causes of impression inaccuracy?
Dimensional Stability
Dimensional stability refers to the lack of dimensional changes of the impression over time. There are six major sources of dimensional change: (1) polymerization shrinkage, (2) loss of a condensation reaction by-products (water or alcohol), (3) thermal contraction from oral temperature to room temperature, (4) absorption of water or disinfectant over a period of time, (5) incomplete recovery of deformation because of viscoelastic behavior, and (6) incomplete recovery because of plastic deformation. Figure 13-12 shows the mean linear contraction for four elastomers using the method described in American National Standards Institute American Dental Association (ANSI/ADA) Specification No. 19 and ISO 4823.

Figure 13-12 shows that if maximal accuracy is to be maintained, the slurry mix for a stone die or cast should be poured within the first 30 minutes after the polysulfide and condensation silicone impression are removed from the mouth. The stability of the addition silicone and polyether materials suggests that these impressions do not have to be poured with a gypsum product immediately. Research has shown that pouring between 24 hours and 1 week produced casts as accurate as those made in the first hour. Multiple pouring of the impressions and removal of the casts will not alter the dimensional stability of the impression, even though a substantial force is needed each time a cast is removed from the impression.
One variable that can have a negative effect on the polyether impression is the absorption of water or fluids and the simultaneous leaching of the water-soluble plasticizer during disinfectant immersion longer than 10 minutes. However, recent research has shown that 30-minute immersion does not have a negative effect on the dimensions. Nonetheless, the polyether impression should be stored in a dry (relative humidity below 50%), cool environment to maintain its accuracy and should never be left for protracted periods in disinfecting solutions.
Why are hydrophilic materials potentially more susceptible to distortion during disinfection prior to being poured with a gypsum-forming product?
Disinfection
All elastomeric impression materials can be disinfected with all disinfectants registered with the U.S. Environmental Protection Agency (EPA) without the loss of surface quality or accuracy if the disinfection time is short. The impressions should be immersed for the time specified for each disinfectant, as discussed in the last section. After disinfection, the impression should be removed, rinsed, and poured with the gypsum product as soon as possible.
Table 13-4 shows a guide for selecting appropriate disinfection methods for all types of impressions that are subsequently transferred to a dental laboratory.
Material | Method | Recommended Disinfectant | Comments |
---|---|---|---|
Alginate | Immersion with caution Use only disinfectant for a short-term exposure time (<10 min for alginate) | Chlorine compounds or iodophors | Short-term glutaraldehyde has been shown to be acceptable, but time is inadequate for disinfection. |
Agar | Do not immerse in alkaline glutaraldehyde! | ||
Polysulfide and silicone | Immersion | Glutaraldehydes, chlorine compounds, iodophors, phenolics | Disinfectants requiring more than 30-min exposure times are not recommended. |
Polyether | Immerse with cautionUse disinfectant only for a short exposure time (<10 min) | Chlorine compounds or iodophors | ADA recommends any of the disinfectant classes; however, short-term exposures are essential to avoid distortion. |
ZOE impression paste | Immersion preferred; spraying can be used for bite registrations | Glutaraldehydes or iodophors | Not compatible with chlorine compounds! Phenolic spray can be used. |
How does the surfactant added to hydrophilize PVS affect the contact angle of water droplets during the setting and pouring of casts with gypsum?
Wettability of Impression Materials and Hydrophilization of PVS
The contact angles of distilled water on set silicone impressions are approximately 100°, which makes this group of materials the most hydrophobic among all elastomeric impression materials. The use of a more hydrophobic impression material may lead to a high frequency of voids in the cast. It is possible to spray a surfactant on these hydrophobic impressions prior to pouring with gypsum mixtures. A nonionic surfactant may be added to the bulk of the PVS during the manufacturing process, and the resultant material is called a hydrophilized PVS.
In vitro studies of the surface wettability of set impressions typically use distilled water as the probing liquid. The results confirm that there are significant reductions in the contact angles of hydrophilized PVS relative to their nonhydrophilized counterparts. The use of an aqueous solution saturated with CaSO 4 yields similar results. Examination of stone casts confirms that elastomers, which exhibit the least contact angle with water, also produce stone casts with fewer voids.
The use of optical tensiometry, which analyzes the shape of liquid drops on the surface using a video image, allows observation of the contact angles of water droplets on freshly mixed impression materials in real time. Numerous studies have reported a decrease in water contact angles on silicones, hydrophilized PVS, and polyethers over the setting period. This decrease can be as much as 70° for hydrophilized PVS and less for polyether. Chemical analyses of water droplets show that they all acquire substances from their respective impression materials. For hydrophilized PVS, the water droplets absorb a sufficient quantity of surfactant and become a liquid of lower surface tension. The lowering of surface tension makes water droplets spread wider on the surface of PVS, yielding lower contact angles. This observation leads to the hypothesis that hydrophilicity of the PVS does not change much, but the acquired surfactant has reduced the surface tension of the probing water, making it readily spread over the PVS. Some clinical observations seem to support this hypothesis because there is no difference between nonhydrophilized and hydrophilized PVS with respect to the reproducibility of detail on wet surfaces. However, another clinical study reports that hydrophilized PVS yields a significantly higher proportion of void-free impressions than its nonhydrophilized counterpart. For polyethers, the substances acquired by the water droplets represent a low-molecular-weight compound associated with polyethers, and their effect on the surface tension of the water droplet is small. Thus a smaller decrease in the contact angle is observed.
As soon as hydrophilized PVS encounters oral fluids during impression making, it begins to release surfactant to its surroundings. It is known that there is an adequate amount of surfactant trapped on the surface of set PVS impressions, thereby providing hydrophilicity for the pouring of gypsum dies. Whereas one might believe that the decrease of contact angle over the period of setting is equivalent to good wetting of the impression material on moist tooth surfaces, the clinical relevance remains unclear for two reasons. First, the decrease in contact angle may be attributed mainly to the lowering of the surface tension of the water droplets by the surfactant, so the actual improvement in the hydrophilicity of the impression may be much less significant than it appears. Second, there are conflicting clinical observations. This conflict is not surprising when one realizes that some materials contain very hydrophilic surfactants that are readily leached from the surface, whereas others contain surfactants that are balanced in their hydrophilic and lipophilic properties so that the lipophilic end remains buried near the surface while the hydrophilic chain is exposed on the surface. In the latter case, very little leaching occurs.
Effect of Mishandling
The failure to produce an accurate epoxy or gypsum die or cast is more likely associated with an error in handling rather than a deficiency in the properties of the impression material. The common failures experienced with impression materials and their causes are summarized in Table 13-5 . A summary of the characteristics of the elastomeric impression materials is given in Table 13-6 .
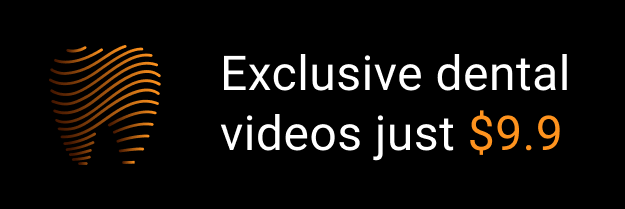