Key Terms
Denture liner The polymeric material used to replace the tissue-contacting (intaglio) surface of an existing denture. A denture liner may be hard or soft, short or long term.
Long-term resilient soft liner A resilient polymeric material that is bonded to the tissue-contacting surface of a denture for cushioning and/or improved retention. Such liners typically are heat-processed, and thus, they are more durable than chemical-activated, short-term soft liners and tissue conditioners.
Rebasing The process of replacing the entire denture base of an existing complete or partial denture.
Relining The process of replacing the tissue-contacting surface of an existing denture.
Resilient soft denture liner A polymeric material that is placed on the tissue-contacting surface of a denture base to absorb some of the mastication impact energy by acting as a type of “shock absorber” between the occlusal surfaces of a denture and the underlying oral tissues. A denture soft liner may also be used to engage natural or prosthetic undercuts so as to provide retention, stability, and support. These materials may be placed chairside (directly) or in the dental laboratory (indirectly).
Short-term soft liner (tissue conditioner) A resilient polymeric material that is employed for brief periods (up to 14 days) to absorb masticatory impact and adapt to changing ridge contours (e.g., following the extraction of teeth or surgical alteration of an edentulous or partially edentulous ridge). Such materials are typically chemical-activated polymers and tend to degrade more rapidly than heat-activated resins.
The Glossary of Prosthodontic Terms, 9th edition, defines a complete denture as a removable dental prosthesis that replaces the entire dentition and associated structures of the maxilla or mandible. Such a prosthesis is composed of artificial teeth attached to a denture base. In turn, the denture base derives its support through contact with the oral tissues, teeth, or dental implants.
Although individual denture bases can be formed from metals or ceramics, the majority of denture bases are fabricated using common polymers. Such polymers are chosen based on availability, dimensional stability, handling characteristics, color, and compatibility with oral tissues.
A description of commonly used denture base polymers is presented in this chapter. Considerable attention is given to individual processing systems and polymerization techniques. In addition, methods for improving the fit and dimensional stability of resin-based prostheses are provided.
Acrylic Resins
Since the mid-1940s, the majority of denture bases have been fabricated using poly(methyl methacrylate) resins, also known as PMMA . PMMA is a colorless transparent solid that can be tinted with pigments, dyes, fillers, and fibers to provide almost any color, shade, and degree of translucency. The color, optical characteristics, and dimensional properties of PMMA remain stable under normal intraoral conditions, and its physical properties have proven to be adequate for dental applications.
One decided advantage of PMMA is the relative ease with which this material can be processed. PMMA denture base material is commonly supplied as a powder–liquid system ( Figure 11-1 ). The liquid contains mostly methyl methacrylate monomers, di- or tri-methacrylate crosslinking agents ( Figure 11-2 ), and an inhibitor, and the powder contains predominantly PMMA resin in the form of micro-sized beads (or spheres). When the liquid and powder are mixed in the proper proportions, a workable mass is formed. The material is introduced into a properly formed mold cavity and polymerized under heat and pressure. Recall from Chapter 2, Chemistry of Polymerization , that polymerization can be activated by chemical, heat, and light energy. Microwave energy may also be used for polymerization. In addition, computer-aided design/computer-aided manufacturing (CAD-CAM) technologies of milling PMMA pucks and three-dimensional (3-D) printing using methacrylate-based monomers ( Chapter 15, Additive Manufacturing—3D printing ) are also available.


General Technique of Denture Base Fabrication
Several processing techniques are available for the fabrication of denture bases ( Figures 11-3 to 11-11 ). Each technique requires a suitable impression of the associated dental arch, followed by the fabrication of an accurate gypsum cast. In turn, a resin record base is fabricated on the cast. Wax is added to the record base, and prosthetic teeth are positioned in the wax ( Figure 11-3, A ). The prosthetic teeth are related to the opposing dentition and evaluated in the patient’s mouth before proceeding.

In the laboratory, a bronze denture flask is used, and the completed tooth arrangement is encased in a suitable investing medium (usually gypsum). A plastic denture flask is mandatory if microwave energy is used. Subsequently, the denture flask is opened, and the wax denture base is eliminated in a hot-water bath, leaving the prosthetic teeth in place. After a thorough cleansing of the mold, an appropriate separator is applied to the gypsum surface. In turn, a resin denture base material is introduced into the mold cavity and polymerized under pressure. Following polymerization, the denture is recovered and prepared for clinical placement.
Not all denture base processing requires flasks. When light-activated resin or CAD-CAM processing is used, no flask is needed. In this chapter, we will discuss commonly available denture base materials and the properties and additional applications of denture base resins.
Heat-Activated Denture Base Resins
Heat-activated materials are used in the fabrication of nearly all denture bases. The use of heat-activated polymers involves the preparation of a gypsum mold for compression molding, preparation of denture base resin, and a thermal polymerization using a water bath or microwave oven.
Compression Molding Technique
As a rule, heat-activated denture base resins are shaped via compression molding. Therefore the compression molding technique is described in detail in the following subsections.
Preparation of the Mold
Before mold preparation, prosthetic teeth must be selected and arranged in a manner that will fulfill both esthetic and functional requirements. In turn, the completed tooth arrangement is sealed to the master gypsum cast.
At this stage, the master cast and completed tooth arrangement are removed from the dental articulator ( Figure 11-3, A ). The master cast is coated with a thin layer of separator to prevent adherence of dental gypsum to the master cast during the flasking process. The lower half of a denture flask is filled with freshly mixed gypsum, and the master cast is placed into this mixture. The gypsum is contoured to facilitate wax elimination, packing, and deflasking procedures ( Figure 11-3, B ). Upon reaching the initial set, the gypsum surfaces are coated with an appropriate separator.
The upper half of the selected denture flask is then positioned atop the lower half of the flask. A surface-tension–reducing agent is applied to exposed wax surfaces, and a second mix of dental gypsum is prepared. This increment of gypsum is poured into the denture flask. Care must be taken to ensure that the investing material achieves intimate contact with all external surfaces of the mounted teeth. The investing gypsum is added until all surfaces of the tooth arrangement and denture base are completely covered. Incisal and occlusal surfaces are minimally exposed to facilitate subsequent deflasking ( Figure 11-3, C ). The gypsum is then permitted to set and is coated with a colorless separator.
At this point, an additional increment of gypsum is mixed, and the remainder of the flask is filled. The lid of the flask is gently seated, and the gypsum is allowed to harden ( Figure 11-3, D ).
After the gypsum has hardened, the record base and wax must be removed from the mold. To accomplish this task, the denture flask is immersed in boiling water for 4 minutes. The flask is then removed from the water, and the appropriate segments are separated. The record base and softened wax remain in the lower half of the denture flask, while the prosthetic teeth remain firmly embedded in the investing gypsum of the remaining segment ( Figure 11-3, E ). The record base and softened wax are carefully removed from the surface of the mold. Residual wax is removed from the mold cavity using wax solvent. The mold cavity is subsequently cleaned with a mild detergent solution and rinsed with boiling water.
What is the purpose of a separating medium that is used during denture base fabrication? What is the chemical basis for popular separating media?
Selection and Application of a Separating Medium
The next step in denture base fabrication involves the application of an appropriate separating medium onto the surfaces of the mold cavity. This medium prevents direct contact between the denture base resin and the mold surface. Failure to place an appropriate separating medium can lead to two major difficulties: (1) If water is permitted to diffuse into the denture base resin, the moisture can affect the polymerization rate and the optical and physical properties of the resultant denture base. (2) If dissolved polymer or free monomer soaks into the mold surface, portions of the investing medium can fuse to the denture base, affecting the physical and esthetic properties of processed denture bases.
Currently, the most popular separating agents are water-soluble colloidal alginate solutions. When applied to gypsum surfaces, these solutions produce thin, relatively insoluble calcium alginate films. These films prevent direct contact of denture base resins and the surrounding dental gypsum.
A small amount of separator is dispensed into a disposable container. Then, a fine brush is used to apply the separating medium onto the exposed surfaces of a warm, clean gypsum mold ( Figure 11-3, F ). Separator should not contact the exposed portions of acrylic resin teeth because the separator interferes with chemical bonding between acrylic resin teeth and denture base resins. Subsequently, the mold sections are oriented to prevent “pooling” of separator and permitted to dry.
Preparation of Heat-Activated Denture Resin
As previously noted, most PMMA resin systems include powder and liquid components ( Figure 11-1 ). The powder consists of spheres of PMMA, other copolymer, and a small amount of benzoyl peroxide, termed the initiator. Upon heating, the benzoyl peroxide generates free radicals, which are responsible for initiating polymerization. The liquid is predominantly methyl methacrylate monomer with small amounts of hydroquinone, as an inhibitor to prevent untimely polymerization of the liquid during storage. Inhibitors also retard the curing process and thereby increase working time.
Crosslinking agents, such as glycol dimethacrylate, may be added to the liquid. Glycol dimethacrylate is chemically and structurally similar to methyl methacrylate and can be incorporated into growing methyl methacrylate polymer chains ( Figure 11-2 ). It is important to note that methyl methacrylate possesses one carbon-carbon double bond per molecule, and glycol dimethacrylate possesses two double bonds per molecule. As a result, an individual molecule of glycol dimethacrylate may serve as a “bridge” or “cross-member” linking two growing polymer chains. If sufficient glycol dimethacrylate is included in the mixture, numerous interconnections may be formed. These interconnections yield a netlike structure that provides resistance to deformation and solvent swelling that may be caused by exposure to ethanol in the diet. Crosslinking agents are incorporated into the liquid component at a concentration of 1% to 2% by volume.
Why do denture base resin systems contain polymer beads? How does the inclusion of polymer beads affect volumetric shrinkage?
Polymer-to-Monomer Ratio
A proper polymer-to-monomer ratio is important in the fabrication of well-fitting denture bases with desirable physical properties. Unfortunately, most discussions of the polymer-to-monomer ratio provide little practical information for dental personnel. Furthermore, these discussions do not address the relationships between molecular events and the gross handling characteristics of denture base resins.
As discussed in Chapter 5, Composition and Function , on resin-based composites, the polymerization of denture base resins results in shrinkage. Research indicates that the polymerization of methyl methacrylate monomers to form PMMA yields a 21% decrease in the volume of material. To minimize dimensional changes, resin manufacturers incorporate a significant fraction of polymer beads, like the fillers added in resin-based composites.
When the powder and liquid components are mixed in the proper proportions, a doughlike mass results. Commercial products generally exhibit a polymer-to-monomer ratio of approximately 3:1 by volume. This ratio provides sufficient monomer to thoroughly wet the polymer beads but does not contribute excess monomer that would produce increased polymerization shrinkage. Using a 3:1 ratio, the volumetric shrinkage can be limited to approximately 7% (about 2.3% linear shrinkage). Polymerization shrinkage is compensated by the initial setting expansion of the gypsum mold and swelling of the polymerized acrylic resin upon exposure to water in the denture flask and in clinical service. Consequently, the completed prosthesis displays little dimensional change.
What are the five physical stages through which properly mixed denture base resins pass? During which stage should compression molding be initiated?
Polymer-Monomer Interaction
When monomer and polymer are mixed in the proper proportions, a workable mass is produced. Upon standing, without subjecting to heat for initiation of polymerization, the resultant mass passes through five distinct stages. These stages can be described as (1) sandy, (2) stringy, (3) doughlike, (4) rubbery or elastic, and (5) stiff.
During the sandy stage, little or no interaction occurs on a molecular level. Polymer beads remain unaltered, and the consistency of the mixture can be described as “coarse” or “grainy.” Later, the mixture enters a stringy stage when the monomer attacks the surfaces of individual polymer beads and is absorbed into the beads, causing swelling of the beads and dissolution of these non-crosslinked polymer chains into the liquid monomer. These polymer chains uncoil, thereby increasing the viscosity of the mix. This stage is characterized by “stringiness” or “stickiness” when the material is touched or drawn apart.
Subsequently, the mass enters a doughlike stage. On a molecular level, an increased number of polymer chains enter the solution. The mixture may be envisioned as polymeric strands within a volume of liquid monomer. (Visualize strands of cooked spaghetti in a pot containing water.) Clinically, the mass behaves as a pliable dough. It is no longer tacky and does not adhere to the surfaces of the mixing vessel or spatula. This is the ideal consistency for compression molding. As a result, the material should be introduced into the mold cavity during the latter phases of the doughlike stage.
Following the doughlike stage, the mixture enters a rubbery or elastic stage. This is because some monomer is dissipated by evaporation, and some penetrates further into remaining polymer beads. The mass rebounds when compressed or stretched. Because the mass no longer flows freely to assume the shape of its container, the rubbery acrylic is not moldable by conventional compression techniques.
Upon standing for an extended period, the mixture becomes stiff. This is the result of the evaporation of unreacted monomer. From a clinical standpoint, the mixture appears dry and is resistant to mechanical deformation.
Dough-Forming Time
The time required for the resin mixture to reach a doughlike stage is termed the dough-forming time. American National Standards Institute/American Dental Association (ANSI/ADA) Standard No. 139—Dental Base polymers: 2020 (ISO 20795-1:2013 Dentistry—Base polymers—Part 1: Denture base polymers) requires that this consistency be attained in less than 40 minutes from the start of the mixing process. In clinical use, the majority of denture base products reach a doughlike consistency in less than 10 minutes.
Working Time
Working time is defined as the time a denture base material remains in the doughlike stage. This period is critical to the compression molding process. ANSI/ADA Standard No. 139 requires the dough to remain moldable for at least 5 minutes.
Ambient temperature affects the working time. Hence the working time of a denture resin can be extended via refrigeration. A significant drawback associated with this technique is that moisture may condense on the resin when it is exposed to ambient air. The presence of moisture may degrade the physical and esthetic properties of a processed resin. Moisture contamination can be avoided by storing the resin in an airtight container. Following removal from the refrigerator, the container should not be opened until it reaches room temperature.
Packing
The introduction of denture base resin into the mold cavity is termed packing. This process represents critical steps in denture base fabrication. While in a doughlike state, the resin is removed from its mixing container and rolled into a cylindrical form. Monomer is painted over the necks of the denture teeth to promote bonding to the denture base. Subsequently, the resin form is bent into a horseshoe shape and placed into the portion of the flask that houses the prosthetic teeth ( Figure 11-4, A ). A thin polyethylene packing sheet is placed over the master cast, and the flask is reassembled.

The flask assembly is placed into a press, and pressure is applied incrementally ( Figure 11-4, B ). Slow application of pressure permits the resin dough to flow uniformly throughout the mold space. The application of pressure is continued until the denture flask is fully closed. In turn, the flask is opened, and the polyethylene packing sheet is removed from the surface of the resin with a rapid, sustained tug.
Excess resin will be found on the relatively flat areas surrounding the mold cavity. This excess resin is called flash. Using a rounded instrument, the flash is carefully teased away from the body of resin that occupies the mold cavity ( Figure 11-4, C ). Care is taken not to chip the gypsum surfaces of the mold. Pieces of gypsum that have become dislodged must be removed so that they are not incorporated into the processed denture base.
A fresh polyethylene packing sheet is placed between the major portions of the flask, and the flask assembly is once again placed in the press. Trial closures are repeated until minimal flash is observed.
At this stage, the mold is closed for the last time with no packing sheet interposed. The mold sections are aligned and placed in the flask press. Again, pressure is incrementally applied. Following definitive closure, the flask is transferred to a flask carrier ( Figure 11-4, D ), which maintains pressure on the flask assembly during denture base processing. A cross-sectional representation of the denture flask assembly is presented in Figure 11-5 .

Injection Molding Technique
In addition to the compression molding techniques, denture bases can be fabricated via injection molding using specially designed flasks. One half of the flask is filled with freshly mixed gypsum, and the master cast is settled into this mixture. The gypsum is appropriately contoured and permitted to set. Subsequently, sprues or ingates are attached to the wax denture base to introduce resin during the injection-molding process ( Figure 11-6, A ). The remaining half of the flask is positioned, and the investment process is completed ( Figure 11-6, B ). Wax elimination is performed as previously described ( Figure 11-6, C ), and the flask is reassembled. Subsequently, the flask is placed into a carrier that maintains pressure on the assembly during resin introduction and processing.

Upon completion of the foregoing steps, resin is mixed and injected into the mold cavity ( Figure 11-6, D ). The flask is then placed into a water bath for polymerization of the denture base resin if a heat-curing resin is used. Upon completion, the denture is recovered, adjusted, finished, and polished.
Currently, there is some debate regarding the comparative accuracy of denture bases fabricated by compression molding and those fabricated by injection molding. Available data and clinical information indicate that denture bases fabricated by injection molding can provide slightly improved clinical accuracy.
Polymerization Procedure
During denture base fabrication, heat is applied to the resin by immersing the denture flask and flask carrier in a water bath. The water is heated to a prescribed temperature and maintained at that temperature for a period suggested by the manufacturer.
Temperature Rise
The polymerization of denture base resins is exothermic, and the amount of heat evolved can affect the properties of processed denture bases. Representative temperature changes occurring in water, investing gypsum, and resin are presented in Figure 11-7 . The temperature profile of the investing gypsum closely parallels the heating curve for the water. The temperature of the denture base resin lags somewhat during the initial stages of the heating process. This is caused by the fact that the resin occupies a position in the center of the mold, and therefore, heat penetration takes longer.

As the denture base resin rises slightly above 70 °C, the temperature of the resin begins to increase rapidly. In turn, the decomposition rate of benzoyl peroxide is significantly increased. This sequence of events leads to an increased rate of polymerization and an accompanying increase in the exothermic heat of reaction.
What causes porosity in denture bases? How can these defects be minimized?
Polymerization Cycle
Controlled heating processes are used to guide the polymerization of heat-activated denture bases. Such heating processes are termed polymerization cycles or curing cycles. The relationship between the heating rate and temperature rise within the denture base resin is illustrated in Figure 11-8 . The polymerization cycle represented by curve C probably would yield porosity in thick portions of the denture because the temperature of the resin exceeds the boiling point of the monomer (100.8 °C). On the other hand, the polymerization cycle represented by curve A probably would result in the presence of residual monomer because the resin temperature does not reach the boiling temperature of the monomer. To minimize the likelihood of undesirable outcomes, carefully controlled heating cycles have been developed.

One technique involves processing the denture base resin in a constant-temperature water bath at 74 °C (165 °F) for 8 hours or longer, with no terminal boiling treatment. A second technique consists of processing in a 74 °C water bath for 8 hours, and then increasing the temperature to 100 °C for 1 hour. A third technique involves processing the resin at 74 °C for approximately 2 hours and increasing the temperature of the water bath to 100 °C and processing for 1 hour. All techniques are commonly used and equally effective.
Following completion of the chosen polymerization cycle, the denture flask should be permitted to cool to room temperature. Rapid cooling can result in distortion of the denture base because of differences in the thermal contraction of resin and investing gypsum. To minimize potential difficulties, the flask should be removed from the water and bench-cooled for 30 minutes. Subsequently, the flask should be immersed in cool tap water for 15 minutes. The denture base may then be deflasked and prepared for delivery. To decrease the probability of unfavorable dimensional changes, the denture should be stored in water until the clinical fitting appointment.
Polymerization via Microwave Energy
PMMA resin may be polymerized using microwave energy because the methyl methacrylate molecule is asymmetrical and therefore “lossy.” This technique employs a specially formulated resin and a nonmetallic flask ( Figure 11-9 ). A conventional 1100-watt, 24-GHz microwave oven is used to supply the thermal energy required for polymerization.

The major advantage of this technique is the speed with which polymerization may be accomplished. Despite the decreased processing time, overheating can occur in thick sections, causing the monomer to boil and yielding porosity. It should also be noted that although the polymerization time is reduced, the required technician time is unaffected. Available information indicates that the physical properties of microwave resins are comparable to those of conventional resins. Furthermore, the fit of denture bases polymerized using microwave energy is comparable to those processed via conventional compression molding techniques.
CAD-CAM Procedures in Denture Base Fabrication
Although the first CAM software program was developed by Dr. Patrick Hanratty in the 1950s, it was not until the 1990s that CAD-CAM technologies were used to generate complete dentures. The first English-language account of computer-aided technology in the fabrication of complete dentures was published by Maeda et al. in 1994. The authors employed stereolithography to generate complete dentures in a proof-of-concept effort. Beginning in 1995, Goodacre employed a series of procedures intended to facilitate the fabrication of complete dentures using subtractive techniques. In the ensuing years, Kawahata et al. and Kanazawa et al. used computer numerical control (CNC) milling to advance and refine previous efforts. CNC is an automated control of machining and 3-D printers by means of a computer. In 2012, Goodacre et al. published the first clinical account of CAD-CAM dentures milled from prepolymerized PMMA.
Advantages associated with CAD-CAM fabrication of complete dentures include enhanced material properties and improved dimensional stability of the resultant prostheses. PMMA “pucks” are fabricated at increased pressures and under strictly controlled thermal conditions. Consequently, these materials display decreased porosity, increased polymerization, and a diminished residual monomer content. Moreover, the prepolymerized resins used in current subtractive processes are approximately 8% more dense than their conventionally processed counterparts. These factors are likely to yield enhanced clinical performance and decreased surface colonization by undesirable species such as Candida albicans.
Machining of prepolymerized PMMA specimens also affects the clinical adaptation and the dimensional stability of completed denture bases. As previously noted, dentures fabricated via compression-molding techniques are subject to polymerization shrinkage during the fabrication process. In addition, compression-molded prostheses are susceptible to distortion upon recovery from the mold. Corresponding changes are not observed in machined specimens because polymerization shrinkage and stress relaxation occur before the machining process. As a result, milled denture bases exhibit improved adaptation to the underlying ridges upon clinical placement and are less susceptible to distortion because relaxation has occurred prior to the machining process.
Currently, the most common CAD-CAM techniques for the fabrication of complete dentures employ milling technologies. Clinicians are responsible for accurately capturing the anatomical ridge forms, identifying critical landmarks (e.g., incisal edge position, facial midline, lip line, etc.), recording desired ridge/arch relationships, and determining appropriate tooth shades and forms. This information is transferred to the dental laboratory or manufacturer responsible for prosthesis fabrication. Design is accomplished in a virtual environment (CAD) and subsequently exported to a milling device for production (CAM).
In the existing environment, dominant systems employ two-component manufacturing processes. The required denture base is manufactured as one component, and the corresponding teeth are manufactured as a separate yet continuous monolithic component. The denture base and tooth forms may be joined in a variety of ways, and the prosthesis is finished, polished, and prepared for clinical placement.
What are the benefits associated with the chemical-activated processes compared with heat-activated processes?
Chemical-Activated Denture Base Resins
The same initiator used in heat-activated denture base resin can also be activated by a tertiary amine, such as dimethyl-para-toluidine. The tertiary amine may be added to the liquid monomer. Upon mixing liquid and powder components, the tertiary amine causes decomposition of the benzoyl peroxide contained in the powder. As a result, free radicals are produced, and polymerization progresses in a manner similar to that described for heat-activated systems. Chemical activation does not require the application of thermal energy and can be completed at room temperature. For this reason, chemical-activated resins often are referred to as cold-curing, self-curing, or autopolymerizing resins.
Technical Considerations
The powder and liquid components are mixed according to the manufacturer’s directions and permitted to attain a doughlike consistency. The working time for chemical-activated resins is shorter than that for heat-activated materials. Therefore special attention must be paid to the consistency of the material and rate of polymerization.
A lengthy initiation period is desirable because this provides adequate time for trial closures of the denture flask. One method for prolonging the initiation period is to decrease the temperature of the resin mass. This may be accomplished by refrigerating the liquid component and/or the mixing vessel before the mixing process. When the powder and liquid are mixed, the polymerization process is slowed, the resin mass remains in a doughy stage for an extended period, and the working time is prolonged.
Mold preparation and resin packing are accomplished in the same manner described for heat-activated resins. In cases of chemical-activated resins with minimal working times, it is doubtful that more than two trial closures can be made. Therefore extreme care must be taken to ensure that a proper amount of resin is employed, and a minimal number of trial closures are needed.
Processing Considerations
Following definitive closure of the denture flask, pressure must be maintained throughout the polymerization process. The time required for polymerization will vary with the material chosen.
Initial hardening of the resin generally will occur within 30 minutes, but polymerization continues for an extended period. To ensure sufficient polymerization, the flask should be held under pressure for a minimum of 3 hours.
The polymerization of chemical-activated resins is never as complete as the polymerization of heat-activated materials. Resins polymerized via chemical activation generally display 3% to 5% free monomer, whereas heat-activated resins exhibit 0.2% to 0.5% free monomer. Therefore it is important that the polymerization of chemical-activated resins be as complete as possible. Failure to achieve a high degree of polymerization will predispose the denture base to dimensional instability and can lead to tissue irritation.
Heat-Activated Versus Chemical-Activated Denture Base
The residual monomer in chemical-activated denture base materials creates two difficulties. First, the residual monomer acts as a plasticizer, resulting in decreased transverse strength of the denture resin. Second, the residual monomer serves as a potential tissue irritant, thereby compromising the biocompatibility of the denture base.
From a physical standpoint, chemical-activated resins display slightly less shrinkage than their heat-activated counterparts. This imparts greater dimensional accuracy to chemical-activated resins.
The color stability of chemical-activated resins generally is inferior to the color stability of heat-activated resins. This property is related to the presence of tertiary amines within the chemical-activated resins. Such amines are susceptible to oxidation and accompanying color changes that affect the appearance of the resin. Discoloration of these resins can be minimized via the addition of stabilizing agents that prevent such oxidation.
Fluid-Resin Technique
The fluid-resin technique employs a pourable, chemical-activated resin for the fabrication of denture bases. When mixed in the proper proportions, a low-viscosity resin is produced. This resin is poured into a mold cavity, subjected to increased atmospheric pressure, and allowed to polymerize at ambient temperature. Laboratory aspects of the fluid-resin technique are described in the following paragraphs.
Tooth arrangement is accomplished using accepted prosthodontic principles. The completed tooth arrangement is then sealed to the underlying master cast and placed in a specially designed flask ( Figure 11-10, A ). The flask is filled with a reversible hydrocolloid-based investment medium, and the assembly is cooled. Following gelation of the hydrocolloid, the master cast with the attached tooth arrangement is removed from the flask ( Figure 11-10, B ). At this stage, sprues and vents are cut from the external surface of the flask to the mold cavity ( Figure 11-10, C ).
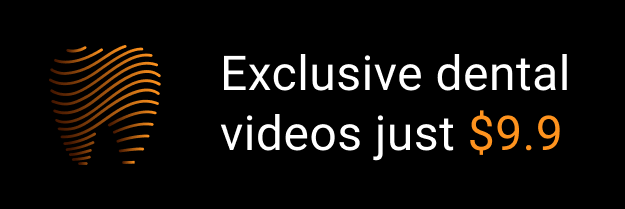