Introduction
In addition to their cholesterol-lowering effects, the statin class of drugs appears to enhance osteogenesis and suppress bone resorption, which could be a clinical concern during orthodontic treatment. In this animal study, we aimed to determine whether atorvastatin (ATV) affects orthodontic tooth movement (OTM) through osteoclast inhibition. Furthermore, we analyzed the potential adverse effects of ATV on long-bone turnover and endochondral ossification.
Methods
Rats were administered ATV (15 mg/kg) or saline solution via gavage (n = 12 animals/group), starting 2 weeks before initial OTM. Tooth displacement was measured after 7, 14, and 21 days. Histologic sections of the maxilla and femur were obtained after 14 and 21 days of OTM and stained (hematoxylin and eosin; TRAP assay) for histomorphometric analysis.
Results
ATV was associated with significant ( P <0.05) reductions in OTM and osteoclast counts. Independently of drug administration, OTM increased the number of osteoclasts and reduced the bone-volume ratio compared with the control maxillae without OTM. Long-term statin administration did not appear to affect femoral endochondral ossification.
Conclusions
This experimental study showed that the long-term use of ATV can significantly promote osteoclast inhibition and slow the OTM in the first week in rats. Under physiologic conditions, the drug did not affect bone turnover and endochondral ossification.
Highlights
- •
Atorvastatin transiently affects orthodontic tooth movement.
- •
During orthodontic movement, atorvastatin inhibits osteoclastogenesis transiently.
- •
Pharmacologic bone modulation could be a strategy to regulate tooth movement.
- •
Long-term atorvastatin administration did not affect endochondral ossification.
Epidemiologic studies have shown a significant increase in the prevalence of diseases such as obesity and hyperlipidemia in adults, with the latter regarded as the main cause of coronary atherosclerosis. According to Mercado et al, 36.7% of adults, or 78.1 million persons aged 21 years or over, in the United States alone were on or eligible for lipid-lowering treatment; 55.5% of them were taking cholesterol-lowering medications. The drugs most widely used for this purpose are the statins, which lower cholesterol levels by inhibiting hydroxymethylglutaryl-coenzyme A reductase, the rate-controlling enzyme of the mevalonate pathway. Studies suggest that, in addition to their cholesterol-lowering effects, statins may influence bone turnover, enhancing osteogenesis and suppressing bone resorption. Attempts to ascertain the mechanism of statin-regulated bone anabolism have suggested 3 aspects: promotion of osteogenesis, inhibition of osteoblast apoptosis, and suppression of osteoclastogenesis. Although the effects of statins on bone anabolism have been widely demonstrated in laboratory studies, their clinical effects are not convincing.
The biologic reaction of periodontal tissues during orthodontic tooth movement (OTM) is characterized by an aseptic acute inflammatory response in the early stages, followed by a transient, aseptic chronic inflammation. Chemokines, cytokines, and growth factors are the main molecules that orchestrate this inflammatory response, which is followed by osteoclastogenesis and bone resorption, and then by osteoblast and new bone formation. Current biologic knowledge raises the possibility that pharmacologic modulation of these periodontal cellular and molecular responses may affect OTM, as shown in various experimental models. Research has demonstrated that statins reduce OTM and relapse; however, the biologic mechanisms underlying these clinical effects are unknown. Since statins are among the most commonly prescribed pharmaceutic agents for prevention of cardiovascular diseases, their plausible effect of arresting tooth movement could be a concern in orthodontic practice.
Clinical trials have shown that statins are well tolerated in adult and younger populations, but long-term data regarding the impact of statin therapy on growth and development are limited. In a study by Macpherson et al, treatment of children and adolescents with statins over 2 years had no impact on height, weight, body mass index, and sexual maturation. On the other hand, preclinical studies have suggested that these drugs increase chondrocyte proliferation and longitudinal bone growth, which could have implications for their clinical use in the pediatric population. These discrepancies between clinical trials, in-vitro experiments, and in-vivo studies are puzzling, and further research is necessary to investigate the potential adverse effects of statins on bone turnover and endochondral ossification.
In an attempt to mimic the clinical perspective of orthodontic treatment in patients taking statins, we developed an experimental animal model in which high-dose statin administration was started before OTM, simulating a protocol used in humans. We hypothesized that atorvastatin (ATV) treatment in rats might reduce OTM by inhibiting osteoclastogenesis. Furthermore, we analyzed its potential adverse effects on long-bone turnover and endochondral ossification.
Material and methods
Twenty-four male Wistar rats (age, 6 weeks; weight, approximately 330-340 g) was the sample size estimated by the resource equation method. They were housed four to a cage, under a 12-hour light and dark cycle, at a controlled ambient temperature of 23°C, and given food and water ad libitum. All animal care and use procedures were conducted in keeping with the internationally accepted guidelines in the National Institutes of Health Guide for the Care and Use of Laboratory Animals and were approved by the relevant institutional ethics committee of the School of Dentistry of Federal University of Rio Grande do Sul (number 28401) in Brazil.
The animals were randomly divided into 2 groups: experimental (ATV, n = 12) and control (saline solution [SAL], n = 12). Those in the experimental group received atorvastatin (ATV; Medley Farmacêutica, Suzano, Brazil), 15 mg per kilogram daily via gavage, and those in the control group received 0.1 mL of phosphate-buffered saline solution via the same route. Saline solution or drug administration continued until the animals were killed ( Fig 1 ).

After 14 days of saline solution or drug administration, the animals were anesthetized with ketamine and xylazine (80 mg/kg and 5 mg/kg, respectively) for orthodontic appliance placement. This procedure consisted of placing a superelastic nickel-titanium closed-coil spring between the maxillary right first molar and incisors, as described elsewhere. Our protocol was based on previous studies demonstrating that 50 cN of force is sufficient to provide substantial OTM. The device was kept in place for 21 days ( Fig 1 ) to generate mesial displacement of the first molar. In a split-mouth design, the maxillary right side of each rat served as the experiment (OTM), and the maxillary left side, without OTM, served as internal control. Throughout the study, the animals were evaluated weekly for weight gain or loss, appliance breakage, and gingival or other soft tissue inflammation.
Precise plaster models of the maxilla were obtained from impressions made with silicone material (Perfil; Vigodent, Rio de Janeiro, Brazil) and dental stone (Durone; Dentsply Sirona, York, Pa). Impressions were made every 7 days under anesthesia ( Fig 1 ). The occlusal surfaces were photographed (DSC-H10; Sony, Tokyo, Japan) at 300 dpi and magnified (4 times) in ImageJ software (version 1.44; National Institutes of Health, Bethesda, Md). For each photograph, a 100-mm ruler was placed next to the cast to calibrate measurements. The mean distance between the distal surface of the first molar and the mesial surface of the second molar, measured at 3 points on each cast, was calculated for each animal and averaged for the 2 groups. The validity of these data was confirmed by comparing them with the data from a well-described methodology, carried out by measuring the distance between the first and second molars with a digital caliper. The intraclass correlation between both methods was considered satisfactory (>0.8).
Tooth displacements were evaluated after 7, 14, and 21 days of OTM; histologic analysis was done 14 and 21 days after orthodontic appliance insertion, as shown in Figure 1 .
At each time point of analysis (days 14 and 21), 12 animals, 6 per group, were killed by overdose of ketamine and xylazine. Maxillae and distal left femurs were immediately resected and fixed by immersion in 10% buffered formalin for 24 hours. The specimens were demineralized in 10% ethylenediaminetetraacetic acid, pH 7, for 30 to 60 days. Then the samples were dehydrated through an ethanol series, embedded in paraffin, cut into 5-μm longitudinal sections, stained with hematoxylin and eosin, and prepared for a TRAP assay. TRAP is an enzyme expressed in mature osteoclasts and in their precursors, which develop an osteoclastic phenotype at early stages and promote bone resorption. Briefly, for TRAP staining, histologic sections were selected and incubated in acetate buffer (pH, 5.0) containing naphthol AS-MX phosphate (Sigma, St Louis, Mo), Fast Red Violet LB Salt (Sigma), and 50 mmol per liter of sodium tartrate. The sections were counterstained with hematoxylin.
Histomorphometric analyses of the maxilla specimens were performed with 4 subgroups: ATV + OTM, experimental hemimaxillae (with OTM) from animals in the ATV group; control ATV, control hemimaxillae (without OTM) from animals in the ATV group; SAL + OTM, experimental hemimaxillae from animals in the SAL group; and control SAL, control hemimaxillae from animals in the SAL group. Comparisons across subgroups were performed at each time point of analysis (14 and 21 days) or by analysis of the overall mean. For evaluation of femur specimens, the sample was divided into 2 groups: ATV (n = 18) and SAL (n = 18). In this case, between-group comparisons were done considering overall mean values.
Hematoxylin and eosin and TRAP slides were visualized in an Eclipse 90i microscope (Nikon, Tokyo, Japan) coupled to a Coolsnap EZ camera (Photometrics, Tucson, Ariz). Microphotographs were captured using NIS Elements Imaging 3.10 Sp2 software (Nikon). All histomorphometric measurements and terminology were in accordance with the American Society for Bone and Mineral Research recommendations.
For maxillary bone turnover, the supporting structures of the molars were evaluated using the NIS Elements Imaging 3.10 Sp2 software (Nikon). Under high magnification (100 times), the number of osteoclasts was counted on the most mesial root of the first molars. The region of interest was the periodontal tissue at the distal surface of the mesial root of the first molar. The mesial root of the first molar was chosen because it is the largest of the 5 roots of rat teeth, thus allowing histologic evaluation of the entire root structure. Furthermore, it is commonly used for analysis in tooth-movement studies. According to Gonzalez et al, during OTM in rats, a major stress occurs in the periodontal tissues adjacent on the middle part of the mesial root. Then we tend to argue that the region of interest could represent an important remodeling zone during OTM. Moreover, in a previous study in our laboratory, a high level of data reproducibility of this histologic field (osteoclast number and bone volume) was verified. Cells were considered to be osteoclasts if they were TRAP-positive, multinucleated, and located on the bone surface or in Howship’s lacunae.
The maxillary bone-volume ratio, expressed as the ratio of cancellous bone volume to total tissue volume, was defined in the region of interest of the stained sections using Photoshop (CS6; Adobe Systems, San Jose, Calif) and ImageJ software, following the method suggested by Egan et al. The total osteoclast count and bone volume were recorded for each animal and then averaged for each group.
For femoral bone turnover, using NIS Elements Imaging 3.10 Sp2 software, the number of osteoclasts was counted in 10 fields chosen randomly in the metaphyseal region of the femur. The bone-volume ratio of 5 rectangular areas of subchondral bone tissue was calculated according to the protocol outlined by Ho et al. The total number of osteoclasts and the bone-volume ratio were recorded for each animal and then averaged for each group, For endochondral ossification, growth-plate cartilage and hypertrophic zone thickness were calculated as the means of 10 measurements obtained at randomly chosen locations in the hematoxylin and eosin-stained sections, again using NIS Elements Imaging 3.10 Sp2 software.
All histologic sections and plaster models were identified with a random numeric sequence to codify experimental periods and groups. The acquired digital images were analyzed by an examiner (G.S.D.) blinded to the experimental groups and periods. To obtain data reproducibility, a calibration process was performed in 10% of the specimens (plaster models and histologic sections), randomly chosen and evaluated twice, with a 15-day interval between the first and second evaluations. Then the random error was assessed according to Dahlberg’s equation, S2 = Ʃ2d/2n, where S2 is the error variance, and d is the difference between 2 determinations of the same variable. When acceptable values were obtained (<10%), the examiner was considered to be accurate, and the measurements were performed in the overall samples.
Statistical analysis
Data are presented as means and standard deviations or standard errors as appropriate. A linear mixed model with repeated measures for the factor of time was used to evaluate the main effects and interactions of group (ATV or SAL) and time (days 7, 14, and 21) on OTM. Between-group comparisons of maxillary histomorphometric parameters were performed using 1-way analysis of variance followed by the LSD multiple comparison test (for homogeneous variances) or the Games-Howell test (for heterogeneous variances). When comparisons were made between 2 groups, the independent Student t test was used. Results were processed in Statistics for Windows (version 18.0; SPSS, Chicago, Ill), and the significance level was set at 5% ( P <0.05) for all tests.
Results
There was no difference in weight changes between groups during the experimental period. The mean weights at the end of the study were 327.18 g (SD, ±86.54 g) in the SAL animals and 344.56 g (SD, ±55.44 g) in ATV animals.
After 7, 14, and 21 days of OTM, the first molar displacements were 310.13 ± 14.79, 381.38 ± 33.33, and 485.85 ± 68.94 μm, respectively, in the SAL group vs 263.53 ± 14.79, 334.78 ± 33.33, and 439.25 ± 68.94 μm, respectively, in the ATV group. Statistical analysis indicated that administration of ATV decreased tooth movement significantly ( P <0.05), as shown in Figure 2 , A and B . From day 0 to day 7, the rate of OTM decreased in the ATV group, compared with the SAL group (263.53 vs 310.13 μm, respectively). After that, this rate increased progressively in the SAL and ATV groups (176 vs 175 μm from days 7 to 21, respectively). From day 0 to day 7, the rate of tooth movement decreased in the ATV group, compared with the SAL group (263.53 vs 310.13 μm, respectively). After that, this rate increased progressively in the SAL and ATV groups (176 vs 175 μm from days 7 to 21, respectively).

During OTM, ATV administration reduced the overall osteoclast count but did not affect the overall bone-volume ratio compared with SAL ( Fig 2 , C and D ). However, when analyzing the drug effect at each time point separately, only after 14 days of OTM did ATV promote osteoclast inhibition ( Fig 3 , A and B ). At this time point, the number of TRAP-positive cells in the ATV group was significantly smaller than that in experimental maxillae of the SAL animals (with OTM) and equal to that observed in control maxillae of the SAL animals (without OTM). During OTM, there was a trend toward increased osteoclast counts only in the SAL group when compared with its respective control maxillae (without OTM), as shown in Figure 3 , A and B . After 21 days, there were no statistical differences in osteoclast count across study groups.

Regarding osteoclast distribution, our data indicated that, independently of drug or saline solution administration, these cells were observed in the periphery around bone blood vessels (Haversian canals) or scattered through the periodontal ligament (PDL) ( Fig 3 , A ). After 14 days, chronic inflammatory cells were observed in the region of interest; after 21 days, there appeared to be resolution of the cellular infiltrate, with increasing amounts and maturation of bone. However, in some specimens, the width of the PDL remained enlarged, especially in the SAL group ( Fig 3 , C ).
After 14 and 21 days of OTM, the bone-volume ratios were significantly reduced in the SAL and ATV groups compared with their respective controls (without OTM). However, after 14 days of OTM, the bone-volume ratio in the ATV group was statistically similar to that in the control maxillae of the SAL group, as shown in Figure 3 , C and D . Without orthodontic force (maxillae without OTM), osteoclast count and bone volume were statistically similar between the ATV and SAL groups ( Fig 3 , B and D ).
ATV did not affect long-bone turnover or endochondral ossification. As shown in the Table , ATV did not affect femur osteoclastogenesis or bone volume. Growth plate cartilage and hypertrophic zone thickness were increased in the ATV group when compared with the SAL group; however, the differences between the groups were not significant ( P = 0.259 and P = 0.09, respectively).
ATV | SAL | P | |
---|---|---|---|
Osteoclasts number | 26.33 ± 9.14 | 20.25 ± 8.90 | NS |
Bone-volume ratio (%) | 27.10 ± 9.91 | 20.97 ± 7.73 | NS |
Thickness of hypertrophic zone (μm) | 67.20 ± 12.09 | 58.25 ± 12.64 | NS |
Thickness of growth plate cartilage (μm) | 136.70 ± 24.50 | 124.29 ± 27.81 | NS |
Results
There was no difference in weight changes between groups during the experimental period. The mean weights at the end of the study were 327.18 g (SD, ±86.54 g) in the SAL animals and 344.56 g (SD, ±55.44 g) in ATV animals.
After 7, 14, and 21 days of OTM, the first molar displacements were 310.13 ± 14.79, 381.38 ± 33.33, and 485.85 ± 68.94 μm, respectively, in the SAL group vs 263.53 ± 14.79, 334.78 ± 33.33, and 439.25 ± 68.94 μm, respectively, in the ATV group. Statistical analysis indicated that administration of ATV decreased tooth movement significantly ( P <0.05), as shown in Figure 2 , A and B . From day 0 to day 7, the rate of OTM decreased in the ATV group, compared with the SAL group (263.53 vs 310.13 μm, respectively). After that, this rate increased progressively in the SAL and ATV groups (176 vs 175 μm from days 7 to 21, respectively). From day 0 to day 7, the rate of tooth movement decreased in the ATV group, compared with the SAL group (263.53 vs 310.13 μm, respectively). After that, this rate increased progressively in the SAL and ATV groups (176 vs 175 μm from days 7 to 21, respectively).
During OTM, ATV administration reduced the overall osteoclast count but did not affect the overall bone-volume ratio compared with SAL ( Fig 2 , C and D ). However, when analyzing the drug effect at each time point separately, only after 14 days of OTM did ATV promote osteoclast inhibition ( Fig 3 , A and B ). At this time point, the number of TRAP-positive cells in the ATV group was significantly smaller than that in experimental maxillae of the SAL animals (with OTM) and equal to that observed in control maxillae of the SAL animals (without OTM). During OTM, there was a trend toward increased osteoclast counts only in the SAL group when compared with its respective control maxillae (without OTM), as shown in Figure 3 , A and B . After 21 days, there were no statistical differences in osteoclast count across study groups.
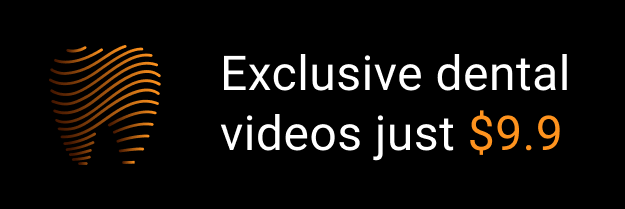