Abstract
The aim of this study was to investigate the capacity of human dental follicle cells (hDFCs) for bone formation in vivo. hDFCs were obtained from wisdom teeth extracted from patients aged 14 and 22 years. hDFCs from the 5th to 8th passages were grown in three-dimensional (3D) culture using gelatin sponges. Cells were transplanted onto the calvaria of F344/NJcl-rnu/rnu male rats (immunodeficient rats). Haematoxylin and eosin (HE) staining and immunohistochemistry were performed, and newly formed bone was evaluated by micro-computed tomography (micro-CT). HE staining showed newly formed bone in 3D culture. Immunohistochemistry showed bone morphogenetic protein 2 (BMP-2), runt-related transcription factor 2 (RUNX2), and osterix staining in areas with newly formed bone. Furthermore, micro-CT showed that, in comparison to controls, transplanted hDFCs promoted better bone quality and bone mineral density (BMD 582 ± 131.1 vs. 300.5 ± 77.7 mg/cm 3 ; P = 0.039), bone mineral content (BMC 5.6 ± 1.1 vs. 2.1 ± 0.4 mg; P = 0.006), bone volume (BV 9.7 ± 0.5 × 10 −3 vs. 7.0 ± 0.4 × 10 −3 cm 3 ; P = 0.002), BMC/total volume (TV) (399.9 ± 76.3 vs. 147.7 ± 30.8 mg/cm 3 ; P = 0.006), and BV/TV (69.1 ± 3.6% vs. 49.6 ± 3.1%; P = 0.002). This suggests that human dental follicles are potentially useful for regenerative therapy.
The management of traumatic bone loss and reconstruction of segmental mandibular defects remains a challenge in the clinical setting. The reconstruction of large bone defects is performed widely, using either autografts or allografts. Autologous bone grafting promotes osteoinduction and functions as a scaffold for bony ingrowth. However, this procedure is limited by donor site morbidity and the amount of tissue that can be harvested. As a clinically effective bone regeneration strategy, stem cells represent a promising alternative for regenerative therapy. Human dental follicles are an ectomesenchymally derived connective tissue surrounding the tooth germ and contain stem cells of the periodontium. Human dental follicles (hDFCs) have the capacity to mineralize under in vitro culture conditions with osteogenic induction medium (OIM). Dental follicles are typically discarded after the extraction of impacted third molars and may be useful as a source of cells in regenerative therapy.
Dexamethasone (DEX) has been shown to have contrasting actions. DEX is typically added to the cell culture for osteoblast differentiation from mesenchymal stem cells. We previously reported that hDFCs cultured in OIM DEX(+) were stained with alizarin red S, and found that alkaline phosphatase (ALP) activity increased on culture with OIM DEX(+) when compared with cultures on growth medium (GM) in vitro. However, DEX also has negative effects on osteoblast differentiation and mineralization. The negative effects of DEX on bone formation result in preferential adipogenic differentiation of mesenchymal stem cells, inducing osteoporosis. This suggests that DEX plays a major role in the pathogenesis of osteonecrosis. A recent study reported that hDFCs have a unique potential for differentiation into osteoblast lineage cells without DEX in vitro.
The aim of this study was to investigate the capacity of hDFCs for mineralization after culture without DEX for bone regeneration therapy.
Materials and methods
Cell cultures
hDFCs were obtained from patients aged 14 and 22 years after obtaining informed consent. hDFCs were obtained using a previously reported method. In brief, normal human impacted third molars were surgically removed and collected. Dental follicle tissues were digested in a solution of 0.1 U/ml collagenase type I and 1 U/ml dispase (Roche, Mannheim, Germany) for 1 h at 37 °C. Attached hDFCs were cultured at 37 °C in 100-mm dishes using mesenchymal stem cell growth medium (GM; consisting of mesenchymal stem cell basal medium supplemented with foetal bovine serum (FBS), l -glutamine, and penicillin/streptomycin; Lonza, Walkersville, MD, USA) in a humidified incubator (CO 2 incubator MCO-175M; Sanyo, Osaka, Japan) in the presence of 5% CO 2 in air. Experiments using hDFCs were performed in accordance with the guidelines established by the ethics committee of the study institution.
Three-dimensional culture
hDFCs obtained from the 5th to 8th passages were used in the experiments. Gelatin sponges containing β-tricalcium phosphate (MedGel Scaffold; MedGel, Kyoto, Japan) were used for three-dimensional (3D) culture, as described previously. In brief, the scaffold was washed with phosphate buffered saline (PBS) and cell culture medium before use. hDFCs were suspended in GM at 5.0 × 10 5 cells/ml, and the hDFC suspension (25 μl) was then placed on the scaffold in 96-well plates. GM or osteoinduction medium without dexamethasone (OIM DEX(−); mesenchymal stem cell basal medium and osteogenic SingleQuots consisting of FBS, l -glutamine, penicillin/streptomycin, ascorbate, and β-glycerophosphate; Lonza) was added after 1 h, and cells were cultured in a humidified incubator in the presence of 5% CO 2 in air at 37 °C.
Transplantation procedure
The transplantation procedure was designed to evaluate bone formation and vascularization of hDFCs in vivo. F344/NJcl-rnu/rnu male rats (immunodeficient rats, age 9 weeks, weight 200–230 g) were purchased from CLEA Japan Inc. (Tokyo, Japan). Rats were assigned randomly to two groups ( n = 3 per group). Group 1 was the control group: hDFC 3D culture with GM (weight 220.2 ± 8.5 g). Group 2 was the OIM DEX(−) group: hDFC 3D culture with OIM DEX(−) (weight 228.3 ± 3.0 g). All animals were anaesthetized with an intraperitoneal injection of 25 mg/kg pentobarbital sodium (Somnolently R; Kyoritsu Seiyaku, Tokyo, Japan) and the surgical site was prepared aseptically. Transplantation of the 3D culture within a polytetrafluoroethylene (PTFE) tube (inner diameter 3 mm, outer diameter 4 mm, and height 2 mm) into the rats was performed as described previously. In this experimental model, an interpositional space was prepared with a PTFE tube immediately below the periosteum. This allowed observation of newly formed bone from both the periosteum and cortical bone sides because the tube space was intercepted by the surrounding tissues. A linear skin incision was made along the edge of the skull with a number 15 surgical blade. The periosteum of the calvaria was carefully removed with a periosteal raspatory after elevation of the skin–periosteum flap. Next, the 3D cultures were inserted into a PTFE tube and placed on the calvarial surface. The overlying tissue was sutured closed.
All animals were treated in accordance with the university animal use guidelines.
Histological analysis
Rats were sacrificed at 28 days after surgery. The calvarial bone area including the PTFE tube was extirpated en-bloc. Excised samples were fixed in 10% neutral-buffered formalin solution, decalcified in 10% ethylenediaminetetraacetic acid (EDTA), and embedded in paraffin. Semi-serial sections (4 μm) were obtained in a sagittal orientation. Sections were stained with haematoxylin and eosin (HE) and observed by light microscopy.
Immunohistochemical analysis
For both groups, sections of the calvarial bone area, including the PTFE tube, were stained immunohistochemically. Sections were deparaffinized and endogenous peroxidase activity was quenched by incubating in 3% H 2 O 2 in methanol for 15 min at room temperature. Sections were then washed with PBS, and were incubated with mouse monoclonal antibody against rat bone morphogenetic protein 2 (BMP-2; 1:500) (ab6285; Abcam, Tokyo, Japan), rabbit polyclonal antibody against rat runt-related transcription factor 2 (RUNX2; 1:500) (ab23981; Abcam), mouse monoclonal antibody against rat osterix (1:500) (ab22442; Abcam), or rabbit polyclonal antibody against rat vascular endothelial growth factor (VEGF-A20; 1:50) (sc152; SantaCruz, Tokyo, Japan) for 1 h in room air. Secondary antibody staining was performed with reagents from the ChemMate EnVision/HRP kit (diaminobenzidine (DAB); Dako, Tokyo, Japan), as per the manufacturer’s protocols. Immunocomplexes were visualized using 3′,3′-diaminobenzidine, and sections were then counterstained with Mayer’s haematoxylin.
Micro-computed tomography (micro-CT) analysis
Quantitative image analysis of bone formation was performed using an in vivo micro-CT system (Rigaku-mCT, Tokyo, Japan), as described elsewhere. Rats were deeply anaesthetized and placed on the object stage. Calvarial bone at the PTFE tube was then scanned using a micro-CT system with an X-ray source of 90 kV/88 μA. Imaging of the newly formed bone area containing the inner cavity of the PTFE tube was then performed over a full 360° rotation, with an exposure of 17 s. An isotropic resolution of 20 μm × 20 μm × 20 μm voxels was selected. Original 3D images were displayed and analyzed with i-View software (J. Morita, Kyoto, Japan). Analysis of new bone formation was measured, and structural indices of the newly formed bone were calculated using a morphometric programme (TRI/3D-BON; Ratoc System Engineering, Tokyo, Japan). 3D analysis directly measured total volume (TV, cm 3 ), bone volume (BV, cm 3 ), and bone mineral content (BMC, mg) of the new bone formation in the inner cavity of the PTFE tube. Volumetric density (BV/TV, %) and bone mineral density (BMD = BMC/BV, mg/cm 3 ) were calculated. The quantity of new bone was evaluated using the ratio of bone volume to total volume (BV/TV) and the density of the new bone was calculated as BMD.
Statistics
Data were expressed as the mean ± standard deviation (SD) of two samples. The significance of differences between culture samples was determined by Welch’s t -test, and a P -value of <0.05 was considered to be statistically significant.
Results
Histological findings
We confirmed new bone formation in vivo. hDFC 3D cultures were placed into PTFE tubes and were transplanted between the periosteum and calvaria. Newly formed bone was seen between the cortical bone and periosteum by HE staining at 28 days after transplantation ( Fig. 1 A–D) . Many woven osteoids, enlarged capillary vessels, and spindle-shaped cells were observed among the scaffold layers ( Fig. 1 C and D). Similarly, osteoblasts were observed around osteoids ( Fig. 1 C and D).
Micro-CT analysis
Micro-CT was used to quantify the mineralization of the scaffolds within the PTFE tubes at 28 days after transplantation, allowing assessment of the entire volume of newly formed bone. Colour images showing BMD were obtained by micro-CT analysis. The inner PTFE tube contained newly formed bone on the calvaria side ( Fig. 2 ). A small amount of newly formed bone was seen in the control group (GM culture) on the calvaria side ( Fig. 2 A). A greater area of newly formed bone was seen in the OIM DEX(−) culture group ( Fig. 2 B).
We next analyzed the BMD, BMC, BV, BMC/TV, and BV/TV using micro-CT ( Fig. 3 ), and found that the OIM DEX(−) culture group showed significantly higher values than the control (GM culture) group for all items tested.
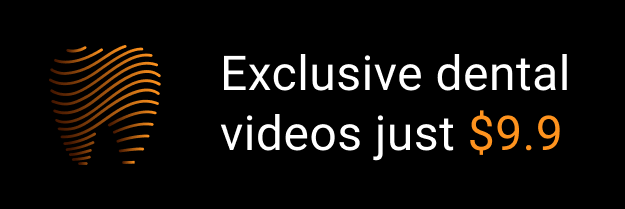