Abstract
Objectives
Calcium and phosphate ion-releasing resin composites are promising for remineralization. However, there has been no report on incorporating antibacterial agents to these composites. The objective of this study was to develop antibacterial and mechanically strong nanocomposites incorporating a quaternary ammonium dimethacrylate (QADM), nanoparticles of silver (NAg), and nanoparticles of amorphous calcium phosphate (NACP).
Methods
The QADM, bis(2-methacryloyloxyethyl) dimethylammonium bromide (ionic dimethacrylate-1), was synthesized from 2-( N , N -dimethylamino)ethyl methacrylate and 2-bromoethyl methacrylate. NAg was synthesized by dissolving Ag 2-ethylhexanoate salt in 2-(tert-butylamino)ethyl methacrylate. Mechanical properties were measured in three-point flexure with bars of 2 mm × 2 mm × 25 mm ( n = 6). Composite disks (diameter = 9 mm, thickness = 2 mm) were inoculated with Streptococcus mutans. The metabolic activity and lactic acid production of biofilms were measured ( n = 6). Two commercial composites were used as controls.
Results
Flexural strength and elastic modulus of NACP + QADM, NACP + NAg, and NACP + QADM + NAg matched those of commercial composites with no antibacterial property ( p > 0.1). The NACP + QADM + NAg composite decreased the titer counts of adherent S. mutans biofilms by an order of magnitude, compared to the commercial composites ( p < 0.05). The metabolic activity and lactic acid production of biofilms on NACP + QADM + NAg composite were much less than those on commercial composites ( p < 0.05). Combining QADM and NAg rendered the nanocomposite more strongly antibacterial than either agent alone ( p < 0.05).
Significance
QADM and NAg were incorporated into calcium phosphate composite for the first time. NACP + QADM + NAg was strongly antibacterial and greatly reduced the titer counts, metabolic activity, and acid production of S. mutans biofilms, while possessing mechanical properties similar to commercial composites. These nanocomposites are promising to have the double benefits of remineralization and antibacterial capabilities to inhibit dental caries.
1
Introduction
Approximately half of all dental restorations fail within 10 years, and replacing them consumes nearly 60% of the average dentist’s practice time . Dental composites are increasingly used due to their excellent esthetics and improved performance . However, composites in vivo had more biofilm and plaque accumulation than other restorative materials . Plaques adjacent to the restoration margins could result in secondary caries and compromise the restoration’s longevity. Indeed, previous reports demonstrated that: (1) the two main challenges facing composite restorations are secondary caries and bulk fracture ; (2) caries at the restoration margins are a main reason for replacing the existing restorations ; (3) replacing the failed restorations accounts for 50–70% of all restorations ; (4) replacement dentistry costs $5 billion in the USA each year . Therefore, there is a great need to improve the longevity of composite restorations by incorporating bioactive agents to combat microbial destruction and recurrent caries while sustaining the load-bearing capability .
Acidogenic bacteria such as Streptococcus mutans ( S. mutans ) and their biofilms, upon exposure to fermentable carbohydrates, are responsible for dental caries . Hence, efforts have been made to develop antibacterial dental composites. One important class of such composites involved the use of polymers containing quaternary ammonium salts (QAS) . QAS are widely used in water treatment, surface coatings, and the food industry due to their low toxicity and potent antimicrobial activity . Antibacterial QAS monomers such as 12-methacryloyloxydodecylpyridinium bromide (MDPB) copolymerize with other monomers in the composite to form polymer matrices that can combat bacteria. The resulting composite decreased the attachment of S. mutans and plaque accumulation, and inhibited the progression of secondary root caries . Recently, a unique QAS dimethacrylate monomer was synthesized and incorporated into dental polymers to effectively reduce S. mutans colonization .
A second class of antibacterial dental composites contain silver (Ag) that can kill oral bacteria such as S. mutans . Ag is known to have antibacterial, antifungal, and antiviral capabilities . For dental composites, it is desirable to incorporate silver nanoparticles with a high surface area into the resin to reduce the Ag particle concentration necessary for efficacy . Thus, it would be possible to obtain a strong antibacterial capability without compromising the composite color and mechanical properties. Indeed, a recent study used a unique approach to prepare nanocomposites with evenly dispersed Ag nanoparticles at a mass fraction of 0.08% to achieve a 40% reduction in bacteria coverage on the nanocomposites .
Another class of dental composites contains calcium phosphate (CaP) particles as a portion of the filler phase for remineralization purposes . These composites released supersaturating levels of calcium (Ca) and phosphate (PO 4 ) ions and remineralized tooth lesions in vitro . Recently, novel CaP nanoparticles of sizes of about 100 nm were synthesized via a spray-drying technique and filled into dental composites . These nanocomposites achieved Ca and PO 4 release similar to those of previous CaP composites, while possessing much better mechanical properties . A literature search revealed no report on combining the best of the three therapeutic approaches: antibacterial monomers, antibacterial Ag nanoparticles, and Ca and PO 4 ion release via CaP nanoparticles. The rationale for this combination was for the composites to not only have a remineralization capability as previous studies have demonstrated, but also to possess strong antibacterial activities.
Therefore, the objective of the present study was to develop novel nanocomposites with ACP and Ag nanoparticles in a QAS-containing resin matrix to achieve caries-inhibiting and stress-bearing capabilities. It was hypothesized that (1) adding QAS or Ag nanoparticles to the ACP nanocomposite would result in significant antibacterial properties; (2) adding QAS and Ag together in the same nanocomposite would achieve even stronger antibacterial capabilities; (3) the novel antibacterial nanocomposites would have mechanical properties matching those of commercial composites without antibacterial capabilities.
2
Materials and methods
2.1
Fabrication of calcium phosphate nanocomposite
A spray-drying technique as described previously was used to make nanoparticles of ACP (Ca 3 [PO 4 ] 2 ). To make nanoparticles of ACP (referred to as NACP), calcium carbonate (CaCO 3 , Fisher, Fair Lawn, NJ) and dicalcium phosphate anhydrous (CaHPO 4 , Baker Chemical Co., Phillipsburg, NJ) were dissolved into an acetic acid solution to obtain final Ca and PO 4 ionic concentrations of 8 mmol/L and 5.333 mmol/L, respectively. This resulted in a Ca/P molar ratio of 1.5, the same as that for ACP. This solution was sprayed into a heated chamber, and an electrostatic precipitator (AirQuality, Minneapolis, MN) was used to collect the dried particles. This produced NACP with a mean particle size of 116 nm, as measured in a previous study .
Two types of co-fillers were used for reinforcement: barium boroaluminosilicate glass particles of a mean diameter of 1.4 μm (Caulk/Dentsply, Milford, DE), and nano-sized silica glass (Aerosil-OX50, Degussa, Ridgefield, NJ) with a mean diameter of 40 nm. Each glass was silanized with 4% 3-methacryloxypropyltrimethoxysilane and 2% n-propylamine (all by mass, unless otherwise noted). A resin of BisGMA (bisphenol glycerolate dimethacrylate) and TEGDMA (triethylene glycol dimethacrylate) at 1:1 mass ratio was rendered light-curable with 0.2% camphorquinone and 0.8% ethyl 4- N , N -dimethylaminobenzoate (referred to as BisGMA–TEGDMA resin). The NACP mass fraction in the resin was 30%, and the glass mass fraction was 35%, following a previous study . The resin filled with 30% NACP without any glass fillers is referred to as “Resin with 30% NACP without glass”. The composite filled with 30% NACP + 35% nano-silica is referred to as “NACP + nano silica composite”. The composite filled with 30% NACP + 35% barium boroaluminosilicate glass is referred to as “NACP composite”.
2.2
Fabrication of QAS and silver nanocomposites
The synthesis of bis(2-methacryloyloxyethyl) dimethylammonium bromide, termed ionic dimethacrylate-1 (IDMA-1), was described recently . IDMA-1 was selected as the quaternary ammonium dimethacrylate (QADM) to incorporate into the nanocomposites in the present study. Its synthesis was carried out using a modified Menschutkin reaction, where a tertiary amine group was reacted with an organo-halide. A benefit of this reaction is that the reaction products are generated at virtually quantitative amounts and require minimal purification . Briefly, 10 mmol of 2-( N , N -dimethylamino)ethyl methacrylate (DMAEMA, Sigma–Aldrich, St. Louis, MO) and 10 mmol of 2-bromoethyl methacrylate (BEMA, Monomer-Polymer and Dajec Labs, Trevose, PA) were combined with 3 g of ethanol in a 20 mL scintillation vial. A magnetic stir bar was added, and the vial was stirred at 60 °C for 24 h. The solvent was removed via evaporation, forming a clear, colorless, and viscous liquid. The QADM thus obtained was then mixed with the photo-activated BisGMA–TEGDMA resin at a QADM mass fraction of 20%. This resin is referred to as the BisGMA–TEGDMA–QADM resin. A previous study showed that 20% QADM greatly reduced bacterial growth on the polymer surfaces . The BisGMA–TEGDMA–QADM resin was then filled with 30% NACP and 35% barium boroaluminosilicate glass, and this composite is referred to as “NACP + QADM”. Hence, the QADM mass fraction in the final composite was 20% × 35% = 7%.
Silver 2-ethylhexanoate powder (Strem Chemicals, New Buryport, MA) at 0.08 g was dissolved into 1 g of 2-(tert-butylamino)ethyl methacrylate (TBAEMA, Sigma) by stirring, and then 1% of this solution was added to the resin. The mass fraction of Ag salt in the resin was 0.08%, according to a recent study . TBAEMA improves the solubility by forming Ag–N coordination bonds with Ag ions, thereby facilitating the Ag salt to dissolve in the resin solution. TBAEMA was specifically selected since it contains reactive methacrylate groups and therefore can be chemically incorporated into the polymer network upon photopolymerization .
To fabricate the “NACP + NAg” composite, the Ag-TBAEMA was mixed with BisGMA–TEGDMA, and then 30% NACP and 35% barium boroaluminosilicate glass were added to the resin. Since the resin mass fraction was 35% in the composite, the 0.08% of Ag in the resin yielded 0.028% of Ag mass fraction in the composite. To fabricate the “NACP + QADM + NAg” composite, the Ag-TBAEMA was mixed with the BisGMA–TEGDMA–QADM resin. NACP and glass filler levels were selected to yield a cohesive paste that was readily mixed and not dry . Each paste was placed into rectangular molds of (2 × 2 × 25) mm for mechanical testing, and disk molds of 9 mm in diameter and 2 mm in thickness for biofilm experiments. The specimens were photo-polymerized (Triad 2000, Dentsply, York, PA) for 1 min on each side.
In addition, a commercial composite with glass nanoparticles of 40–200 nm and a low level of F release was tested (Heliomolar, Ivoclar, Ontario, Canada) and is referred to as “CompositeF”. The fillers were silica and ytterbium-trifluoride with a filler level of 66.7%. Heliomolar is indicated for Class I and Class II restorations in the posterior region, Classes III–V restorations, and pit and fissure sealing. Another commercial composite, Renamel (Cosmedent, Chicago, IL), served as a non-releasing control (referred to as “CompositeNoF”). It consisted of nanofillers of 20–40 nm with 60% (by mass) fillers in a multifunctional methacrylate ester resin . Renamel is indicated for Classes III, IV, and V restorations. The control specimens were also photo-cured in the same manner as described above.
2.3
Transmission electron microscopy (TEM)
TEM was performed to examine the silver nanoparticles in the resin. The mass fraction of the Ag salt was 0.08% in the resin, the same as that described above. Following a previous study , a thin sheet of mica was partially split and the Ag-containing resin was placed in the gap. The resin-mica sandwich was pressed with an applied load of 2.7 × 10 7 N to form a thin sheet of resin in between the two mica layers . The resin in the mica was photo-cured for 1 min on each side in the same manner as described above. The mica sheet was then split apart after 1 d using a scalpel to expose the polymerized film. An ultrathin layer of carbon was vacuum-evaporated onto the composite (Electron Microscopy Sciences, Hatfield, PA). The carbon-coated sample was then partially submerged in distilled water in order to float the thin film onto the water’s surface. A copper grid was then used to retrieve the film. After drying, TEM was performed on a Tecnai T12 high resolution transmission electron microscope (FEI Company, Hillsboro, OR) using an accelerating voltage of 120 kV. The TEM images were collected and the sizes of the silver particles were measured using AMT V600 image analysis software (Advanced Microscopy Techniques, Woburn, MA).
2.4
Flexural testing
The composite bars were immersed in distilled water at 37 °C for 1 d. Hydrated specimens were then fractured in three-point flexure with a 10-mm span at a crosshead-speed of 1 mm/min on a computer-controlled Universal Testing Machine (5500R, MTS, Cary, NC). Flexural strength ( S ) was calculated as: S = 3 P max L /(2 bh 2 ), where P max is the fracture load, L is span, b is specimen width and h is specimen thickness. Elastic modulus ( E ) was calculated as: E = ( P / d )( L 3 /[4 bh 3 ]), where load P divided by displacement d is the slope of the load–displacement curve in the linear elastic region. The specimens were fractured while still wet, within several minutes from being taken out of water. Six specimens were tested for each material ( n = 6).
2.5
S. mutans inoculation and live/dead assay
S. mutans was selected because it is a cariogenic bacterium and is the primary causative agent of dental caries . S. mutans bacteria were obtained commercially (ATCC 700610, UA159, American Type Culture, Manassas, VA). Their use was approved by University of Maryland. The growth medium consisted of brain heart infusion (BHI) broth (BD, Franklin Lakes, NJ) supplemented with 0.2% sucrose. Fifteen microliters of stock bacteria was added to 15 mL of growth medium and incubated at 37 °C with 5% CO 2 for 16 h, during which the S. mutans were suspended in the growth medium. The inoculation medium was formed by diluting this S. mutans culture 10-fold in growth medium .
Six composites were tested in biofilm experiments: The four nanocomposites listed in Table 1 , and the two control composites. Composite disks were sterilized in an ethylene oxide sterilizer (Anprolene AN 74i, Andersen, Haw River, NC). For each composite, six disks ( n = 6) were used for each biofilm experiment at each time point, except for live/dead staining in which three disks were used for each composite at each time point. Each disk was placed in a well of a 24-well plate and inoculated with 1.5 mL of inoculation medium. The samples were incubated at 5% CO 2 and 37 °C for 1 d to form the initial biofilms, or 3 d to form mature biofilms. The growth medium was changed every 24 h, by transferring the disks to a new 24-well plate with fresh medium. After 1 or 3 d, the biofilms on the disks were washed three times with phosphate buffered saline (PBS) to remove loose bacteria, and then stained using the BacLight live/dead kit (Molecular Probes, Eugene, OR). Live bacteria were stained with Syto 9 to produce green fluorescence, and bacteria with compromised membranes were stained with propidium iodide to produce red fluorescence. The stained disks were examined using an inverted epifluorescence microscope (Eclipse TE2000-S, Nikon, Melville, NY). At each time period, four images were collected on each of three disks per material, yielding 12 images for each condition.
NACP (%) | Glass (%) | BisGMA–TEGDMA (%) | QADM (%) | NAg (%) | |
---|---|---|---|---|---|
NACP composite | 30 | 35 | 35 | 0 | 0 |
NACP + QADM composite | 30 | 35 | 28 | 7 | 0 |
NACP + NAg composite | 30 | 35 | 34.972 | 0 | 0.028 |
NACP + QADM + NAg composite | 30 | 35 | 27.972 | 7 | 0.028 |
a NACP refers to nanoparticles of amorphous calcium phosphate. Glass refers to barium boroaluminosilicate glass particles. BisGMA–TEGDMA refers to a BisGMA and TEGDMA mixture at 1:1 ratio plus 0.2% camphorquinone and 0.8% ethyl 4- N , N -dimethylaminobenzoate. NAg refers to nanoparticles of silver.
2.6
MTT metabolic assay
The composite disks were placed in a 24-well plate, inoculated with 1.5 mL of the inoculation medium, and cultured for 1 d or 3 d. Each disk was transferred to a new 24-well plate for the MTT (3-(4,5-dimethylthiazol-2-yl)-2,5-diphenyltetrazolium bromide) assay. It is a colorimetric assay that measures the enzymatic reduction of MTT, a yellow tetrazole, to formazan. The MTT assay for S. mutans biofilms was previously described . Briefly, 1 mL of MTT dye (0.5 mg/mL MTT in PBS) was added to each well and incubated at 37 °C in 5% CO 2 for 1 h. During this process, metabolically active bacteria reduced the MTT to purple formazan. After 1 h, the disks were transferred to a new 24-well plate, 1 mL of dimethyl sulfoxide (DMSO) was added to solubilize the formazan crystals, and the plate was incubated for 20 min with gentle mixing at room temperature in the dark. After brief mixing via pipetting, 200 μL of the DMSO solution from each well was transferred to a 96-well plate, and the absorbance at 540 nm (optical density OD 540 ) was measured via a microplate reader (SpectraMax M5, Molecular Devices, Sunnvale, CA). A higher absorbance indicates a higher formazan concentration, which in turn indicates more metabolic activity in the biofilm on the composite.
2.7
Lactic acid production and viable cell counts
Composite disks with 3 d biofilms were rinsed in cysteine peptone water (CPW) to remove loose bacteria. Each disk was placed in a new 24-well plate and 1.5 mL of buffered peptone water (BPW) supplemented with 0.2% sucrose was added. BPW medium was used so that the mature biofilm would remain stable during the 3 h acid production assay. The relatively high buffer capacity of BPW should prevent the pH from becoming significantly acidic, as a low pH would hinder bacterial acid production. Disks with biofilms were incubated at 5% CO 2 and 37 °C for 3 h to allow the biofilms to produce acid. After 3 h, the BPW solutions were stored for lactate analysis. Lactate concentrations in the BPW solutions were determined using an enzymatic (lactate dehydrogenase) method . The microplate reader was used to measure the absorbance at 340 nm (OD 340 ) for the collected BPW solutions. Standard curves were prepared using a lactic acid standard (Supelco Analytical, Bellefonte, PA).
After the disks with biofilms were treated for lactic acid production, colony-forming unit (CFU) counts were used to quantify the total number of viable bacteria present on each composite disk. When biofilms are properly dispersed and diluted, each viable bacterium results in a single, countable colony on an agar plate. The disks were transferred into tubes with 2 mL CPW. The biofilms were harvested by sonicating (3510R-MTH, Branson, Danbury, CT) for 3 min, and then vortexing at maximum speed for 20 s using a vortex mixer (Fisher, Pittsburgh, PA). This protocol removed and dispersed the biofilms from the disks. The bacterial suspensions were serially diluted, spread onto BHI agar plates, and incubated for 3 d at 5% CO 2 and 37 °C. At 1 d and 3 d, the number of colonies that grew were counted and used, along with the dilution factor, to calculate total CFUs on each disk.
2.8
Statistical analysis
One-way and two-way analyses of variance (ANOVA) were performed to detect the significant effects of the variables. Tukey’s multiple comparison test was used to compare the data at a p -value of 0.05. Each standard deviation (sd) serves as the estimate for the standard uncertainty associated with a particular measurement.
2
Materials and methods
2.1
Fabrication of calcium phosphate nanocomposite
A spray-drying technique as described previously was used to make nanoparticles of ACP (Ca 3 [PO 4 ] 2 ). To make nanoparticles of ACP (referred to as NACP), calcium carbonate (CaCO 3 , Fisher, Fair Lawn, NJ) and dicalcium phosphate anhydrous (CaHPO 4 , Baker Chemical Co., Phillipsburg, NJ) were dissolved into an acetic acid solution to obtain final Ca and PO 4 ionic concentrations of 8 mmol/L and 5.333 mmol/L, respectively. This resulted in a Ca/P molar ratio of 1.5, the same as that for ACP. This solution was sprayed into a heated chamber, and an electrostatic precipitator (AirQuality, Minneapolis, MN) was used to collect the dried particles. This produced NACP with a mean particle size of 116 nm, as measured in a previous study .
Two types of co-fillers were used for reinforcement: barium boroaluminosilicate glass particles of a mean diameter of 1.4 μm (Caulk/Dentsply, Milford, DE), and nano-sized silica glass (Aerosil-OX50, Degussa, Ridgefield, NJ) with a mean diameter of 40 nm. Each glass was silanized with 4% 3-methacryloxypropyltrimethoxysilane and 2% n-propylamine (all by mass, unless otherwise noted). A resin of BisGMA (bisphenol glycerolate dimethacrylate) and TEGDMA (triethylene glycol dimethacrylate) at 1:1 mass ratio was rendered light-curable with 0.2% camphorquinone and 0.8% ethyl 4- N , N -dimethylaminobenzoate (referred to as BisGMA–TEGDMA resin). The NACP mass fraction in the resin was 30%, and the glass mass fraction was 35%, following a previous study . The resin filled with 30% NACP without any glass fillers is referred to as “Resin with 30% NACP without glass”. The composite filled with 30% NACP + 35% nano-silica is referred to as “NACP + nano silica composite”. The composite filled with 30% NACP + 35% barium boroaluminosilicate glass is referred to as “NACP composite”.
2.2
Fabrication of QAS and silver nanocomposites
The synthesis of bis(2-methacryloyloxyethyl) dimethylammonium bromide, termed ionic dimethacrylate-1 (IDMA-1), was described recently . IDMA-1 was selected as the quaternary ammonium dimethacrylate (QADM) to incorporate into the nanocomposites in the present study. Its synthesis was carried out using a modified Menschutkin reaction, where a tertiary amine group was reacted with an organo-halide. A benefit of this reaction is that the reaction products are generated at virtually quantitative amounts and require minimal purification . Briefly, 10 mmol of 2-( N , N -dimethylamino)ethyl methacrylate (DMAEMA, Sigma–Aldrich, St. Louis, MO) and 10 mmol of 2-bromoethyl methacrylate (BEMA, Monomer-Polymer and Dajec Labs, Trevose, PA) were combined with 3 g of ethanol in a 20 mL scintillation vial. A magnetic stir bar was added, and the vial was stirred at 60 °C for 24 h. The solvent was removed via evaporation, forming a clear, colorless, and viscous liquid. The QADM thus obtained was then mixed with the photo-activated BisGMA–TEGDMA resin at a QADM mass fraction of 20%. This resin is referred to as the BisGMA–TEGDMA–QADM resin. A previous study showed that 20% QADM greatly reduced bacterial growth on the polymer surfaces . The BisGMA–TEGDMA–QADM resin was then filled with 30% NACP and 35% barium boroaluminosilicate glass, and this composite is referred to as “NACP + QADM”. Hence, the QADM mass fraction in the final composite was 20% × 35% = 7%.
Silver 2-ethylhexanoate powder (Strem Chemicals, New Buryport, MA) at 0.08 g was dissolved into 1 g of 2-(tert-butylamino)ethyl methacrylate (TBAEMA, Sigma) by stirring, and then 1% of this solution was added to the resin. The mass fraction of Ag salt in the resin was 0.08%, according to a recent study . TBAEMA improves the solubility by forming Ag–N coordination bonds with Ag ions, thereby facilitating the Ag salt to dissolve in the resin solution. TBAEMA was specifically selected since it contains reactive methacrylate groups and therefore can be chemically incorporated into the polymer network upon photopolymerization .
To fabricate the “NACP + NAg” composite, the Ag-TBAEMA was mixed with BisGMA–TEGDMA, and then 30% NACP and 35% barium boroaluminosilicate glass were added to the resin. Since the resin mass fraction was 35% in the composite, the 0.08% of Ag in the resin yielded 0.028% of Ag mass fraction in the composite. To fabricate the “NACP + QADM + NAg” composite, the Ag-TBAEMA was mixed with the BisGMA–TEGDMA–QADM resin. NACP and glass filler levels were selected to yield a cohesive paste that was readily mixed and not dry . Each paste was placed into rectangular molds of (2 × 2 × 25) mm for mechanical testing, and disk molds of 9 mm in diameter and 2 mm in thickness for biofilm experiments. The specimens were photo-polymerized (Triad 2000, Dentsply, York, PA) for 1 min on each side.
In addition, a commercial composite with glass nanoparticles of 40–200 nm and a low level of F release was tested (Heliomolar, Ivoclar, Ontario, Canada) and is referred to as “CompositeF”. The fillers were silica and ytterbium-trifluoride with a filler level of 66.7%. Heliomolar is indicated for Class I and Class II restorations in the posterior region, Classes III–V restorations, and pit and fissure sealing. Another commercial composite, Renamel (Cosmedent, Chicago, IL), served as a non-releasing control (referred to as “CompositeNoF”). It consisted of nanofillers of 20–40 nm with 60% (by mass) fillers in a multifunctional methacrylate ester resin . Renamel is indicated for Classes III, IV, and V restorations. The control specimens were also photo-cured in the same manner as described above.
2.3
Transmission electron microscopy (TEM)
TEM was performed to examine the silver nanoparticles in the resin. The mass fraction of the Ag salt was 0.08% in the resin, the same as that described above. Following a previous study , a thin sheet of mica was partially split and the Ag-containing resin was placed in the gap. The resin-mica sandwich was pressed with an applied load of 2.7 × 10 7 N to form a thin sheet of resin in between the two mica layers . The resin in the mica was photo-cured for 1 min on each side in the same manner as described above. The mica sheet was then split apart after 1 d using a scalpel to expose the polymerized film. An ultrathin layer of carbon was vacuum-evaporated onto the composite (Electron Microscopy Sciences, Hatfield, PA). The carbon-coated sample was then partially submerged in distilled water in order to float the thin film onto the water’s surface. A copper grid was then used to retrieve the film. After drying, TEM was performed on a Tecnai T12 high resolution transmission electron microscope (FEI Company, Hillsboro, OR) using an accelerating voltage of 120 kV. The TEM images were collected and the sizes of the silver particles were measured using AMT V600 image analysis software (Advanced Microscopy Techniques, Woburn, MA).
2.4
Flexural testing
The composite bars were immersed in distilled water at 37 °C for 1 d. Hydrated specimens were then fractured in three-point flexure with a 10-mm span at a crosshead-speed of 1 mm/min on a computer-controlled Universal Testing Machine (5500R, MTS, Cary, NC). Flexural strength ( S ) was calculated as: S = 3 P max L /(2 bh 2 ), where P max is the fracture load, L is span, b is specimen width and h is specimen thickness. Elastic modulus ( E ) was calculated as: E = ( P / d )( L 3 /[4 bh 3 ]), where load P divided by displacement d is the slope of the load–displacement curve in the linear elastic region. The specimens were fractured while still wet, within several minutes from being taken out of water. Six specimens were tested for each material ( n = 6).
2.5
S. mutans inoculation and live/dead assay
S. mutans was selected because it is a cariogenic bacterium and is the primary causative agent of dental caries . S. mutans bacteria were obtained commercially (ATCC 700610, UA159, American Type Culture, Manassas, VA). Their use was approved by University of Maryland. The growth medium consisted of brain heart infusion (BHI) broth (BD, Franklin Lakes, NJ) supplemented with 0.2% sucrose. Fifteen microliters of stock bacteria was added to 15 mL of growth medium and incubated at 37 °C with 5% CO 2 for 16 h, during which the S. mutans were suspended in the growth medium. The inoculation medium was formed by diluting this S. mutans culture 10-fold in growth medium .
Six composites were tested in biofilm experiments: The four nanocomposites listed in Table 1 , and the two control composites. Composite disks were sterilized in an ethylene oxide sterilizer (Anprolene AN 74i, Andersen, Haw River, NC). For each composite, six disks ( n = 6) were used for each biofilm experiment at each time point, except for live/dead staining in which three disks were used for each composite at each time point. Each disk was placed in a well of a 24-well plate and inoculated with 1.5 mL of inoculation medium. The samples were incubated at 5% CO 2 and 37 °C for 1 d to form the initial biofilms, or 3 d to form mature biofilms. The growth medium was changed every 24 h, by transferring the disks to a new 24-well plate with fresh medium. After 1 or 3 d, the biofilms on the disks were washed three times with phosphate buffered saline (PBS) to remove loose bacteria, and then stained using the BacLight live/dead kit (Molecular Probes, Eugene, OR). Live bacteria were stained with Syto 9 to produce green fluorescence, and bacteria with compromised membranes were stained with propidium iodide to produce red fluorescence. The stained disks were examined using an inverted epifluorescence microscope (Eclipse TE2000-S, Nikon, Melville, NY). At each time period, four images were collected on each of three disks per material, yielding 12 images for each condition.
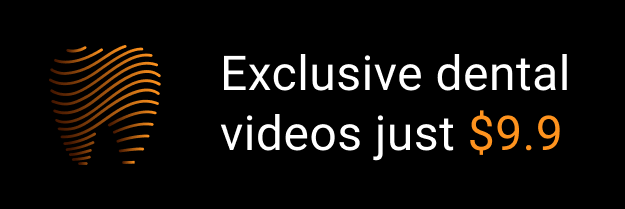