Abstract
Objective
Recurrent caries at the margins is a primary reason for restoration failure. The objectives of this study were to develop bonding agent with the double benefits of antibacterial and remineralizing capabilities, to investigate the effects of NACP filler level and solution pH on Ca and P ion release from adhesive, and to examine the antibacterial and dentin bond properties.
Methods
Nanoparticles of amorphous calcium phosphate (NACP) and a quaternary ammonium monomer (dimethylaminododecyl methacrylate, DMADDM) were synthesized. Scotchbond Multi-Purpose (SBMP) primer and adhesive served as control. DMADDM was incorporated into primer and adhesive at 5% by mass. NACP was incorporated into adhesive at filler mass fractions of 10%, 20%, 30% and 40%. A dental plaque microcosm biofilm model was used to test the antibacterial bonding agents. Calcium (Ca) and phosphate (P) ion releases from the cured adhesive samples were measured vs. filler level and solution pH of 7, 5.5 and 4.
Results
Adding 5% DMADDM and 10–40% NACP into bonding agent, and water-aging for 28 days, did not affect dentin bond strength, compared to SBMP control at 1 day ( p > 0.1). Adding DMADDM into bonding agent substantially decreased the biofilm metabolic activity and lactic acid production. Total microorganisms, total streptococci, and mutans streptococci were greatly reduced for bonding agents containing DMADDM. Increasing NACP filler level from 10% to 40% in adhesive increased the Ca and P ion release by an order of magnitude. Decreasing solution pH from 7 to 4 increased the ion release from adhesive by 6–10 folds.
Significance
Bonding agents containing antibacterial DMADDM and remineralizer NACP were formulated to have Ca and P ion release, which increased with NACP filler level from 10% to 40% in adhesive. NACP adhesive was “smart” and dramatically increased the ion release at cariogenic pH 4, when these ions would be most-needed to inhibit caries. Therefore, bonding agent containing DMADDM and NACP may be promising to inhibit biofilms and remineralize tooth lesions thereby increasing the restoration longevity.
1
Introduction
Resin composites are the principal material for tooth cavity restorations due to their esthetics and direct-filling capability . Advances in polymers and fillers have significantly enhanced the composite properties and clinical performance . However, a serious drawback is that composites tend to accumulate more biofilms/plaques in vivo than tooth enamel and other restorative materials . Biofilms with exposure to fermentable carbohydrates produce acids and are responsible for tooth caries . Furthermore, composite restorations are bonded to the tooth structure via adhesives . The bonded interface is considered the weak link between the restoration and the tooth structure . Hence extensive studies were performed to increase the bond strength and determine the mechanisms of tooth-restoration adhesion . One approach was to develop antibacterial adhesives to reduce biofilms and caries at the margins . The rationale was that, after tooth cavity preparation, there are residual bacteria in the dentinal tubules. In addition, marginal leakage during service could also allow new bacteria to invade into the tooth-restoration interfaces. Therefore, antibacterial bonding agents would be highly beneficial to combat bacteria and caries .
Accordingly, efforts were made to synthesize quaternary ammonium methacrylates (QAMs) and develop antibacterial resins . The 12-methacryloyloxydodecyl-pyridinium bromide (MDPB)-containing adhesive effectively inhibited oral bacteria growth . Antibacterial adhesive containing methacryloxyl ethyl cetyl dimethyl ammonium chloride (DMAE-CB) was also synthesized . Other studies developed a quaternary ammonium dimethacrylate (QADM) for incorporation into resins . Recently, a new quaternary ammonium monomer, dimethylaminododecyl methacrylate (DMADDM), was synthesized and imparted a potent anti-biofilm activity to bonding agent .
Another approach to combat caries was to incorporate calcium phosphate (CaP) particles to achieve remineralization capability . Composites were filled with amorphous calcium phosphate (ACP) in which the ACP particles had diameters of several μm to 55 μm . ACP composites released supersaturating levels of calcium (Ca) and phosphate (P) ions and remineralized enamel lesions in vitro . One drawback of traditional CaP composites is that they are mechanically weak and cannot be used as bulk restoratives . More recently, nanoparticles of ACP (NACP) were synthesized and incorporated into resins . NACP nanocomposite released high levels of Ca and P ions while possessing mechanical properties similar to commercial composite control . NACP nanocomposite neutralized acid challenges , remineralized enamel lesions in vitro , and inhibited caries in vivo . Due to the small particle size and high surface area, NACP in resin would have three main advantages: (1) improved mechanical properties , (2) high levels of ion release , and (3) being able to flow with bonding agent into small dentinal tubules . However, the Ca and P ion release from bonding agent with various amounts of NACP has not been measured in previous studies.
The objectives of this study were to develop bonding agent with double benefits of antibacterial and remineralizing capabilities, to investigate the effect of NACP filler level and solution pH on Ca and P ion release from the adhesive, and to examine the antibacterial and dentin bonding properties. It was hypothesized that: (1) Incorporation of DMADDM and NACP and water-aging for 1 month will not negatively affect the dentin bond strength; (2) DMADDM will impart a strong antibacterial activity to bonding agent, while NACP will have no effect on antibacterial activity; (3) Ca and P ion release will be directly proportional to NACP filler level in adhesive: (4) NACP adhesive will be “smart” to increase the ion release at acidic cariogenic pH, when these ions would be most-needed to inhibit caries.
2
Materials and methods
2.1
Synthesis of NACP and DMADDM
Nanoparticles of ACP (Ca 3 [PO 4 ] 2 ), referred to as NACP, were synthesized using a spray-drying technique as described previously . Briefly, calcium carbonate (CaCO 3 , Fisher, Fair Lawn, NJ) and dicalcium phosphate anhydrous (CaHPO 4 , Baker Chemical, Phillipsburg, NJ) were dissolved into an acetic acid solution to obtain final Ca and P ionic concentrations of 8 mmol/L and 5.333 mmol/L, respectively. The Ca/P molar ratio for the solution was 1.5, the same as that for ACP. The solution was sprayed into the heated chamber of the spray-drying apparatus. The dried particles were collected via an electrostatic precipitator (AirQuality, Minneapolis, MN), yielding NACP with a mean particle size of 116 nm .
The synthesis of DMADDM with an alkyl chain length of 12 was described elsewhere . Briefly, a modified Menschutkin reaction method was used in which a tertiary amine group was reacted with an organo-halide . 2-Bromoethyl methacrylate (BEMA) (Monomer-Polymer and Dajac Labs, Trevose, PA) was used as the organo-halide, and 1-(dimethylamino)docecane (DMAD) (Tokyo Chemical Industry, Tokyo, Japan) was the tertiary amine. Ten mmol of DMAD and 10 mmol of BEMA were added in a 20 mL scintillation vial with a magnetic stir bar . The vial was capped and stirred at 70 °C for 24 h. After the reaction was completed, the ethanol solvent was removed via evaporation, yielding DMADDM as a clear, colorless, and viscous liquid. The reaction and the products were verified via Fourier transform infrared (FTIR) spectroscopy in a previous study .
2.2
Incorporation of DMADDM and NACP into bonding agent
Scotchbond Multi-Purpose (SBMP, 3 M, St. Paul, MN) was used as the parent bonding system to test the effects of incorporation of DMADDM and NACP. According to the manufacturer, SBMP etchant contained 37% phosphoric acid. SBMP primer single-bottle contained 35–45% 2-hydroxyethylmethacrylate (HEMA), 10–20% copolymer of acrylic and itaconic acids, and 40–50% water. SBMP adhesive contained 60–70% BisGMA and 30–40% HEMA.
DMADDM was mixed with SBMP primer at DMADDM/(SBMP primer + DMADDM) = 5% by mass, following previous studies . Similarly, 5% of DMADDM was mixed with SBMP adhesive. NACP was mixed into the adhesive at mass fractions of: 10%, 20%, 30% and 40%. NACP filler levels higher than 40% were not used because the dentin bond strength decreased in preliminary study. The following six bonding systems were tested:
- (1)
Unmodified SBMP primer P and adhesive A (designated as “SBMP control”);
- (2)
P + 5% DMADDM, A + 5% DMADDM (designated as “P&A + 5DMADDM”);
- (3)
P + 5% DMADDM, A + 5% DMADDM + 10% NACP (P&A + 5DMADDM, A + 10NACP);
- (4)
P + 5% DMADDM, A + 5% DMADDM + 20% NACP (P&A + 5DMADDM, A + 20NACP);
- (5)
P + 5% DMADDM, A + 5% DMADDM + 30% NACP (P&A + 5DMADDM, A + 30NACP);
- (6)
P + 5% DMADDM, A + 5% DMADDM + 40% NACP (P&A + 5DMADDM, A + 40NACP).
2.3
Dentin shear bond strength testing
Caries-free human third molars were cleaned and stored in 0.01% thymol solution. Flat mid-coronal dentin surfaces were prepared by cutting off the tips of crowns with a diamond saw (Isomet, Buehler, Lake Bluff, IL) . Each tooth was embedded in a poly-carbonate holder (Bosworth, Skokie, IL) and ground perpendicular to the longitudinal axis on 320-grit silicon carbide paper until the occlusal enamel was removed. The dentin surface was etched with 37% phosphoric acid gel for 15 s and rinsed with water for 15 s . A primer was applied with a brush-tipped applicator and rubbed in for 15 s. The solvent was removed with a stream of air for 5 s, then an adhesive was applied and light-cured for 10 s (Optilux). A stainless-steel iris, having a central opening with a diameter of 4 mm and a thickness of 1.5 mm, was held against the adhesive-treated dentin surface. The opening was filled with a composite (TPH) and light-cured for 60 s. The bonded specimens were stored in water at 37 °C for 1 day or 28 days. A chisel with a Universal Testing Machine (MTS, Eden Prairie, MN) was aligned to be parallel to the composite-dentin interface . The load was applied at a rate of 0.5 mm/min until the bond failed. Dentin shear bond strength, S D , was calculated as: S D = 4 P /( πd 2 ), where P is the load at failure, and d is the diameter of the composite .
2.4
Saliva collection for dental plaque microcosm biofilm model
The dental plaque microcosm biofilm model was approved by the University of Maryland. This model has the advantage of maintaining much of the complexity and heterogeneity of in vivo plaques . To represent the diverse bacterial populations, saliva from ten healthy individuals was combined for the experiments. Saliva was collected from healthy adult donors having natural dentition without active caries or periopathology, and without the use of antibiotics within the past three months . The donors did not brush teeth for 24 h and stopped any food/drink intake for at least 2 h prior to donating saliva. Stimulated saliva was collected during parafilm chewing and kept on ice. An equal volume of saliva from each of the ten donors was combined, and diluted to 70% saliva and 30% glycerol. Aliquots of 1 mL were stored at −80 °C for subsequent use .
2.5
Resin specimens for biofilm experiments
Primer/adhesive/composite tri-layer disks were fabricated following previous studies . First, each primer was brushed on a glass slide, then a polyethylene mold (inner diameter = 9 mm, thickness = 2 mm) was placed on the glass slide. After drying with a stream of air, 10 μL of an adhesive was applied and cured for 20 s with Optilux . Then, the composite (TPH) was placed on the adhesive to fill the mold and cured for 1 min (Triad 2000, Dentsply, Milford, DE). The cured disks were agitated in water for 1 h to remove any uncured monomers, following a previous study . The disks were then immersed in distilled water at 37 °C for 1 day. The disks were then sterilized with an ethylene oxide sterilizer (Anprolene AN 74i, Andersen, Haw River, NC) and de-gassed for 7 days, following the manufacturer’s instructions. The specimens were then used for biofilm experiments.
2.6
Bacteria inoculum and live/dead biofilm staining
The saliva-glycerol stock was added, with 1:50 final dilution, to a growth medium as inoculum. The growth medium contained mucin (type II, porcine, gastric) at a concentration of 2.5 g/L; bacteriological peptone, 2.0 g/L; tryptone, 2.0 g/L; yeast extract, 1.0 g/L; NaCl, 0.35 g/L, KCl, 0.2 g/L; CaCl 2 , 0.2 g/L; cysteine hydrochloride, 0.1 g/L; haemin, 0.001 g/L; vitamin K 1 , 0.0002 g/L, at pH 7 . Each tri-layer disk was placed into a well of a 24-well plate with the primer side facing up. Each well was filled with 1.5 mL of inoculum, and incubated in 5% CO 2 at 37 °C. After 8 h, each specimen was transferred into a new 24-well plate with 1.5 mL fresh medium, and incubated in 5% CO 2 at 37 °C for 16 h. Then, each specimen was transferred into a new 24-well plate with 1.5 mL fresh medium, and incubated for 1 day. This totaled 2 days of incubation, which was shown to form biofilms on resins .
Disks with 2-day biofilms were rinsed with phosphate-buffered saline (PBS) and live/dead stained using the BacLight live/dead bacterial viability kit (Molecular Probes, Eugene, OR). Imaging was performed via confocal laser scanning microscopy (CLSM 510, Carl Zeiss, Thornwood, NY). Three randomly chosen fields of view were photographed for each of six disks, yielding a total of 18 images for each bonding agent.
2.7
MTT assay of biofilm metabolic activity
A MTT assay (3-[4,5-dimethylthiazol-2-yl]-2,5-diphenyltetrazolium bromide) was used to examine the metabolic activity of biofilms . MTT is a colorimetric assay that measures the enzymatic reduction of MTT, a yellow tetrazole, to formazan. Disks with 2-day biofilms ( n = 6) were transferred to a new 24-well plate, and 1 mL of MTT dye (0.5 mg/mL MTT in PBS) was added to each well and incubated at 37 °C in 5% CO 2 for 1 h. During the incubation, metabolically active bacteria metabolized the MTT, a yellow tetrazole, and reduced it to purple formazan inside the living cells. Disks were then transferred to new 24-well plates, and 1 mL of dimethyl sulfoxide (DMSO) was added to solubilize the formazan crystals. The plates were incubated for 20 min with gentle mixing at room temperature. Two hundred μL of the DMSO solution was collected, and its absorbance at 540 nm was measured via a microplate reader (SpectraMax M5, Molecular Devices, Sunnyvale, CA). A higher absorbance means a higher formazan concentration, which indicates more metabolic activity in the biofilms .
2.8
Lactic acid production by biofilms adherent on resin disks
Disks with 2-day biofilms ( n = 6) were rinsed with cysteine peptone water (CPW) to remove loose bacteria, and then transferred to 24-well plates containing 1.5 mL buffered-peptone water (BPW) plus 0.2% sucrose . The specimens were incubated for 3 h to allow the biofilms to produce acid. The BPW solutions were collected for lactate analysis using an enzymatic method. The 340-nm absorbance of BPW was measured with the microplate reader. Standard curves were prepared using a standard lactic acid (Supelco Analytical, Bellefonte, PA) .
2.9
Colony-forming unit (CFU) counts of biofilms adherent on resin disks
Disks with 2-day biofilms were transferred into tubes with 2 mL CPW, and the biofilms were harvested by sonication and vortexing via a vortex mixer (Fisher, Pittsburgh, PA) . Three types of agar plates were prepared. First, tryptic soy blood agar culture plates were used to determine total microorganisms . Second, mitis salivarius agar (MSA) culture plates, containing 15% sucrose, were used to determine total streptococci . This is because MSA contains selective agents crystal violet, potassium tellurite and trypan blue, which inhibit most gram-negative bacilli and most gram-positive bacteria except streptococci, thus enabling streptococci to grow . Third, cariogenic mutans streptococci are known to be resistant to bacitracin, and this property is often used to isolate mutans streptococci from the highly heterogeneous oral microflora. Hence, MSA agar culture plates plus 0.2 units of bacitracin per mL was used to determine mutans streptococci . The bacterial suspensions were serially diluted and spread onto agar plates for CFU analysis .
2.10
Calcium (Ca) and phosphate (P) ion release measurement
Preliminary studies indicated that when NACP was incorporated into SBMP adhesive, there was no loss in dentin bond strength. However, when NACP was incorporated into SBMP primer, the dentin bond strength decreased. Hence NACP was incorporated into SBMP adhesive but not into primer. Four groups of materials were tested for Ca and P ion release: (i) SBMP adhesive + 5% DMADDM + 10% NACP, (ii) SBMP adhesive + 5% DMADDM + 20% NACP, (iii) SBMP adhesive + 5% DMADDM + 30% NACP, (iv) SBMP adhesive + 5% DMADDM + 40% NACP. Each adhesive paste was placed into a rectangular mold of 2 mm × 2 mm × 12 mm following previous studies . The specimen was photo-cured (Triad 2000, Dentsply, York, PA) for 1 min on each open side of the mold and then stored at 37 °C for 1 day. A sodium chloride (NaCl) solution (133 mmol/L) was buffered to three different pH: pH 4 with 50 mmol/L lactic acid, pH 5.5 with 50 mmol/L acetic acid, and pH 7 with 50 mmol/L HEPES. Following previous studies , three specimens of 2 mm × 2 mm × 12 mm were immersed in 50 mL of solution at each pH, yielding a specimen volume/solution of 2.9 mm 3 /mL. This compared to a specimen volume per solution of approximately 3.0 mm 3 /mL in a previous study . For each solution, the concentrations of Ca and P ions released from the specimens were measured at 1, 3, 7, 14, 21, and 28 days. At each time, aliquots of 0.5 mL were removed and replaced by fresh solution. The aliquots were analyzed for Ca and P ions via a spectrophotometric method (DMS-80 UV-visible, Varian, Palo Alto, CA) using known standards and calibration curves .
2.11
Statistical analysis
One-way and two-way analyses-of-variance (ANOVA) were performed to detect the significant effects of the variables. Tukey’s multiple comparison was used to compare the data at p of 0.05.
2
Materials and methods
2.1
Synthesis of NACP and DMADDM
Nanoparticles of ACP (Ca 3 [PO 4 ] 2 ), referred to as NACP, were synthesized using a spray-drying technique as described previously . Briefly, calcium carbonate (CaCO 3 , Fisher, Fair Lawn, NJ) and dicalcium phosphate anhydrous (CaHPO 4 , Baker Chemical, Phillipsburg, NJ) were dissolved into an acetic acid solution to obtain final Ca and P ionic concentrations of 8 mmol/L and 5.333 mmol/L, respectively. The Ca/P molar ratio for the solution was 1.5, the same as that for ACP. The solution was sprayed into the heated chamber of the spray-drying apparatus. The dried particles were collected via an electrostatic precipitator (AirQuality, Minneapolis, MN), yielding NACP with a mean particle size of 116 nm .
The synthesis of DMADDM with an alkyl chain length of 12 was described elsewhere . Briefly, a modified Menschutkin reaction method was used in which a tertiary amine group was reacted with an organo-halide . 2-Bromoethyl methacrylate (BEMA) (Monomer-Polymer and Dajac Labs, Trevose, PA) was used as the organo-halide, and 1-(dimethylamino)docecane (DMAD) (Tokyo Chemical Industry, Tokyo, Japan) was the tertiary amine. Ten mmol of DMAD and 10 mmol of BEMA were added in a 20 mL scintillation vial with a magnetic stir bar . The vial was capped and stirred at 70 °C for 24 h. After the reaction was completed, the ethanol solvent was removed via evaporation, yielding DMADDM as a clear, colorless, and viscous liquid. The reaction and the products were verified via Fourier transform infrared (FTIR) spectroscopy in a previous study .
2.2
Incorporation of DMADDM and NACP into bonding agent
Scotchbond Multi-Purpose (SBMP, 3 M, St. Paul, MN) was used as the parent bonding system to test the effects of incorporation of DMADDM and NACP. According to the manufacturer, SBMP etchant contained 37% phosphoric acid. SBMP primer single-bottle contained 35–45% 2-hydroxyethylmethacrylate (HEMA), 10–20% copolymer of acrylic and itaconic acids, and 40–50% water. SBMP adhesive contained 60–70% BisGMA and 30–40% HEMA.
DMADDM was mixed with SBMP primer at DMADDM/(SBMP primer + DMADDM) = 5% by mass, following previous studies . Similarly, 5% of DMADDM was mixed with SBMP adhesive. NACP was mixed into the adhesive at mass fractions of: 10%, 20%, 30% and 40%. NACP filler levels higher than 40% were not used because the dentin bond strength decreased in preliminary study. The following six bonding systems were tested:
- (1)
Unmodified SBMP primer P and adhesive A (designated as “SBMP control”);
- (2)
P + 5% DMADDM, A + 5% DMADDM (designated as “P&A + 5DMADDM”);
- (3)
P + 5% DMADDM, A + 5% DMADDM + 10% NACP (P&A + 5DMADDM, A + 10NACP);
- (4)
P + 5% DMADDM, A + 5% DMADDM + 20% NACP (P&A + 5DMADDM, A + 20NACP);
- (5)
P + 5% DMADDM, A + 5% DMADDM + 30% NACP (P&A + 5DMADDM, A + 30NACP);
- (6)
P + 5% DMADDM, A + 5% DMADDM + 40% NACP (P&A + 5DMADDM, A + 40NACP).
2.3
Dentin shear bond strength testing
Caries-free human third molars were cleaned and stored in 0.01% thymol solution. Flat mid-coronal dentin surfaces were prepared by cutting off the tips of crowns with a diamond saw (Isomet, Buehler, Lake Bluff, IL) . Each tooth was embedded in a poly-carbonate holder (Bosworth, Skokie, IL) and ground perpendicular to the longitudinal axis on 320-grit silicon carbide paper until the occlusal enamel was removed. The dentin surface was etched with 37% phosphoric acid gel for 15 s and rinsed with water for 15 s . A primer was applied with a brush-tipped applicator and rubbed in for 15 s. The solvent was removed with a stream of air for 5 s, then an adhesive was applied and light-cured for 10 s (Optilux). A stainless-steel iris, having a central opening with a diameter of 4 mm and a thickness of 1.5 mm, was held against the adhesive-treated dentin surface. The opening was filled with a composite (TPH) and light-cured for 60 s. The bonded specimens were stored in water at 37 °C for 1 day or 28 days. A chisel with a Universal Testing Machine (MTS, Eden Prairie, MN) was aligned to be parallel to the composite-dentin interface . The load was applied at a rate of 0.5 mm/min until the bond failed. Dentin shear bond strength, S D , was calculated as: S D = 4 P /( πd 2 ), where P is the load at failure, and d is the diameter of the composite .
2.4
Saliva collection for dental plaque microcosm biofilm model
The dental plaque microcosm biofilm model was approved by the University of Maryland. This model has the advantage of maintaining much of the complexity and heterogeneity of in vivo plaques . To represent the diverse bacterial populations, saliva from ten healthy individuals was combined for the experiments. Saliva was collected from healthy adult donors having natural dentition without active caries or periopathology, and without the use of antibiotics within the past three months . The donors did not brush teeth for 24 h and stopped any food/drink intake for at least 2 h prior to donating saliva. Stimulated saliva was collected during parafilm chewing and kept on ice. An equal volume of saliva from each of the ten donors was combined, and diluted to 70% saliva and 30% glycerol. Aliquots of 1 mL were stored at −80 °C for subsequent use .
2.5
Resin specimens for biofilm experiments
Primer/adhesive/composite tri-layer disks were fabricated following previous studies . First, each primer was brushed on a glass slide, then a polyethylene mold (inner diameter = 9 mm, thickness = 2 mm) was placed on the glass slide. After drying with a stream of air, 10 μL of an adhesive was applied and cured for 20 s with Optilux . Then, the composite (TPH) was placed on the adhesive to fill the mold and cured for 1 min (Triad 2000, Dentsply, Milford, DE). The cured disks were agitated in water for 1 h to remove any uncured monomers, following a previous study . The disks were then immersed in distilled water at 37 °C for 1 day. The disks were then sterilized with an ethylene oxide sterilizer (Anprolene AN 74i, Andersen, Haw River, NC) and de-gassed for 7 days, following the manufacturer’s instructions. The specimens were then used for biofilm experiments.
2.6
Bacteria inoculum and live/dead biofilm staining
The saliva-glycerol stock was added, with 1:50 final dilution, to a growth medium as inoculum. The growth medium contained mucin (type II, porcine, gastric) at a concentration of 2.5 g/L; bacteriological peptone, 2.0 g/L; tryptone, 2.0 g/L; yeast extract, 1.0 g/L; NaCl, 0.35 g/L, KCl, 0.2 g/L; CaCl 2 , 0.2 g/L; cysteine hydrochloride, 0.1 g/L; haemin, 0.001 g/L; vitamin K 1 , 0.0002 g/L, at pH 7 . Each tri-layer disk was placed into a well of a 24-well plate with the primer side facing up. Each well was filled with 1.5 mL of inoculum, and incubated in 5% CO 2 at 37 °C. After 8 h, each specimen was transferred into a new 24-well plate with 1.5 mL fresh medium, and incubated in 5% CO 2 at 37 °C for 16 h. Then, each specimen was transferred into a new 24-well plate with 1.5 mL fresh medium, and incubated for 1 day. This totaled 2 days of incubation, which was shown to form biofilms on resins .
Disks with 2-day biofilms were rinsed with phosphate-buffered saline (PBS) and live/dead stained using the BacLight live/dead bacterial viability kit (Molecular Probes, Eugene, OR). Imaging was performed via confocal laser scanning microscopy (CLSM 510, Carl Zeiss, Thornwood, NY). Three randomly chosen fields of view were photographed for each of six disks, yielding a total of 18 images for each bonding agent.
2.7
MTT assay of biofilm metabolic activity
A MTT assay (3-[4,5-dimethylthiazol-2-yl]-2,5-diphenyltetrazolium bromide) was used to examine the metabolic activity of biofilms . MTT is a colorimetric assay that measures the enzymatic reduction of MTT, a yellow tetrazole, to formazan. Disks with 2-day biofilms ( n = 6) were transferred to a new 24-well plate, and 1 mL of MTT dye (0.5 mg/mL MTT in PBS) was added to each well and incubated at 37 °C in 5% CO 2 for 1 h. During the incubation, metabolically active bacteria metabolized the MTT, a yellow tetrazole, and reduced it to purple formazan inside the living cells. Disks were then transferred to new 24-well plates, and 1 mL of dimethyl sulfoxide (DMSO) was added to solubilize the formazan crystals. The plates were incubated for 20 min with gentle mixing at room temperature. Two hundred μL of the DMSO solution was collected, and its absorbance at 540 nm was measured via a microplate reader (SpectraMax M5, Molecular Devices, Sunnyvale, CA). A higher absorbance means a higher formazan concentration, which indicates more metabolic activity in the biofilms .
2.8
Lactic acid production by biofilms adherent on resin disks
Disks with 2-day biofilms ( n = 6) were rinsed with cysteine peptone water (CPW) to remove loose bacteria, and then transferred to 24-well plates containing 1.5 mL buffered-peptone water (BPW) plus 0.2% sucrose . The specimens were incubated for 3 h to allow the biofilms to produce acid. The BPW solutions were collected for lactate analysis using an enzymatic method. The 340-nm absorbance of BPW was measured with the microplate reader. Standard curves were prepared using a standard lactic acid (Supelco Analytical, Bellefonte, PA) .
2.9
Colony-forming unit (CFU) counts of biofilms adherent on resin disks
Disks with 2-day biofilms were transferred into tubes with 2 mL CPW, and the biofilms were harvested by sonication and vortexing via a vortex mixer (Fisher, Pittsburgh, PA) . Three types of agar plates were prepared. First, tryptic soy blood agar culture plates were used to determine total microorganisms . Second, mitis salivarius agar (MSA) culture plates, containing 15% sucrose, were used to determine total streptococci . This is because MSA contains selective agents crystal violet, potassium tellurite and trypan blue, which inhibit most gram-negative bacilli and most gram-positive bacteria except streptococci, thus enabling streptococci to grow . Third, cariogenic mutans streptococci are known to be resistant to bacitracin, and this property is often used to isolate mutans streptococci from the highly heterogeneous oral microflora. Hence, MSA agar culture plates plus 0.2 units of bacitracin per mL was used to determine mutans streptococci . The bacterial suspensions were serially diluted and spread onto agar plates for CFU analysis .
2.10
Calcium (Ca) and phosphate (P) ion release measurement
Preliminary studies indicated that when NACP was incorporated into SBMP adhesive, there was no loss in dentin bond strength. However, when NACP was incorporated into SBMP primer, the dentin bond strength decreased. Hence NACP was incorporated into SBMP adhesive but not into primer. Four groups of materials were tested for Ca and P ion release: (i) SBMP adhesive + 5% DMADDM + 10% NACP, (ii) SBMP adhesive + 5% DMADDM + 20% NACP, (iii) SBMP adhesive + 5% DMADDM + 30% NACP, (iv) SBMP adhesive + 5% DMADDM + 40% NACP. Each adhesive paste was placed into a rectangular mold of 2 mm × 2 mm × 12 mm following previous studies . The specimen was photo-cured (Triad 2000, Dentsply, York, PA) for 1 min on each open side of the mold and then stored at 37 °C for 1 day. A sodium chloride (NaCl) solution (133 mmol/L) was buffered to three different pH: pH 4 with 50 mmol/L lactic acid, pH 5.5 with 50 mmol/L acetic acid, and pH 7 with 50 mmol/L HEPES. Following previous studies , three specimens of 2 mm × 2 mm × 12 mm were immersed in 50 mL of solution at each pH, yielding a specimen volume/solution of 2.9 mm 3 /mL. This compared to a specimen volume per solution of approximately 3.0 mm 3 /mL in a previous study . For each solution, the concentrations of Ca and P ions released from the specimens were measured at 1, 3, 7, 14, 21, and 28 days. At each time, aliquots of 0.5 mL were removed and replaced by fresh solution. The aliquots were analyzed for Ca and P ions via a spectrophotometric method (DMS-80 UV-visible, Varian, Palo Alto, CA) using known standards and calibration curves .
2.11
Statistical analysis
One-way and two-way analyses-of-variance (ANOVA) were performed to detect the significant effects of the variables. Tukey’s multiple comparison was used to compare the data at p of 0.05.
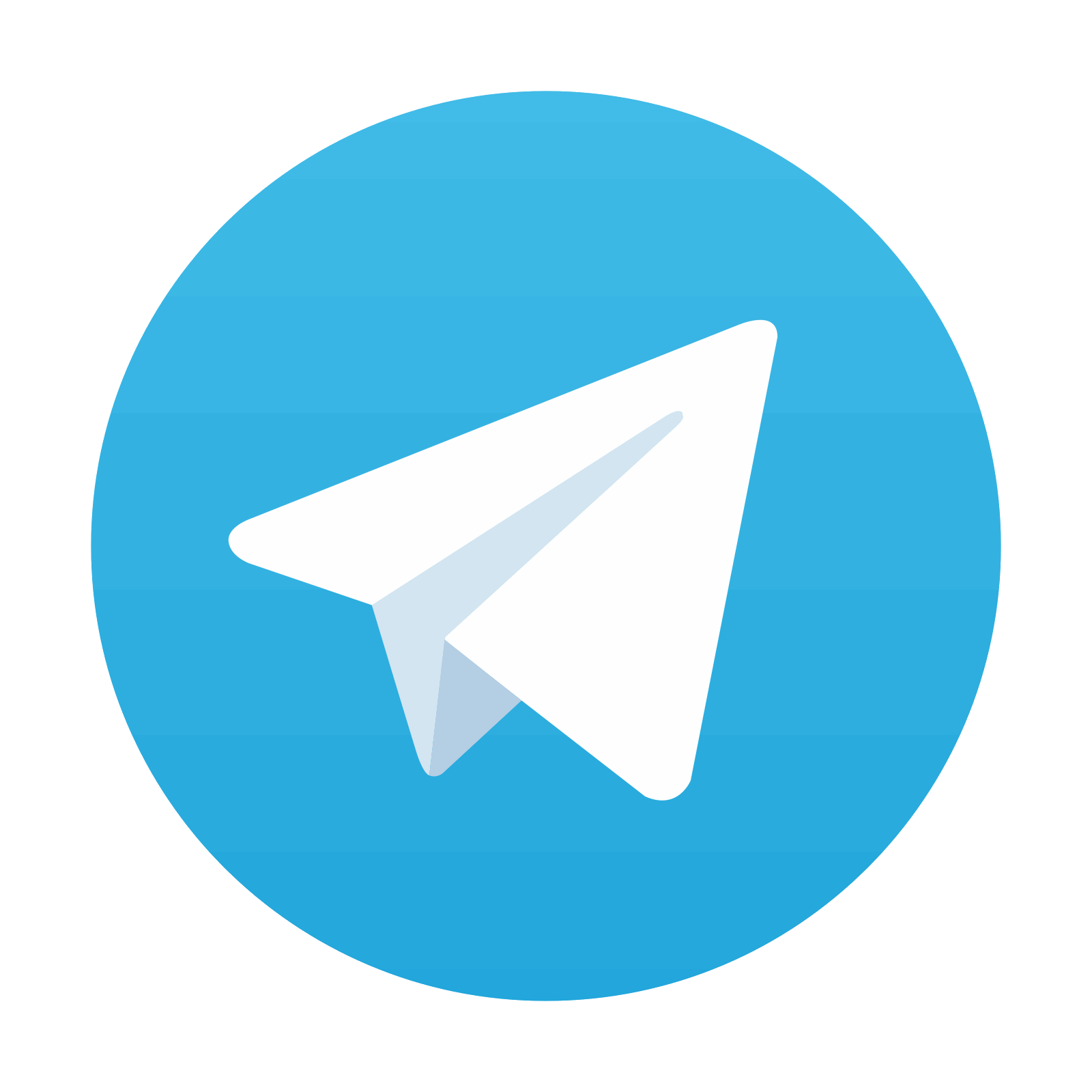
Stay updated, free dental videos. Join our Telegram channel

VIDEdental - Online dental courses
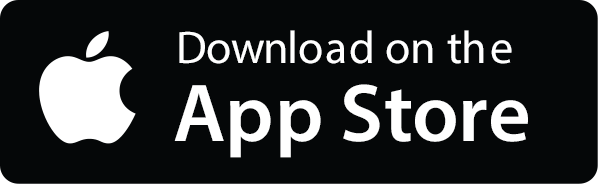
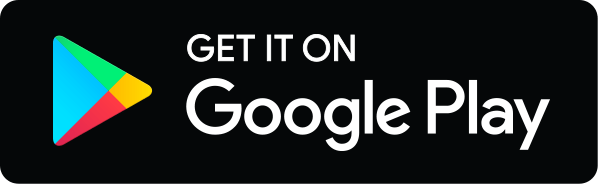