Graphical abstract

Highlights
- •
AgNPs cross-linked within glass ionomer cement (GIC) matrices generated antibiofilm effects at the nanocomposite surface.
- •
AgNP-containing GICs had higher compressive strengths and equivalent flexural strengths to unmodified GICs.
Abstract
Objective
To develop a silver nanoparticle (AgNP) formulation for incorporation into glass ionomer cements (GICs) which minimises biofilm growth on restoration surfaces.
Methods
GICs, Fuji IX, Ketac Molar, and Riva Selfcure were modified with 6, 10 and 24 μg per GIC capsule of α-lipoic acid-capped AgNPs. Monoculture biofilms of Streptococcus mutans were cultured (72 h) on GIC specimens (n = 3) and biofilm accumulation was quantified using a viability stain with confocal laser scanning microscopy. Compression strength and flexural strength (CS & FS) were measured according to ISO 9917-1:2007 (n = 8, n = 25). GIC colour was measured at 0, 1, and 14 days following AgNP incorporation using a digital spectrophotometer. Silver release from AgNP-modified GIC specimens was monitored at 1, 3, 7 and 14 days using inductively coupled plasma-mass spectrometry.
Results
AgNP-modified Fuji IX demonstrated the greatest reduction in biofilm accumulation, with 10 μg Ag/capsule inhibiting biofilm formation by 99%. Ketac Molar and Riva Selfcure required 24 μg Ag/capsule to achieve 78% biofilm reduction. AgNP-modified GICs demonstrated significantly higher CS and FS than sintered silver-containing GICs, and possessed equivalent or higher strength values when compared to unmodified GICs. The colour shades of AgNP-modified GICs were more comparable to VITA shades of non-modified GICs than were sintered silver-containing GICs. The silver (≥99.6%) remained within the GIC for at least two weeks following incorporation.
Significance
AgNP-modified GICs exhibited significant antibiofilm activity and retained mechanical properties equivalent or superior to non-modified GICs. AgNP-modified GICs could reduce bacterial colonisation on and around restorations thereby reducing restoration failure caused by secondary caries.
1
Introduction
Dental restorative materials accumulate greater quantities of plaque (bacterial biofilm) compared to innate dental enamel [ ]. Certain restorative materials promote biofilms with particular characteristics: thick but largely non-viable biofilms form on gold and amalgam restoratives whereas thin viable biofilms develop on ceramics, and biofilms generally result in significant surface deterioration and roughening of composites and GICs [ ]. This deterioration of the composite as a consequence of biofilm formation further enhances additional biofilm accumulation which ultimately causes either material failure or secondary caries at the restorative margin [ , ]. As such, the development of antibacterial materials to prevent secondary caries and increase the longevity of the restoration has been a longstanding goal [ ]. A variety of approaches to incorporate antibacterial agents into dental restoratives have been undertaken, many of which show promise, but also notable shortcomings. For instance, leachable compounds, including chlorhexidine, carolacton and benzalkonium chloride, reduce bacterial viability when incorporated into restorative materials but the effects are short-lived due to a burst release profile (initial rapid release followed by substantial decrease over a short time period) [ ].
GICs are a widely used restorative material with high ion leach rates that halt caries and aid remineralisation [ ]. While no restorative material developed to-date has ideal physical, mechanical, biological and surface properties for all clinical situations, GICs offer desirable properties including adhesion to tooth and metal surfaces, fluoride release, thermal compatibility with enamel, and biocompatibility. GICs offer a range of applications, including atraumatic restorative technique, luting, fissure sealants, orthodontic brackets, lamination techniques, non-stress-bearing restorations, and temporary restorations [ ]. GICs consist of ion-leachable glass (solid phase) typically containing SiO 2 , AlO 3 , CaF 2 , AlPO 4 , Na 3 AlF 6 , and an aqueous solution of an acrylic acid homo- or copolymer (polyelectrolyte) (polymeric or liquid phase) commonly containing tartaric acid to prolong material working time and increase the setting rate [ ]. GICs are the only dental material that undergoes chemical adhesion with the tooth structure due to the reaction between phosphate ions in the dental tissue and carboxylate groups in the GIC [ ]. While some reports suggest that GICs offer cariostatic activity due to F − release [ , ], other studies indicate that the extent of F − leaching is below that required for antimicrobial activity and that antimicrobial effects are not evident after the material is fully cured [ ]. Either way, GICs develop substantial surface biofilms [ ], and fail due to recurrent caries along the tooth-restorative interface [ ]. Thus, F − release alone cannot be relied upon for effective anti-biofilm activity in GICs. Instead, the addition of an antibacterial agent is desired, ideally a component that does not leach or stain/discolour, and that does not reduce mechanical strength. The present work demonstrates that these features are offered by incorporation of size-controlled α-lipoic acid-stabilised AgNPs.
Due to their antimicrobial activity [ ], incorporating AgNPs within GICs and other dental materials has been approached in a variety of ways [ , ]. Typically, the antimicrobial effect produced from AgNP-containing dental materials arises from leaching of either the silver ions or the AgNPs themselves, commonly detected in vitro by bacterial inhibition zones within the vicinity of the material. This is classified as a reservoir system and involves an initial burst effect followed by a longer period of relatively low silver release [ ]. However, the cytotoxic effect of silver has been directly correlated to the proportion of free silver ion release from the materials [ , , ]. We therefore present an alternative incorporation method using pre-synthesised surface-modified AgNPs with terminal carboxylate functional groups to stabilise and bind the AgNPs within the GIC matrix. By cross-linking the functionalised AgNPs within the GIC structure, silver leaching was negligible but a significant contact-based antibiofilm activity was demonstrated.
2
Materials and methods
2.1
AgNP synthesis and characterisation
Synthesis of α-lipoic acid-capped AgNPs was as previously described [ , ]. Briefly, two solutions were formed, each containing docusate sodium salt (0.33 M, AOT ≥ 96%, Cat.No.86140; Sigma Aldrich, Missouri, USA) in n -heptane (40 mL, Cat No.H350-1; Fischer Scientific, New Hampshire, USA). An aqueous solution of silver nitrate (0.13 M, 1.6 mL; Cat.No.10224350; Fisher Scientific) was added to one AOT/heptane solution, forming a microemulsion (EM), and an aqueous solution of sodium borohydride (1.84 M, 1.6 mL; NaBH 4 crystalline 98–99%, Cat.No. ICN10289425; Fischer Scientific) was added to the other AOT/heptane solution, forming a second EM. The two EMs were combined by dropwise addition and stirred in the dark for 1 h. Subsequently, the capping agent, α-lipoic acid (0.08 mM in 0.25 mL ethanol), was added to the combined EMs and stirred for 2 min. A phase separation was induced in the AgNP-containing microemulsion using an acetone: methanol mixture (1:1). The AgNPs were removed from the interface and washed using ethanol to remove any excess capping agent. The ethanol-AgNP mixture was centrifuged at 8000 × g for 5 min. The ethanol supernatant was removed from the AgNP solid and fresh ethanol (1 mL) was added. The AgNPs were temporarily resuspended by manual agitation and recentrifuged (8000 × g for 5 min). The ethanol resuspension and centrifugation were repeated three times. The ethanol was then removed and the AgNP solid dried at room temperature in the dark. The AgNPs were dispersed in deionised H 2 O (pH adjusted to 10.5 with ammonium hydroxide) and centrifuged (16,000 × g for 45 min). The supernatant containing the smallest AgNPs remaining in suspension was transferred to a fresh tube, centrifuged again and the supernatant recovered.
The α-lipoic acid-functionalised AgNPs were analysed in suspension at RT by dynamic light scattering (DLS) using a Zetasizer Nano (ZS model, Malvern Instruments; Malvern, UK), with detection angle of 173°, and a 3 mW He-Ne laser operating at a wavelength of 633 nm. Measurements were repeated four times, with a minimum equilibration time of 120 s. The mean hydrodynamic diameter (Z-average; Z-Avg) and polydispersity index (PDI) values were obtained from correlation functions using the “multiple narrow modes” algorithm based on a non-negative least square fit (Zetasizer software v. 6.20; Malvern Instruments; United Kingdom).
AgNPs were prepared for transmission electron microscopy (TEM) by depositing colloidal samples (10 μL) onto plasma glowed carbon-coated (400 – mesh) copper grids. After 60 s, the excess volume was removed with filter paper and the sample air dried. Images were obtained using a CM100 BioTWIN transmission electron microscope (Philips/FEI Corporation; Eindhoven, Holland) equipped with a LaB6 emitter and a MegaView III digital camera (Olympus Corporation; Tokyo, Japan). Nanoparticle size was determined by a particle analysis programme (Fiji is Just Image J, Soft Imaging System GmbH).
Inductively coupled plasma-mass spectrometry (ICP-MS) was used to determine the silver concentration of the α-lipoic acid-stabilised AgNP suspension and silver, sodium, calcium, and strontium concentrations from GIC leachates. Samples were prepared by addition of quartz distilled HNO 3 (0.1 mL) to 0.5 mL of sample in a Teflon digestion vessel (Cat. No. 010-500-264; SPS Science, Quebec, Canada). The samples were then digested at 100 °C for 1 h reducing the volumes to ≈ 0.2 mL. Sample volumes were increased to 35 mL with deionised H 2 O. Samples were then analysed with an ICP-MS instrument (Model 7900, Agilent, Technologies; California, USA) using a low matrix tune to determine the metal concentration.
2.2
AgNP-modified GIC preparation
The commercial GICs used were Fuji IX GP (Shade A3, Lot: 1711091; GC, Tokyo, Japan), Ketac™ Universal Aplicap™ Molar (Shade A3, Lot: 3257151; 3M-ESPE, St Paul, USA) and Riva Selfcure (Shade A3, Lot: C1711091F; SDI, Bayswater, VIC, AU). Sintered silver-containing GICs were GC Miracle Mix (Lot: 1806191), Ketac™ Silver Aplicap™ (Lot: 3297033), and Riva Silver (Lot: G1707061EG). α-Lipoic acid-coated AgNPs (6, 10 or 24 μg silver) were added to the liquid phase of each GIC capsule after removal of the capsule plunger or side hatch. The plunger/side hatch was then replaced, pushed flush into the capsule, and the contents of the capsule were mixed at high speed on a Caulk Vari-Mix III amalgamator (Dentsply; Pennsylvania, USA) for the time specified by the respective GIC manufacturer. GICs were then extruded into the moulds appropriate for each assay. Specimens were allowed to set for 1 h before analysis.
2.3
Antibiofilm effects of GICs
Streptococcus mutans (UA159) was obtained from the Department of Oral Sciences, University of Otago, New Zealand, and was regularly sub-cultured under anaerobic conditions (GasPak EZ anaerobe container system, BD Biosciences, New Jersey, USA) on Columbia blood agar (CBA; Cat No: 1100; Fort Richard, Mt. Wellington, New Zealand) at 37 °C. Brain heart infusion (BHI) (Cat No: 237500; Difco Laboratories, Detroit, MI, USA) was inoculated from a CBA culture and incubated in 5% CO 2 , at 37 °C for 16 h.
GIC specimens were prepared as 10 mm diameter × 2 mm discs (N = 3 × 3 GIC groups × 3 AgNP concentrations, plus controls). Specimens were sequentially finished on a TegraPol-21 polisher (Struers; Copenhagen, Denmark) with silicon carbide (1200, 2000, 4000 grit) for 30 s each side and sonicated in an ultrasonic cleaner for 5 min between polishing with each grit size. Samples were autoclaved at 121 °C for 15 min. Sterile GIC specimens were placed into the wells of a 24 well plate (CELLSTAR, Greiner Bio-One International; Kremsmünster, Austria) each containing BHI (1 mL) supplemented with 1% sucrose. Each well was inoculated with the bacterial culture (10 μL) deriving an initial absorbance (A600) of 0.01. The plate was placed in a rotary shaker incubator (37 °C, 60 rpm) for 24 h, after which the GIC specimens were aseptically removed and placed into wells containing BHI-sucrose (1 mL), and incubated at 37 °C for 24 h. The transfer of GIC specimens and re-incubation was repeated to produce a 72 h cultured biofilm. GIC specimens were dip-washed three times in phosphate-buffered saline (PBS) to remove non-adherent bacteria.
The biofilm/GIC specimens were treated with SYTO 9 and propidium iodide (Live/Dead™ Bac Light™ bacterial viability kit, Cat. No: L7012, Invitrogen, Thermo Fischer Scientific; Waltham, Massachusetts, USA) and remained at room temperature in the dark for 15 min, as per manufacturer’s instructions. The stained biofilms were gently washed with PBS, excess liquid wicked away, and samples were kept moist during imaging. Stained biofilms were examined with a LSM 710 confocal laser-scanning microscope (Carl Zeiss; Jena, Germany) and digital images captured with Zeiss Zen software, 2009 (3 images per specimen, 3 specimens per group). Three-dimensional stack images of the biofilms were analysed with Comstat2 (Molecular Microbial Ecology Group, Denmark) to quantify live, dead and total biovolumes. The biovolume (μm 3 /μm 2 ) is the number of biomass pixels of each stacked image multiplied by the voxel size, and divided by the substratum area of the image [ ]. Percentage biofilm reduction was calculated as a percentage of the non-modified GIC total biofilm biovolume.
2.4
Compressive strength
The compressive strength of GICs was measured according to the standard ISO 9917:2007 with size modification of the GIC specimens. Cylindrical GIC specimens (2 mm diameter × 3 mm) were prepared in Teflon moulds with a set time of 1 h, after which they were removed and immersed in deionised H 2 O at 37 °C for 24 h. Excess deionised H 2 O was wicked from the cylindrical specimens immediately before placement on the mechanical testing platform. A compressive load was aligned to the long axis of the specimen at a cross-head speed of 1 mm min −1 , using a Universal Testing Machine (Instron 3369; Instron, Norwood, MA, USA) with a 500 N load cell. Deionised H 2 O (pH 10.5, 5 μL)-modified GICs were used as the background control.
2.5
Flexural strength
Rectangular GIC bar specimens (20 mm × 2.0 mm × 2.0 mm) were prepared in Teflon moulds with a set time of 1 h, after which they were removed and immersed in deionised H 2 O at 37 °C for 24 h. Deionised H 2 O (pH 10.5, 5 μL)-modified GICs were used as the background control. Three-point bend tests were carried out on the GIC bar specimens using a universal testing machine with a 100 N load cell equipped with Bluehill 3 modular Software (Instron 3369; Instron, Norwood, MA,USA) and using a self-aligning three-point bending jig (flexural strength of ceramics test fixture ASTM C 1161, configuration A, fixture number WTF-CF-43; Wyoming Test Fixtures, USA) at a crosshead speed of 1 mm min −1 until the specimen fractured (ISO 9917:2007); the maximum force, flexural stress and strain were recorded. Prior to testing, each specimen was measured using a digital calliper and the dimensions entered into the Bluehill 3 software controlling the universal testing machine. Flexural strength (σ) was calculated using Eq. (1) , where F = the maximum load (N), L is the distance between the supports on which the sample is placed (20 mm), b is the width of the specimen (2 mm), and h is the height of the specimen (2 mm).
2.6
Colour measurement of GICs
Colour changes were characterised on GIC specimens prepared as discs (10 mm diameter × 2 mm) using the VITA Easyshade® V (VITA Zahnfabrik; Bad Säckingen, Germany), which utilises the Commission International d‘Eclairage L*a*b* colour space (CIE L*a*b*) compared to a white calibration control. All commercial GIC products were A3 classical tooth shade. GIC specimens were prepared in Teflon moulds and their colour immediately assessed (t = 0). Specimens were then placed in deionised H 2 O at 37 °C for a further 14 days and measurements were taken at day one and 14. Colour differences arising from the incorporation of AgNPs were expressed as ΔE* (Eq. (2) ) where <SPAN role=presentation tabIndex=0 id=MathJax-Element-2-Frame class=MathJax style="POSITION: relative" data-mathml='ΔL*’>Δ?*ΔL*
Δ L *
is the difference of whiteness or brightness, <SPAN role=presentation tabIndex=0 id=MathJax-Element-3-Frame class=MathJax style="POSITION: relative" data-mathml='Δa*’>Δ?*Δa*
Δ a *
was the difference in redness (positive a*) or greenness (negative a*), and <SPAN role=presentation tabIndex=0 id=MathJax-Element-4-Frame class=MathJax style="POSITION: relative" data-mathml='Δb*’>Δ?*Δb*
Δ b *
is the difference in yellowness (positive b*) or blueness (negative b*).
ΔE*=ΔL*2+Δa*2+Δb*212
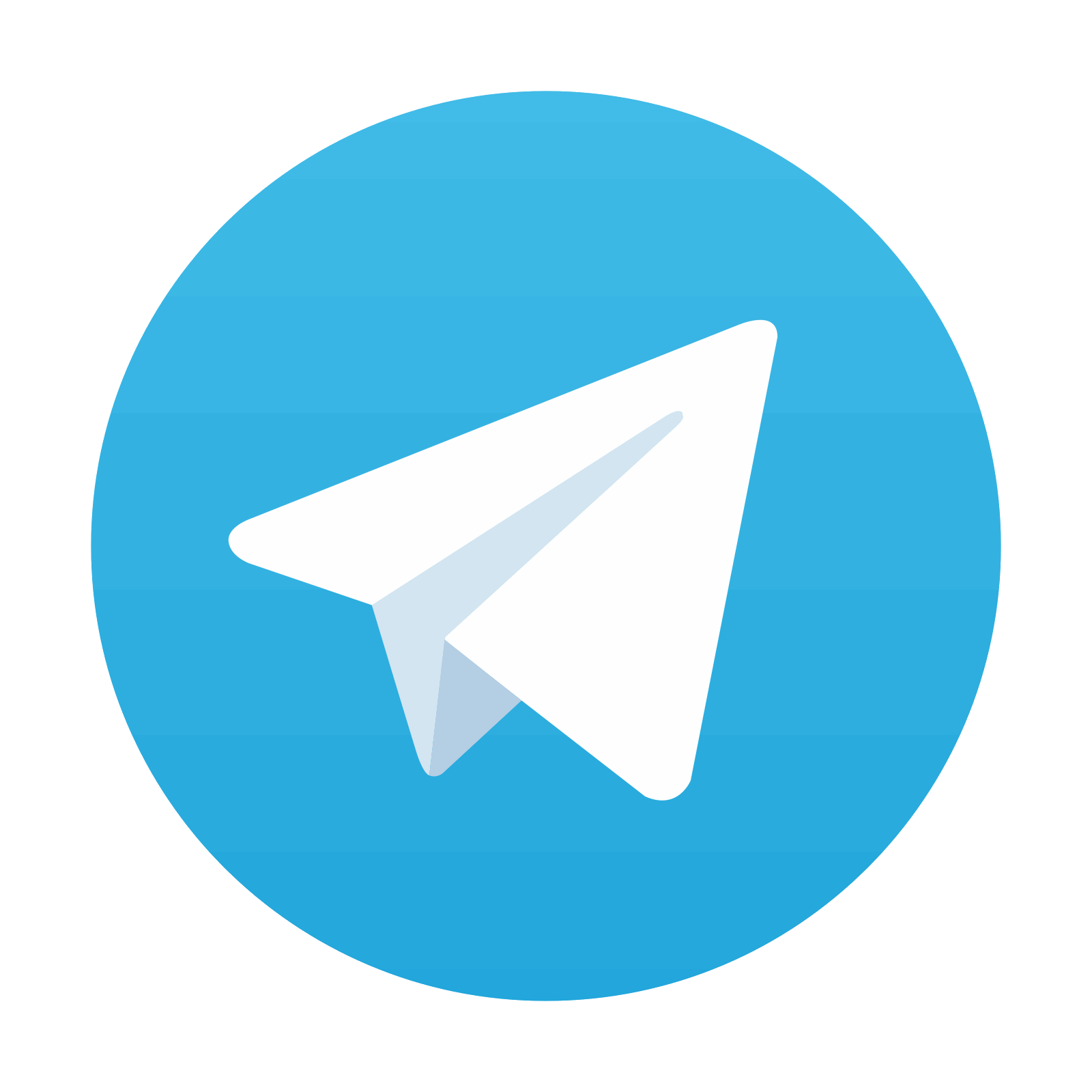
Stay updated, free dental videos. Join our Telegram channel

VIDEdental - Online dental courses
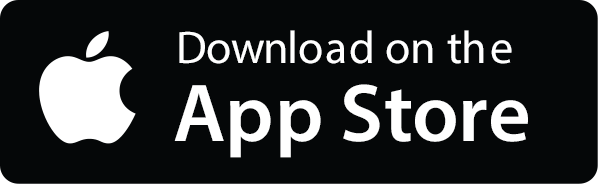
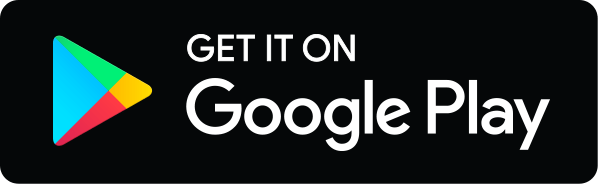
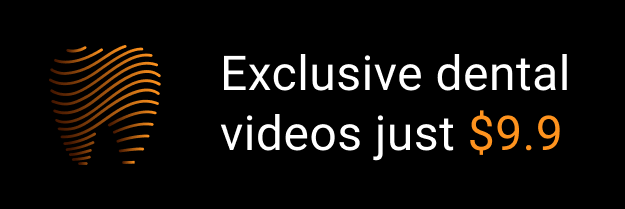