Abstract
Objectives
The aim of the study was to analyze the dissolving depth of adhesive resin monomers into pre-polymerized fiber-reinforced composites (FRC) with either a semi-interpenetrating polymer network (semi-IPN) or a cross-linked polymer (CLP) matrix.
Methods
Five unidirectional FRCs were tested, including one semi-IPN FRC (everStick ® C&B, StickTech, (ES)) and four CLP-FRCs (GrandTec ® , VOCO, (GT); Dentapreg ® , ADM, (DP); TenderFiber ® , Micerium, (TF); Splint-It ® , Pentron Clinical Technologies, (SI)). The FRCs were light-polymerized following manufacturers’ instructions: the oxygen inhibition layers were removed and the adhesive resin (Optibond-FL ® , KerrHawe) was labeled with a fluorescent dye (Rhodamine-B-isothiocyanate), which was then applied to the FRCs (5 min) and light-polymerized (40 s). Specimens were then prepared for confocal laser scanning microscopy. Three FRC strands per group were sectioned orthogonally to the direction of fibers, thus forming nine slices in each group. Four images were taken from each slice and the depths of adhesive penetration were measured in four sites per image ( n = 144 measurements per group).
Significance
Dissolving depths were 17.28 (3.04) μm (ES), 12.58 (2.94) μm (SI), 7.57 (1.91) μm (TF), 3.27 (0.73) μm (DP) and 2.55 (0.63) μm (GT). Samples were normally distributed. Differences between groups were analyzed by ANOVA (PostHoc Scheffé) showing four subgroups ( p = 0.05). The infiltration layers detected were either continuous/homogenous (ES, TF, DP GT) or discontinuous/insular (SI).
Conclusion
The adhesive resin monomers were able to diffuse significantly deeper into pre-polymerized semi-IPN specimens than into CLP materials. Semi-IPN specimens showed a homogenous and comparatively deeper layer of infiltration. The diffusion capabilities of secondary-IPN formation might increase the opportunity to establish a good bond between pre-polymerized FRC and new resin.
1
Introduction
In past decades, glass and ceramic materials have offered attractive stiffness qualities for load-bearing areas in aerospace and architecture, as well as in orthopedics and dentistry as they reveal high intrinsic bond strength . The incorporation of glass fibers into resin composites led to the development of today’s fiber-reinforced composites (FRC) and was evaluated as promising in diverse fields . Today, FRCs are being successfully applied in clinical dentistry. They are used to strengthen removable dentures , periodontal splints and root canal posts . Furthermore, FRC strands have been incorporated into adhesively fixed partial dentures (FPDs) in order to reinforce these prostheses . Recently, there have been reports on FRC implants . Merging fiber and composite technology allows dentists to partly overcome the limitations of conventional resin composites, namely considerable polymerization shrinkage, brittleness and low fracture toughness. Studies show that flexural and fatigue properties as well as the load-bearing capacities of FRCs could be improved to a certain extent .
For FRC technology, different types of glass fibers are in use. As continuous glass fibers, i.e., E-glass fibers (electrical glass), S-glass fibers (strength glass) and R-glass fibers (resistance glass) can be found in polymer-based FRCs in descending frequency . Fibers need to be pre-treated with a silane coating to ensure a reliable bond between fibers and resin matrix . One FRC (DP) displays a plasma-enhanced chemical vapour deposition (PECV) coating. Here, it is reported that reaction nests are formed by plasma ions hitting the glass. The manufacturer claims that as a result of this process, a direct bond between monomers and glass fibers becomes possible . However, such suggested advantageous physical properties of PECV-coating are not supported by controlled pre-clinical or clinical studies.
Also, the resin matrices of different pre-impregnated FRC materials differ in their composition. Either they consist of dimethacrylate monomers (e.g. Bis-GMA, UDMA) polymerizing to a cross-linked polymer (CLP matrix, Table 1 ) or they are composed of two polymers. PMMA polymerizes into a linear polymer which is interlaced with dimethacrylate monomers forming a separate cross-linked polymer known as a semi-Interpenetrating Polymer Network (semi-IPN, Table 1 ) . Consequently, the semi-IPN differs from a typical cross-linked polymer (CLP) in that there are two independent polymer networks which are not linked by chemical bonds .
Name | everStick ® C&B | GrandTec ® | Tender Fiber ® | Splint-it ® | Dentapreg ® PFU |
---|---|---|---|---|---|
Code | ES | GT | TF | SI | DP |
Manufacturer | StickTech Ltd. Turku Finland | Voco Cuxhaven Germany | Micerium Avegno Italy | Pentron Clinical Technologies Orange (CA) USA | ADM Brno Czech Republic |
Size of FRC | 1.5 mm × 12 mm | 2 mm × 5.5 mm | 1.7 mm × 12 mm | 3 mm | 3 mm × 0.3 mm × 50 mm |
Type of matrix | Semi-IPN | CLP | CLP | CLP | CLP |
Resin matrix | PMMA Bis-GMA | UDMA TEGDMA | Bis-GMA | Bis-GMA | Bis-GMA TEGDMA |
Type of Fibers | E-glass | R-glass | Glass fibers | Silicate glass fibers and polyethylene fibers | S2-glass |
Number of Fibers | 4000 | 4000 − 4500 | 6400 | Not specified | 8300 |
Direction of Fibers | Unidirectional | Unidrectional | Unidirectional | Unidirectional | Unidirectional |
Coating of Fibers | Epoxy silane | Silane | Silane | Not specified | Plasma-enhanced chemical vapour deposition (PECVD) |
What is the benefit of such composite polymers? Bonding between FRC and resin monomers or composite resin usually relies on the presence of a non-polymerised surface layer, the oxygen inhibition layer (OIL). However, in case the OIL is not present anymore (as in indirect or semi-direct restoration, a pre-fabricated FRC post or repair of FRC restoration in vivo), only residual functional groups in the pre-cured material are available for covalent bonding between “old” and “fresh” material. Here, semi-IPN structures offer the possibility to be dissolved by “fresh” monomers . During this so-called secondary-IPN, the polymer is first dissolved by solvent molecules of the adhesive resin swelling it to a gel state. Then, monomers diffuse into the solvent-rich regions . The dissolving gradient is influenced by exposure time, temperature, type of monomeric solvent and the polymeric structure of the substrate . Linear polymers can be fully dissolved whereas cross-linked polymers do not dissolve . The benefit of the semi-IPN structure for secondary-IPN bonding is therefore most valuable when pre-fabricated FRC restorations/posts must be attached to tooth substance or when an FRC restoration needs to be repaired in the oral cavity.
The aim of this investigation was to analyze the dissolving depth of monomers into pre-polymerized FRCs with either semi-IPN or CLP resin matrices. The working hypothesis was that interdiffusion would vary among materials and that monomers would be able to diffuse significantly deeper into the semi-IPN FRC than into CLP FRCs.
2
Materials and methods
2.1
Specimen preparation
Five materials were selected, one semi-IPN FRC (everStick ® C&B, StickTech, Turku, Finland) and four CLP-FRCs (GrandTec ® , VOCO, Cuxhaven, Germany; Dentapreg ® , ADM, Brno, Czech Republic; TenderFiber ® , Micerium, Avengno, Italy; Splint-It ® , Pentron Clinical Technologies, Orange, California, USA, Table 1 ). Ten millimeters of FRC material was cut off, then light-polymerized (Bluephase C8, Ivoclar Vivadent AG, Schaan, Liechtenstein, light intensity 680 mW/cm 2 , 40 s) following manufacturerś instructions. The oxygen inhibition layer (OIL) was removed using sandblasting (Al 2 O 3 sandblast powder from KaVo Rondoflex 2013, Kaltenbach & Voigt GmbH & Co., Biberach, Germany; particle size 27 μm) for 5 s. An ethanol solution (50%) was then rubbed over the surface with a microbrush to ensure complete removal of the OIL. To obtain a negative control zone half of the specimen surface was covered with nail polish (L’Oreal Jet Set ® ) ( Fig. 1 ). An adhesive resin (Optibond FL ® , KerrHawe, Bioggio, Switzerland) mixed with a fluorescent dye (Rhodamine-B-isothiocyanate, E. Merck, Darmstadt, Germany) was applied to the specimen surfaces using a microbrush. Specimens were then stored under a light-protection shield (Viva Pad ® , Ivoclar Vivadent AG, Schaan, Liechtenstein) for 5 min to allow monomers to dissolve into the FRCs . Longer impregnation periods (up to several hours) to enhance dissolving have been reported but such lengthy treatment times are impractical for clinical use and were therefore not considered. Then a second light polymerization (Bluephase C8, Ivoclar Vivadent AG, 40 s) was done. During preparation, specimens were handled with a pair of tweezers to avoid any surface contamination. This step-by-step procedure was followed in order to simulate the clinical processing of a pre-polymerized FRC, for example as a fiber framework for an indirect (laboratory made) or semi-direct adhesively bonded fixed partial denture (FPD) .

As a subgroup, all materials were processed once with intact OIL on their surfaces. The reason for this subgroup analysis was to visualize and evaluate the dissolved interface with intact OIL in terms of a positive control ( Fig. 2 ). The specimen preparation protocol was identical to the procedure described above except for the removal of the OIL with sandblasting and ethanol solution application.

FRC specimens were then embedded in auto-polymerizing acrylic resin (Paladur transparent ® , Kulzer, Wehrheim, Germany) and sectioned orthogonally to their long axis using a microtome saw (Leica 1600, Leica Microsystems, Wetzlar, Germany). Slice thickness was 200 nm. Sectioning of specimens at a defined angle of 90° was essential for the comparison of dissolving depths ( Fig. 3 ).

2.2
Evaluation of FRC – adhesive resin interfaces
Specimen slices were fixed on microscope slides (Histobond, Marienfeld, Lauda-Köningshofen, Germany) and evaluated using a confocal laser scanning microscope (CLSM, Leica, Diaplan, Bensheim, Germany). In the light optical mode, the image sections of specimens were chosen and image sequences were optimized on the monitor screen. Then the microscope was switched to the laser mode and the images were recorded. The morphological patterns of diffusion and dissolving depths were evaluated by one examiner using image editing software (Adobe Photoshop Elements, Version 5.1, Service Pack 3, Adobe Systems Incorporated, San Jose, CA, USA). Four images were recorded from each slice documenting a complex mapping of all areas. The dissolving depths were measured at four sites in each image.
During prior experiments, we had found that using adhesive resin without filler particles (e.g. Stick Resin ® , Stick Tech Ltd, Turku, Finland) made it more difficult to distinguish the dissolved layer than it was when using adhesive resin with filler particles ( Figs. 4 and 5 ). An adhesive (Optibond FL ® Adhesive, KerrHawe) containing hydroxyethylmethacrylate (HEMA) was used since Lastumäki et al. and Tezvergil et al. showed that HEMA can effectively dissolve the linear phases of semi-IPN, thus enhancing bond strength . With a size of 0.6 μm, the filler particles of Optibond FL ® are not able to diffuse into the semi-IPN. Only monomers dissolve the polymer and interlace into the interchain spacing which is between 5 and 10 Å . Consequently, the filler particle borderline marked the outer boundary of the dissolved layer. The internal boundary was clearly defined by non-infiltrated/non-fluorescent FRC ( Fig. 5 ). By using these distinct structures the examiner could more accurately define and measure the depths of interdiffusion ( Figs. 5–9 ).


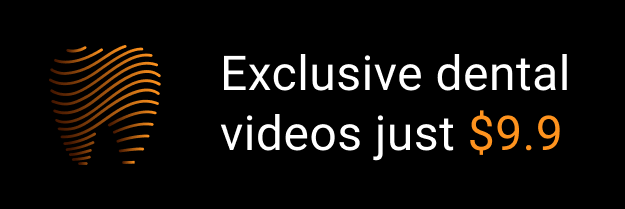