Abstract
Objectives
To evaluate the effect of adding a matrix metalloproteinase (MMP) inhibitor (BB94, Batimastat) to the primer of a three-step etch and rinse adhesive system on caries-affected dentin (CaD) MMP activity, and to assess the effect of such an inclusion on the chemical content of the CaD-adhesive interface.
Methods
Caries-infected dentin (CiD) was excavated selectively from freshly extracted human carious teeth using a chemo-mechanical agent. Each tooth was sectioned into three slabs through the CaD retained cavity. These were treated with either Optibond FL “OB” (Kerr, Orange, USA) without MMP inhibitor, or with 500 μM BB94 prior to the application of OB primer and bond, or with OB primer that contained 5 μM BB94. In situ zymography and Raman micro-spectroscopy were used to investigate MMP activity and the changes in the chemical content at the CaD/adhesive interface, respectively.
Results
Data showed the use of OB adhesive with BB94 resulted in immediate interfacial MMP inhibition, by direct application (93.3%) and by means of a drug delivery system (80%), as demonstrated by in situ zymography. Raman imaging revealed 33% higher resin infiltration into MMP-inhibited adhesive interfaces (SE 3.88).
Significance
Through competitive inhibition by batimastat (BB94), a proportion of the MMPs found in CaD were inhibited immediately and irreversibly. Such a competitive mechanism brings the adhesive primer close to the collagen matrix and enhances the dental adhesive wettability, which is a proposed mechanism to explain the presence of more resin within the hybrid layer.
1
Introduction
Contemporary minimally invasive, tooth-preserving biological operative caries management techniques encourage the excavation of the superficial caries-infected dentine (CiD) with the aim to preserve and seal the deeper caries-affected dentine (CaD), closer to the pulp so helping to maintain its long term vitality . More precisely, it is the often discolored, more superficial aspect of the CaD which is involved in the hybridization process with dental adhesive agents. This zone is characterized by the presence of a dense, partially exposed collagen matrix as a result of partial demineralisation and defibrillation of the unprotected collagen fibrils when compared to underlying sound dentin. This allows penetration of the dental adhesive and increased hybrid layer thickness . However, adhesive bonding to CaD results in a documented reduction of the micro-tensile bond strength when compared to that of sound dentin .
The acidic environment found within superficial CaD activates various intrinsic matrix metalloproteinases (MMPs) causing degradation of the organic collagen matrix as the lesion progresses . In addition, the use of acid-etch and/or an acidic adhesive primer in the restorative procedure leads to MMP activation within sound dentin . This suggests that the interface between the adhesive and CaD is subjected to an increase in MMP activity when compared to a sound dentin-adhesive interface, as a result of a combination of acidic environments.
Several techniques have been described to detect MMPs within the carious lesion. Using substrate zymography performed on excavated caries, MMP-2, MMP-8 and MMP-9 have been identified . Shimada and co-workers found that the quantity of immunogold-labeled MMP-8 and MMP-9 was reduced in CaD in comparison to sound dentin . However, there was no difference demonstrated in MMP-2 quantity between the two tissues. In situ zymography was used to detect MMP activity in carious dentin and to identify the sound dentin-adhesive interface MMP activity . The MMP activity of the CaD-adhesive interface has not been detected or described previously. This might be due to the irregularity and variability of the hybrid layer formed which makes its use difficult as a controlled substrate for laboratory investigation .
Recently, Raman spectroscopy has been used to detect chemical changes within carious dentin . Using the spectrum of selected points across a sound dentin-adhesive interface, Spencer et al. were able to detect and quantify the chemical composition, including the resin content of the interface for different adhesive systems . Furthermore, relative differences in penetration were observed for the adhesives’ constituents. The hydrophilic component (HEMA) infiltrated the demineralized sound dentin more than the hydrophobic component of the adhesive (bis-GMA) . Comparing the chemical changes within the CaD-adhesive interface and the sound dentin-adhesive interface, Wang et al. found that the former had a greater demineralization depth with a more diverse contents profile than the latter interface . Raman micro-spectroscopy has been used to measure the penetration depth and the degree of monomer conversion within sound dentin by identifying the dental adhesives’ specific peaks and recognizing the changes within them .
The aims of the present study were, firstly, to evaluate the effect of adding MMP inhibitor (BB94) to the primer of a three-step (Type 1) etch and rinse adhesive system on CaD MMP activity. Secondly, the effect of such an inclusion on the chemical content of the CaD-adhesive interface was assessed. The null hypotheses were that the addition of MMP inhibitor to the three-step etch and rinse adhesive system would not alter the CaD MMP activity or the chemical content of the CaD-adhesive interface at a statistical significance predetermined at α = 0.05.
2
Materials and methods
2.1
Sample preparation
Eight carious human teeth, ICDAS score “6+”, were collected using an ethics protocol reviewed and approved by the East Central London Research Ethics Committee (Reference 10/H0721/55). All teeth were stored in distilled water and used within one week after extraction. Caries-infected dentin was excavated selectively from the eight teeth with the aid of a chemo-mechanical agent, Carisolv ® gel and its specific hand instruments (OraSolv AB, Gothenburg, Sweden). The gel was applied and agitated as per manufacturer’s instructions, using the proprietary abrasive metal mace-tip instrument. Once the gel had become cloudy, it was rinsed away and a second fresh mix of gel was applied and further agitated. Excavation was deemed complete when the gel failed to become cloudy and the cavity was checked with a dental explorer for a rubbery but scratchy and discolored CaD consistency.
Each tooth was sectioned longitudinally through the CaD using a slow-speed water-cooled diamond blade (Diamond wafering blade XL 12205, Benetec Ltd., London, UK) into three 2 mm-thick dentin slabs per tooth. The sides of each slab were protected using a celluloid matrix strip. The CaD was acid etched using 35% phosphoric acid for 15 s and then rinsed with water then gently air-dried in order to remove excess water without overdrying the dentin substrate.
The three slabs were treated as follows: (1) the first slab (negative control) received Optibond FL “OB” (Kerr, Orange, USA) primer and bond, applied following the manufacturer’s instructions. (2) The second slab (positive control) was conditioned with 500 μM BB94 (British Biotech Ltd., Oxford, UK) prior to the application of OB primer and bond. (3) The third slab (experimental group) was treated with OB primer that contained 5 μM BB94, prepared as described previously by Almahdy et al. . The bond was applied on top of the conditioned CaD as per manufacturer’s instructions. All slabs were restored with Filtek™ Supreme Ultra resin composite (3 M ESPE, St. Paul, USA) applied using a flat plastic in two or three 2 mm-thick increments. A blue light source (470 nm, ∼600 mW cm −2 with a 10 mm tip, Optilux VLC, Demetron Research Co., CT, USA) was used to photo-activate the dental adhesives and each resin composite increment for 20 and 40 s respectively.
2.2
In situ zymography
Prior to the application of OB primer and bond, freshly reconstituted FITC-conjugated collagen (D-12060, Molecular Probes, Eugene, USA) was applied immediately, as an MMP substrate, onto the etched CaD of all slabs using a fully wicked microbrush. Additionally, Rhodamine B powder to 10 −3 % (w/v) was mixed with OB primer before its application in this experiment.
Each sample was stored at 37 °C at 100% humidity for 24 h. Prior to examination, each slab was hand-polished using 800, 1000 and 1200 grit SiC papers sequentially, with ultrasonication for 3 min between each paper grade. A confocal laser scanning microscope (CLSM; Leica TCS SP2, Leica Microsystems, Heidelberg GmbH, Germany) was used to evaluate the dentin slabs using a 100× 1.4NA oil immersion objective. A FITC fluorescence signal was obtained by exciting the samples with a 488 nm laser and the emission recorded through a 520–540 nm bandpass filter. Excitation by 568 nm laser and emission through a 600–630 nm filter was used to detect Rhodamine B fluorescence.
The presence of FITC signal within the hybrid layer of five reproducible, pre-selected areas (40 μm field width) was recorded in each slab. A total of 15 areas were included for each type of treatment group. The same slabs were stored in distilled water at 37 °C and were re-examined after two weeks using standardized settings. The emission of FITC signal represented the MMP activity as a result of the breakdown of the FITC-conjugated collagen ( Fig. 1 ).

2.3
CaD-adhesive interface characterization
Five extracted human carious teeth were used in this part of the study. Each sample was stored at 37 °C and 100% humidity for 24 h. Prior to examination, the excess resin composite was removed manually using 1000 grit SiC paper.
Using a Renishaw “inVia” Raman microscope (Renishaw Plc, Wotton-under-Edge, UK), each slab was examined with white light illumination and a 50 × 50 image montage was created with a 20× 0.4NA air objective. For each slab, two separate areas that contained resin composite, dental adhesive, hybrid layer, CaD and sound dentin tissue were selected. These areas were scanned in the StreamLine™ scanning mode using a 785 nm diode laser (100 mW laser power) through the 20× 0.4NA air objective while the Raman signal was acquired using a 600 line/mm grating centered between 849 cm −1 and 1603 cm −1 and 2 s CCD exposure time. The spectral resolution was 8.55 cm −1 .
Ten areas (700 μm × 1400 μm with 2.2 μm step size) were scanned non-destructively for each treatment group immediately (week 0). The slabs were stored in distilled water at 37 °C and were re-scanned after two weeks (week 2) and again after one month (week 4), using the same operating parameters. Pearson-based cluster analysis of the dataset (over 1.8 million spectra) was performed using an in-house program, described previously by Almahdy et al. . Average line profiles for all clusters were obtained across each sample starting from resin composite toward sound dentin. Scans that demonstrated at least 50 μm of resin composite zone at the beginning of the average line profile and at least 50 μm of sound dentin zone at the end, were included in the analysis. Any scan failing to show both contents was excluded (Supplement Fig. 1).
The profiles of one of the included and one of the excluded scans with their photo-micrographic appearance; (a) the included scans profile started with approximately 90% resin cluster in the resin composite area and then dropped as the scan move toward the sound dentin tissue. The sound dentin cluster (the other internal control) started near the CaD-adhesive interface and then kept increasing till the end of the scanned area. (b) One of the excluded scans that shows around 50% resin at the resin composite area and no sound dentin cluster was detected.
Photo-micrographs of the scanned area were used to determine the location of the hybrid layer and the average cluster content within this layer was calculated for all groups. Two-way ANOVA and Tukey’s post hoc test were used to compare the differences in the relative contribution of each cluster between the three groups at the three different times.
2
Materials and methods
2.1
Sample preparation
Eight carious human teeth, ICDAS score “6+”, were collected using an ethics protocol reviewed and approved by the East Central London Research Ethics Committee (Reference 10/H0721/55). All teeth were stored in distilled water and used within one week after extraction. Caries-infected dentin was excavated selectively from the eight teeth with the aid of a chemo-mechanical agent, Carisolv ® gel and its specific hand instruments (OraSolv AB, Gothenburg, Sweden). The gel was applied and agitated as per manufacturer’s instructions, using the proprietary abrasive metal mace-tip instrument. Once the gel had become cloudy, it was rinsed away and a second fresh mix of gel was applied and further agitated. Excavation was deemed complete when the gel failed to become cloudy and the cavity was checked with a dental explorer for a rubbery but scratchy and discolored CaD consistency.
Each tooth was sectioned longitudinally through the CaD using a slow-speed water-cooled diamond blade (Diamond wafering blade XL 12205, Benetec Ltd., London, UK) into three 2 mm-thick dentin slabs per tooth. The sides of each slab were protected using a celluloid matrix strip. The CaD was acid etched using 35% phosphoric acid for 15 s and then rinsed with water then gently air-dried in order to remove excess water without overdrying the dentin substrate.
The three slabs were treated as follows: (1) the first slab (negative control) received Optibond FL “OB” (Kerr, Orange, USA) primer and bond, applied following the manufacturer’s instructions. (2) The second slab (positive control) was conditioned with 500 μM BB94 (British Biotech Ltd., Oxford, UK) prior to the application of OB primer and bond. (3) The third slab (experimental group) was treated with OB primer that contained 5 μM BB94, prepared as described previously by Almahdy et al. . The bond was applied on top of the conditioned CaD as per manufacturer’s instructions. All slabs were restored with Filtek™ Supreme Ultra resin composite (3 M ESPE, St. Paul, USA) applied using a flat plastic in two or three 2 mm-thick increments. A blue light source (470 nm, ∼600 mW cm −2 with a 10 mm tip, Optilux VLC, Demetron Research Co., CT, USA) was used to photo-activate the dental adhesives and each resin composite increment for 20 and 40 s respectively.
2.2
In situ zymography
Prior to the application of OB primer and bond, freshly reconstituted FITC-conjugated collagen (D-12060, Molecular Probes, Eugene, USA) was applied immediately, as an MMP substrate, onto the etched CaD of all slabs using a fully wicked microbrush. Additionally, Rhodamine B powder to 10 −3 % (w/v) was mixed with OB primer before its application in this experiment.
Each sample was stored at 37 °C at 100% humidity for 24 h. Prior to examination, each slab was hand-polished using 800, 1000 and 1200 grit SiC papers sequentially, with ultrasonication for 3 min between each paper grade. A confocal laser scanning microscope (CLSM; Leica TCS SP2, Leica Microsystems, Heidelberg GmbH, Germany) was used to evaluate the dentin slabs using a 100× 1.4NA oil immersion objective. A FITC fluorescence signal was obtained by exciting the samples with a 488 nm laser and the emission recorded through a 520–540 nm bandpass filter. Excitation by 568 nm laser and emission through a 600–630 nm filter was used to detect Rhodamine B fluorescence.
The presence of FITC signal within the hybrid layer of five reproducible, pre-selected areas (40 μm field width) was recorded in each slab. A total of 15 areas were included for each type of treatment group. The same slabs were stored in distilled water at 37 °C and were re-examined after two weeks using standardized settings. The emission of FITC signal represented the MMP activity as a result of the breakdown of the FITC-conjugated collagen ( Fig. 1 ).
2.3
CaD-adhesive interface characterization
Five extracted human carious teeth were used in this part of the study. Each sample was stored at 37 °C and 100% humidity for 24 h. Prior to examination, the excess resin composite was removed manually using 1000 grit SiC paper.
Using a Renishaw “inVia” Raman microscope (Renishaw Plc, Wotton-under-Edge, UK), each slab was examined with white light illumination and a 50 × 50 image montage was created with a 20× 0.4NA air objective. For each slab, two separate areas that contained resin composite, dental adhesive, hybrid layer, CaD and sound dentin tissue were selected. These areas were scanned in the StreamLine™ scanning mode using a 785 nm diode laser (100 mW laser power) through the 20× 0.4NA air objective while the Raman signal was acquired using a 600 line/mm grating centered between 849 cm −1 and 1603 cm −1 and 2 s CCD exposure time. The spectral resolution was 8.55 cm −1 .
Ten areas (700 μm × 1400 μm with 2.2 μm step size) were scanned non-destructively for each treatment group immediately (week 0). The slabs were stored in distilled water at 37 °C and were re-scanned after two weeks (week 2) and again after one month (week 4), using the same operating parameters. Pearson-based cluster analysis of the dataset (over 1.8 million spectra) was performed using an in-house program, described previously by Almahdy et al. . Average line profiles for all clusters were obtained across each sample starting from resin composite toward sound dentin. Scans that demonstrated at least 50 μm of resin composite zone at the beginning of the average line profile and at least 50 μm of sound dentin zone at the end, were included in the analysis. Any scan failing to show both contents was excluded (Supplement Fig. 1).
The profiles of one of the included and one of the excluded scans with their photo-micrographic appearance; (a) the included scans profile started with approximately 90% resin cluster in the resin composite area and then dropped as the scan move toward the sound dentin tissue. The sound dentin cluster (the other internal control) started near the CaD-adhesive interface and then kept increasing till the end of the scanned area. (b) One of the excluded scans that shows around 50% resin at the resin composite area and no sound dentin cluster was detected.
Photo-micrographs of the scanned area were used to determine the location of the hybrid layer and the average cluster content within this layer was calculated for all groups. Two-way ANOVA and Tukey’s post hoc test were used to compare the differences in the relative contribution of each cluster between the three groups at the three different times.
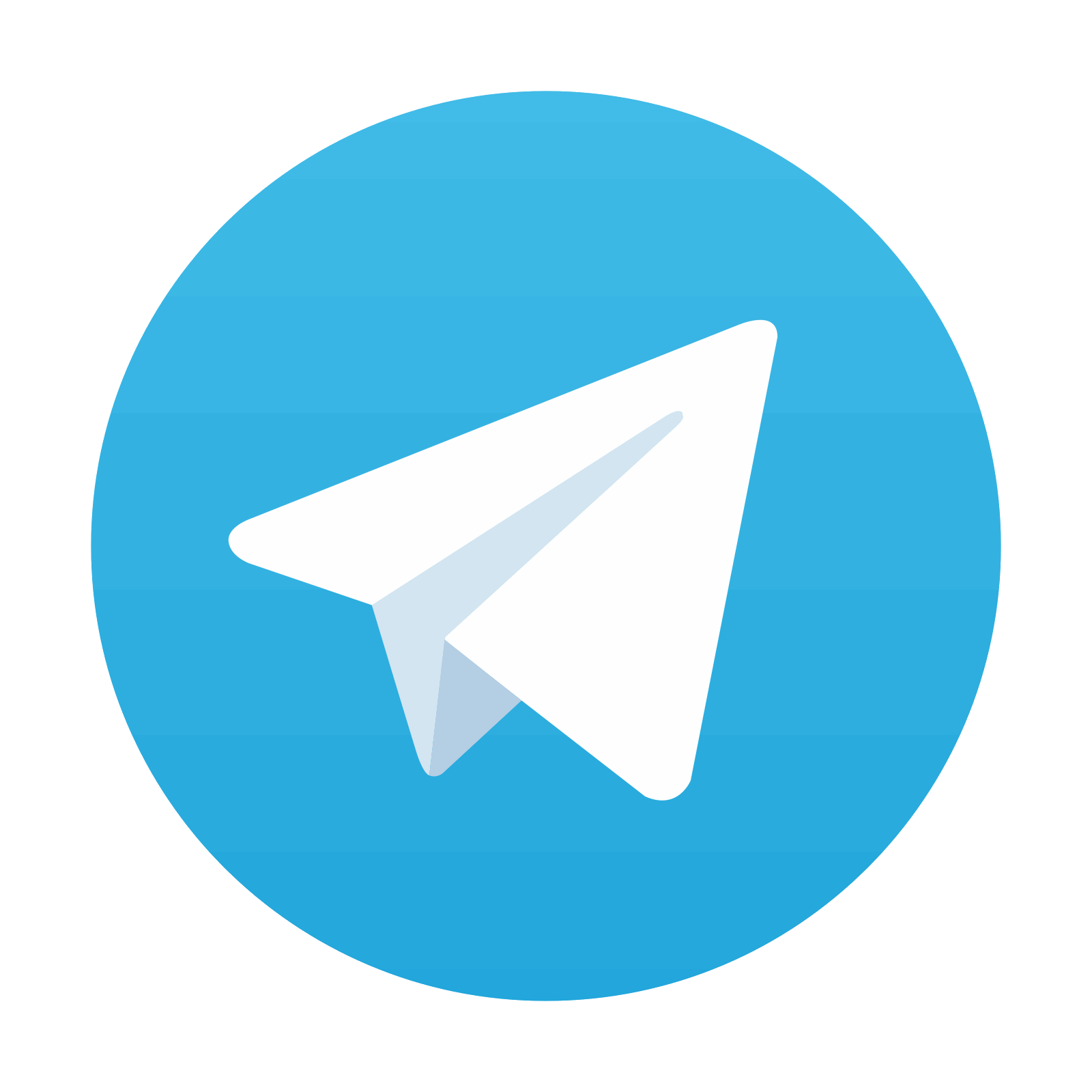
Stay updated, free dental videos. Join our Telegram channel

VIDEdental - Online dental courses
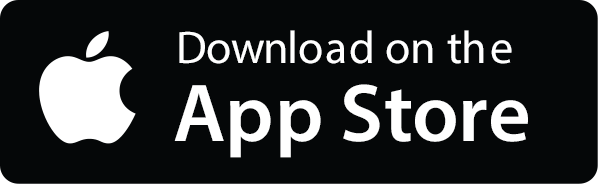
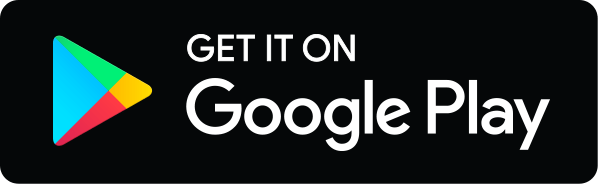