Several all-ceramic systems have been developed in dentistry to meet the increased expectations of patients and dentists for highly aesthetic, biocompatible, and long-lasting restorations. However, early bulk fractures or chippings have led the research community to investigate the mechanical performance of the all-ceramic systems. This overview explores the current knowledge of monolithic and bilayer dental all-ceramic systems, addressing composition and processing mechanisms, laboratory and clinical performance, and possible future trends for all-ceramic materials.
Over the last 3 decades, a trend to shift toward metal-free restorations has been observed in the dental field. To meet the increased demands of patients and dentists for highly aesthetic, biocompatible, and long-lasting restorations, several types of all-ceramic systems have been developed. Silicate and glass ceramics are used as a veneer for metal or all-ceramic cores to optimize form and aesthetics. In a monolithic application, small-sized restorations such as inlays, onlays, laminate veneers, and crowns can also be fabricated. High-strength ceramics such as aluminum and zirconium oxide ceramics were developed as a core material for crowns and fixed dental prostheses (FDPs) to extend the indication ranges to the high-load bearing areas. Most recently, monolithic zirconia restorations are increasingly promoted for single-crown and full-mouth rehabilitation, in particular for patients with parafunctional habits.
Because of advances in computer aided design (CAD) and computer aided manufacturing (CAM) technologies, the high-strength ceramic systems have become increasingly popular. Zirconia, specifically yttria-containing tetragonal zirconia polycrystal (Y-TZP), with unsurpassed mechanical properties ( Table 1 ), has had its clinical application expanded from single crowns and short-span FDPs to multiunit and full-arch zirconia frameworks as well as to implant abutments and complex implant superstructures to support fixed and removable prostheses. Zirconia in its pristine form can be considered as a reliable framework material with reported failure rates lower than those observed for silicate or alumina frameworks ( Table 2 ). However, questions regarding the overall success of bilayer zirconia/veneer restorations have been raised as a result of early chipping/fracture events observed while in oral function. As a result, various research groups have tried to better understand the potential problems such as the thermal interaction between the ceramic core and veneering porcelain, their respective processing techniques, and the effect of framework configuration on veneer support, in an attempt to overcome early chippings/fractures and enhance the long-term service of all-ceramic systems.
Material | Modulus (GPa) | Hardness (GPa) | Toughness (MPa m 1/2 ) | Strength (MPa) | CTE°C X × 10 −6 |
Firing (°C) |
---|---|---|---|---|---|---|
Porcelain | ||||||
Vitablocks (feldspathic) | 45 | NA | NA | 154 | 9.4 | 780–790 |
Lava Ceram | 78 | 5.3 | 1.1 | 100 | 10.5 | 810 |
IPS e.max ceram | 60–70 | 5.4 | NA | 90 | 9.5 | 750 |
IPS e.max ZirPress (flour-apatite) | 65 | 5.4 | NA | 110 | 10.5–11 | 900–910 |
Glass-ceramic | ||||||
Dicor | 75 | 3.4 | 1.4 | 290 | 9.8 | 850 |
Empress esthetic (leucite) | 65–68 | 6.2 | 1.3 | 160 | 16.6–17.5 | 625 |
IPS e.max Press (lithium disilicate) | 95 | 5.8 | 2.75 | 400 | 10.2–10.5 | 915–920 |
IPS e.max CAD (lithium disilicate) | 95 | 5.8 | 2.25 | 360 | 10.2–10.5 | 840 |
Alumina | ||||||
In-Ceram Alumina | 280 | 20 | 3.5 | 500 | 7.2 | 2053 melting point |
Procera Alumina | 340 | 17 | 3.2 | 695 | 7.0 | 1600 |
Zirconia | ||||||
Cercon | 210 | 12 | 9 | 1300 | 10.5 | 1350 |
IPS e.max Zir CAD |
210 | 13 | 5.5 | 900 | 10.8 | 1500 |
Lava | 210 | 14 | 5.9 | 1048 | 10.5 | 1480 |
DSC Zirkon | 210 | 12 | 7 | 1200 | 10.4 | 1500 |
In-Ceram YZ | 210 | 12 | 5.9 | >900 | 10.5 | 2706 melting point |
Procera Zirconia | 210 | 14 | 6 | 1200 | 10.4 | 1550 |
Prettau Zirkon Zirkonzahn | 210 | 12.5 | NA | 1000 | 10 | 1600 |
Tooth | ||||||
Dentin | 16 | 0.6 | 3.1 | — | 11–14 | — |
Enamel | 94 | 3.2 | 0.3 | — | 2–8 | — |
Study | Ceramic Materials | Observation Period (y) | Type of Restoration | Sample Size | Survival Rate (%) | Framework Fracture (%) | Veneer Fracture (%) |
---|---|---|---|---|---|---|---|
Cehreli et al, 2009 | Cercon Veneer n.s. |
2 | Single crowns | 15 | NS | 7 | 0 |
Groten & Huttig, 2010 | Cercon Cercon Ceram Kiss |
2 | Single crowns | 54 | 98 | 0 | 9 |
Ortorp et al, 2009 | Noble Procera Zirconia Vita Lumin Noble Rondo Zirconia |
3 | Single crowns | 204 | 92.7 | 0 | 2 |
Schmitt et al, 2010 | Lava Lava Ceram |
3 | Single crowns | 19 | 100 | 0 | 5 |
Schmitter et al, 2009 | Cercon Cercon Ceram S |
2 | 4- to 7-unit FDP | 30 | 96.6 | 3 | 3 |
Tsumita et al, 2010 | Cercon Creation ZI |
2 | 3-unit FDP | 21 | NS | 0 | 14 |
Sailer et al, 2009 | Cercon Cercon Ceram S |
3 | 3- to 5-unit FDP | 38 | 100 | 0 | 25 |
Beuer et al, 2009 | Cercon Cercon Ceram Express |
3 | 3-unit FDP | 21 | 90.5 | 5 | 0 |
Roediger et al, 2010 | Cercon Cercon Ceram S Experimental veneering ceramic |
4 | 3- to 4-unit FDP | 99 | 94 | 1 | 13 |
Wolfart et al, 2009 | Cercon Cercon Ceram S Cercon Ceram Express |
4 | 3- to 4-unit FDP/Cantilever FDP | 24 34 |
96 92 |
0 0 |
13 12 |
Sailer et al, 2007 | Cercon Experimental veneering ceramic |
5 | 3- to 5-unit FDP | 57 | 73.9 | 8 | 15 |
Crisp et al, 2008 | Lava Lava Ceram |
1 | 3- to 4-unit FDP | 38 | 100 | 0 | 3 |
Raigrodski et al, 2006 | Lava Veneer n.s. |
2.5 | 3-unit FDP | 20 | NS | 0 | 25 |
Schmitt et al, 2009 | Lava Lava Ceram |
3 | 3- to 4-unit FDP | 27 | 100 | 0 | 11 |
Vult von Steyern et al, 2005 | DC Zirkon Vita D |
2 | 3- to 5-unit FDP | 20 | NS | 0 | 15 |
Tinschert et al, 2008 | DC Zirkon Vita D |
3 | 3- to 10-unit FDP/Cantilever FDP | 65 | NS | 0 | 6 |
Edelhoff et al, 2008 | Digident Initial ZirKeramik GC |
3 | 3- to 6-unit FDP | 21 | 90.5 | 0 | 10 |
Molin and Karlsson, 2008 | Denzir Vita D IPS Empress |
5 | 3-unit FDP | 19 | 100 | 0 | 36 |
Nothdurft & Pospiech, 2009 | Cercon Cercon Ceram Kiss |
0.5 | Implant-supported single crowns | 40 | NS | 0 | 8 |
Larsson et al, 2006 | Denzir Esprident Triceram |
1 | Implant-supported FDP | 13 | NS | 0 | 53 |
Larsson et al, 2010 | Cercon Cercon Ceram S |
3 | Implant-supported full-arch FDP | 10 | 100 | 0 | 34 |
This overview presents the current knowledge of monolithic and bilayer all-ceramic systems and addresses composition and processing mechanisms, laboratory and clinical performance, and possible future trends.
Reinforced glass ceramics
The interest in nonmetallic and biocompatible restorative materials increased after the introduction of the feldspathic porcelain crown in 1903 by Land. In 1965, McLean pioneered the concept of adding aluminum oxide to feldspathic porcelain in an attempt to enhance mechanical properties. However, the clinical shortcomings of these materials, such as brittleness, crack propagation, low tensile strength, wear resistance, and marginal accuracy, discontinued its use.
Increased strength in glassy ceramics can be achieved by adding appropriate fillers that are uniformly dispersed throughout the glass, a technique termed dispersion strengthening. Leucite is used as a reinforcing crystalline at a concentration of 35 to 45 vol%. In the early 1990s, the lost wax press technique was introduced to dentistry as an innovative processing method for all-ceramic restorations. Examples of leucite-reinforced glass ceramics are VITA VMK 68 (VITA Zahnfabrik, Bad Säckingen, Germany), Finesse All-Ceramic, (Dentsply, York, PA, USA), Optec OPC (Jeneric, Wallingford, CT, USA), and IPS Empress (Ivoclar Vivadent, Schaan, Principality of Liechtenstein). The molding procedure of IPS Empress is conducted at 1080°C in a special, automatically controlled furnace. Leucite crystals are formed through a controlled surface crystallization process in the SiO 2 -Al 2 O 3 -K 2 O glass system. Tangential compressive stresses develop around the crystals on cooling because of the difference in the coefficient of thermal expansion (CTE) between leucite crystals and the glassy matrix. These stresses contribute to crack deflection and improved mechanical performance. IPS Empress, for instance, exhibits a flexural strength of 120 to 180 MPa and a CTE of 15 to 18.5 × 10 −6 K −1 m/m. The material is suitable for fabrication of inlays, onlays, veneers, and crowns. Favorable clinical long-term data with high survival rates are described for IPS Empress inlays, onlays (90% after 8 years ), veneers (94.4% after 12 years ), and crowns (95.2% after 11 years ) in the dental literature.
Leucite glass ceramics can also be machined with various CAD/CAM systems. Multicolored blocks were recently developed to reproduce color transitions and shading as well as different levels of translucency to reproduce natural teeth ( Fig. 1 ). The use of leucite-reinforced glass ceramic has significantly declined because of the introduction of lithium disilicate glass ceramics with significantly improved mechanical and aesthetic properties and is addressed in detail in the next section.
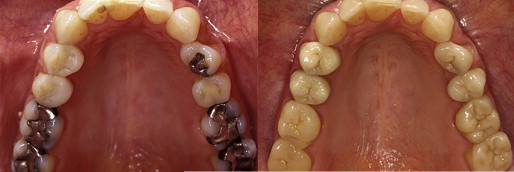
Lithium disilicate glass ceramics
A significantly higher strength of 350 MPa was achieved with a glass ceramic of the SiO 2 -Li 2 O-K 2 O-ZnO-P 2 O 5 -Al 2 O 3 -La 2 O 3 system by precipitating lithium disilicate (Li 2 Si 2 O 5 ) crystals. The crystal content of up to 70 vol% is considerably higher than that of leucite materials. High-temperature x-ray diffraction studies revealed that both lithium metasilicate (Li 2 SiO 3 ) and crystobalite form during the crystallization process before the growth of lithium disilicate (Li 2 Si 2 O 5 ) crystals. The final microstructure consists of highly interlocked lithium disilicate crystals, 5 μm in length and 0.8 μm in diameter. Thermal expansion mismatch between lithium disilicate crystals and glassy matrix results in tangential compressive stresses around the crystals, potentially responsible for crack deflection and strength increase. Crystal alignment after heat pressing of the lithium disilicate glass ceramic leads to multiple crack deflections. The lithium disilicate ceramic was introduced as IPS Empress 2 (Ivoclar Vivadent) in 1998 and is moldable as leucite glass ceramics, but at a lower temperature of 920°C. The CTE is 10.5 × 10 −6 K −1 m/m.
High survival rates were observed for anterior and posterior IPS Empress 2 crowns (95.5% after 10 years ) in long-term clinical studies ( Fig. 2 ). The clinical success of 3-unit IPS Empress 2 FDPs is predominately limited by bulk fracture failure within the connector area, in particular when the connector size is less than the recommended dimensions (70% survival rate after 5 years ) ( Fig. 3 ).
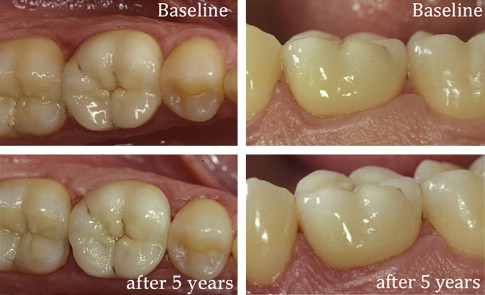
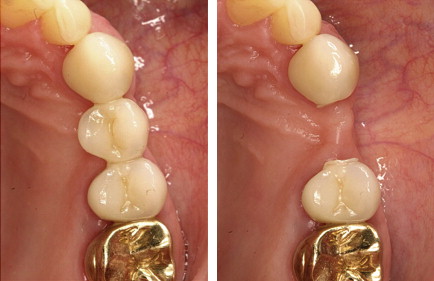
A newly developed pressable lithium disilicate glass ceramic (IPS e.max Press, Ivoclar Vivadent) with improved physical properties (flexural strength, 440 MPa) and translucency through different firing process has been developed in the SiO 2 -Li 2 O-K 2 O-ZnO-P 2 O 5 -Al 2 O 3 -ZrO 2 system. The pressable lithium disilicate ceramic can be used in monolithic application for inlays, onlays, and posterior crowns or as a core material for crowns and 3-unit FDPs in the anterior region. Apatite glass ceramics are recommended for veneering.
Clinical data exhibited high survival rates on IPS e.max Press onlays (100% after 3 years), crowns (96,6% after 3 years), monolithic inlay retained FDPs (100% after 4 years), and full crown retained FDPs (93% after 8 years).
Recently, a lithium disilicate glass ceramic (IPS e.max CAD, Ivoclar Vivadent) has been designed for CAD/CAM processing technology. The milled lithium disilicate block is exposed to a 2-stage crystallization process. Lithium metasilicate crystals are precipitated during the first stage. The resulting glass ceramic has a crystal size range of 0.2 to 1.0 μm with approximately 40 vol% lithium metasilicate crystals. At this precrystallized state, the CAD/CAM block exhibits a flexural strength of 130 to 150 MPa, which allows simplified machining and intraoral occlusal adjustment. The final crystallization process occurs after milling of the restoration at 850°C in vacuum. The metasilicate crystal phase is dissolved completely, and the lithium disilicate crystallizes. This process also converts the blue shade of the precrystallized block to the selected tooth shade and results in a glass ceramic with a fine grain size of approximately 1.5 μm and a 70% crystal volume incorporated in a glass matrix. CAD/CAM-processed lithium disilicate glass ceramic demonstrates a flexural strength of 360 MPa (see Table 1 ). Because of the favorable translucency and shade assortment the material can be used for fully anatomic (monolithic) restorations with subsequent staining characterization or as a core material with subsequent coating with veneering ceramics. The manufacturer recommends its use for anterior or posterior crowns, implant crowns, inlays, onlays, and veneers. Preliminary clinical results on single crowns revealed high survival rates (100% after 2 years ) and are hence extremely promising.
Lithium disilicate glass ceramics
A significantly higher strength of 350 MPa was achieved with a glass ceramic of the SiO 2 -Li 2 O-K 2 O-ZnO-P 2 O 5 -Al 2 O 3 -La 2 O 3 system by precipitating lithium disilicate (Li 2 Si 2 O 5 ) crystals. The crystal content of up to 70 vol% is considerably higher than that of leucite materials. High-temperature x-ray diffraction studies revealed that both lithium metasilicate (Li 2 SiO 3 ) and crystobalite form during the crystallization process before the growth of lithium disilicate (Li 2 Si 2 O 5 ) crystals. The final microstructure consists of highly interlocked lithium disilicate crystals, 5 μm in length and 0.8 μm in diameter. Thermal expansion mismatch between lithium disilicate crystals and glassy matrix results in tangential compressive stresses around the crystals, potentially responsible for crack deflection and strength increase. Crystal alignment after heat pressing of the lithium disilicate glass ceramic leads to multiple crack deflections. The lithium disilicate ceramic was introduced as IPS Empress 2 (Ivoclar Vivadent) in 1998 and is moldable as leucite glass ceramics, but at a lower temperature of 920°C. The CTE is 10.5 × 10 −6 K −1 m/m.
High survival rates were observed for anterior and posterior IPS Empress 2 crowns (95.5% after 10 years ) in long-term clinical studies ( Fig. 2 ). The clinical success of 3-unit IPS Empress 2 FDPs is predominately limited by bulk fracture failure within the connector area, in particular when the connector size is less than the recommended dimensions (70% survival rate after 5 years ) ( Fig. 3 ).
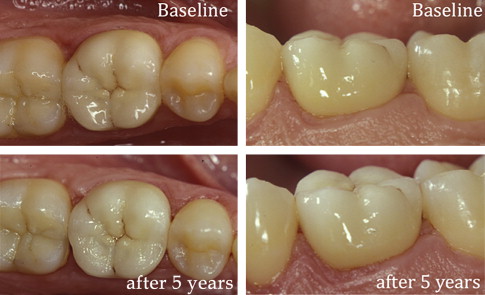
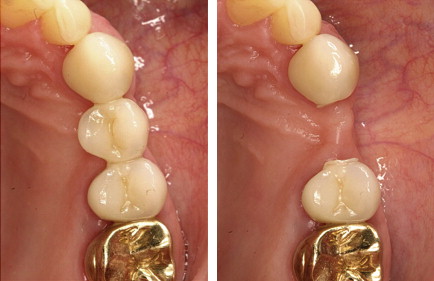
A newly developed pressable lithium disilicate glass ceramic (IPS e.max Press, Ivoclar Vivadent) with improved physical properties (flexural strength, 440 MPa) and translucency through different firing process has been developed in the SiO 2 -Li 2 O-K 2 O-ZnO-P 2 O 5 -Al 2 O 3 -ZrO 2 system. The pressable lithium disilicate ceramic can be used in monolithic application for inlays, onlays, and posterior crowns or as a core material for crowns and 3-unit FDPs in the anterior region. Apatite glass ceramics are recommended for veneering.
Clinical data exhibited high survival rates on IPS e.max Press onlays (100% after 3 years), crowns (96,6% after 3 years), monolithic inlay retained FDPs (100% after 4 years), and full crown retained FDPs (93% after 8 years).
Recently, a lithium disilicate glass ceramic (IPS e.max CAD, Ivoclar Vivadent) has been designed for CAD/CAM processing technology. The milled lithium disilicate block is exposed to a 2-stage crystallization process. Lithium metasilicate crystals are precipitated during the first stage. The resulting glass ceramic has a crystal size range of 0.2 to 1.0 μm with approximately 40 vol% lithium metasilicate crystals. At this precrystallized state, the CAD/CAM block exhibits a flexural strength of 130 to 150 MPa, which allows simplified machining and intraoral occlusal adjustment. The final crystallization process occurs after milling of the restoration at 850°C in vacuum. The metasilicate crystal phase is dissolved completely, and the lithium disilicate crystallizes. This process also converts the blue shade of the precrystallized block to the selected tooth shade and results in a glass ceramic with a fine grain size of approximately 1.5 μm and a 70% crystal volume incorporated in a glass matrix. CAD/CAM-processed lithium disilicate glass ceramic demonstrates a flexural strength of 360 MPa (see Table 1 ). Because of the favorable translucency and shade assortment the material can be used for fully anatomic (monolithic) restorations with subsequent staining characterization or as a core material with subsequent coating with veneering ceramics. The manufacturer recommends its use for anterior or posterior crowns, implant crowns, inlays, onlays, and veneers. Preliminary clinical results on single crowns revealed high survival rates (100% after 2 years ) and are hence extremely promising.
Glass-infiltrated ceramics
Another group of all-ceramic systems involves glass-infiltrated ceramics. In-Ceram Alumina (VITA Zahnfabrik) was the first all-ceramic system available for single-unit restorations and 3-unit anterior bridges with a high-strength ceramic core fabricated with the slip-casting technique. A slurry of densely packed (70–80 wt%) Al 2 O 3 is applied and sintered to a refractory die at 1120°C for 10 hours. This process produces a porous skeleton of alumina particles, which is infiltrated with lanthanum glass in a second firing at 1100°C for 4 hours to eliminate porosity and increase strength. The core is veneered with feldspathic porcelain. In-Ceram Zirconia (VITA Zahnfabrik) is a modification of the original In-Ceram Alumina system, with an addition of 35% partially stabilized zirconia oxide to the slip composition to strengthen the ceramic. Traditional slip-casting techniques can be used, or the material can be copy-milled from prefabricated, partially sintered blanks and then veneered with feldspathic porcelain. As the core is opaque and lacks translucency, the use of this material for anterior regions becomes problematic. Clinical performance of glass-infiltrated ceramics for crowns and FDPs indication has been evaluated in a systematic review. However, with the development of high-strength ceramics, recent literature on glass-infiltrated ceramics became sparse.
High-strength oxide ceramics
Although high-strength ceramic in dentistry includes alumina and zirconia, this section focuses mainly on zirconia because of its superior mechanical properties compared with alumina and the current controversial aspects of its clinical performance.
Zirconia ceramics have gained a remarkable interest in biomedical sciences. The first reference concerning their application in medicine appeared in the late sixties; 20 years later, Christel and colleagues studied their use in orthopedic surgery. In the early 1990s, zirconia was introduced to dentistry, and in recent years, a large number of publications appeared in the literature.
Zirconia, characterized by a dense, monocrystalline homogeneity, possesses low thermal conductivity, low corrosion potential, and good radiopacity. High biocompatibility, low bacterial surface adhesion, and favorable optical properties of zirconia ceramics are reported. Zirconia in its pure form is a polymorph that has 3 temperature-dependant phases that are monoclinic (room temperature to 1170°C), tetragonal (1170°C–2370°C), and cubic (2370°C to melting point). With the addition of stabilizing oxides such as magnesia, ceria, yttria, and calcium to zirconia, the tetragonal phase is retained in a metastable condition at room temperature, enabling a phenomenon called transformation toughening to occur. In response to mechanical stimuli, the partially stabilized crystalline tetragonal zirconia transforms to the more-stable monoclinic phase, with a local volume increase of approximately 4%. This increase in volume counteracts further crack propagation by compression at the tip of the crack. Compared with high-strength alumina ceramic, zirconia has twice the flexural strength (900–1200 MPa). In addition, high fracture toughness (9–10 MPa∙m 1/2 ) has been described. The most commonly used dental zirconia formulations are glass-infiltrated zirconia toughened alumina ceramics (In-Ceram, VITA Zahnfabrik) and 3 mol% Y-TZP. Y-TZP has been used for root canal posts, frameworks for posterior teeth, implant-supported crowns, multiunit FDPs, resin-bonded FDPs, custom-made bars to support fixed and removable dental prostheses, implant abutments, and dental implants.
A magnesium-doped partially stabilized zirconia (Mg-PSZ, Denzir M, Dentronic AB, Sweden) is available, but porosity and large grain size has limited its success.
Zirconia and CAD/CAM Technology
An array of CAD/CAM systems has evolved since F. Duret introduced the concept in 1971. Some CAD/CAM systems (Denzir, Cadesthetics, Skellefteå, Sweden, and DC-Zircon, DCS Dental AG, Allschwil, Switzerland) machine fully sintered Y-TZP blocks, which have been processed by hot isostatic pressing. Because of the hardness and poor machinability of fully sintered Y-TZP, a robust milling system and extended milling periods are required. Most of the available CAD/CAM systems shape blocks of partially sintered zirconia. Milling from partially sintered blocks involves machining enlarged frameworks in a so-called green state. These blocks are then sintered to their full strength, which is accompanied by shrinkage of the milled framework by approximately 25% to the desired dimensions. Examples of these systems are CERCON (Dentsply Friadent, Mannheim, Germany), LAVA (3M ESPE, Seefeld, Germany), Procera (Nobel Biocare, Gothenburg, Sweden), Etkon (Straumann, Basel, Switzerland), and Cerec (Sirona, Bensheim, Germany).
The advantage of industrialized blank fabrication and reproducible and consistent CAM resulted in increased process reliability and cost-effectiveness of CAD/CAM-fabricated restorations. Labor-intensive waxing, casting, and soldering of frameworks accompanied with conventional laboratory procedures can be avoided with the application of CAD/CAM technology. With extensive frameworks, particularly used in implant dentistry, and the escalating costs of precious alloys, all-ceramic restorations are competitive with conventional metal-ceramic restorations (MCRs) from a cost perspective.
Zirconia and Low-Temperature Degradation
A major drawback of zirconia restorations compared with metal-ceramics is the material-inherent accelerated aging that has been observed to occur in zirconia in the presence of moisture. This aging phenomenon is known as low-temperature degradation (LTD) and was first described by Kobayashi and colleagues in 1981. At relatively low temperatures (150°C–400°C), slow tetragonal to monoclinic transformations occur, initiating at the surface of polycrystalline zirconia and subsequently progressing into the bulk of the material. Transformation of 1 grain is accompanied by an increase in volume, which causes stress on the surrounding grains and microcracking. Water penetration into these cracks then exacerbates the process of surface degradation, and the transformation progresses. The growth of the transformation zone results in severe microcracking, grain pullout, and finally surface roughening, which ultimately leads to strength degradation. Any factor that is detrimental to the stability of tetragonal zirconia is susceptible to promote LTD. Among these factors are grain size, the amount of stabilizer, and the presence of residual stress. At present, there is no clear correlation between LTD and failure predictability when zirconia is used as a dental bioceramic.
Clinical Data on Zirconia-Supported Restorations
Although zirconia was introduced to dentistry in the early 1990s, limited information about its clinical performance can be found. A literature search from 1990 through September 2010 using electronic databases (PubMed and MEDLINE) revealed a total of 21 clinical trials on zirconia-supported restorations (see Table 2 ). Long-term clinical data for zirconia-supported restorations with observation periods exceeding 5-year time frames are not yet available. Most studies (n = 14) investigated predominately posterior FDPs, whereas a small number focused on (n = 4) crowns. Only 3 studies described the clinical behavior of implant-supported zirconia-supported FDPs and single crowns.
Veneered Zirconia Clinical Failure Modes
Core/framework fractures
Catastrophic fractures within the zirconia core ceramic are reported at 7% for single crowns after 2 years and at 1% to 8% for FDPs after 2 to 5 years (see Table 2 ). Occlusal overloading caused by bruxism (crown fracture after 1 month ) or trauma (connector fracture 5-unit FDP after 38 months ) and insufficient framework thickness at 0.3 mm (crown-abutment fractures in 3-unit FDP ) were mentioned as the main reasons for zirconia core bulk fractures. Fractographic analyses of clinically failed zirconia crowns showed that radial fractures propagating upwards from the cementation surface site resulted in bulk fractures and caused catastrophic failures of the restorations. Microscopic examinations of failed zirconia-based FDPs revealed that core bulk fractures were most commonly located in the connector area and initiated from the gingival surface, where tensile stresses were the greatest because of occlusal loading. Core bulk fracture in the connector area was also attributed to damage induced during fabrication. A higher susceptibility to connector fracture was noted with an increased span of FDPs.
Veneering ceramic cohesive fractures
The major drawbacks noted in all clinical studies on zirconia-supported restorations were less related to the framework integrity than to wear and failure of the veneering ceramics. Cohesive fractures within the veneering ceramic (chipping) were described as the most frequent reason for failures, irrespective of the applied zirconia veneer system (see Table 2 ). Veneer fracture rates are reported at 2% to 9% for single crowns after 2 to 3 years and at 3% to 36% for FDPs after 1 to 5 years. Implant-supported zirconia-based restorations revealed even higher rates at 8% for single crowns after 6 months and at 53% for FDPs after 1 year. Impaired proprioception and rigidity of osseointegrated implants correlated with higher functional impact forces might further exacerbate cohesive veneer fractures. To date, only one 3-year prospective clinical study involving implant-supported full-arch Y-TZP reconstructions is available. Despite the short follow-up period and limited number of patients (n = 10), no framework fractures were observed; however, chip-off fractures were observed in 34% of the units cemented in 9 of 10 patients.
Depending on the size and localization, cracks leading to veneer fractures can severely compromise the aesthetics and function of zirconia-supported restorations. Fractographic analysis of clinically failed veneered zirconia restorations revealed cohesive veneer failures, with cracks originating from the occlusal surface and propagating to the core veneer interface, leaving an intact core. In many clinical studies, it seemed that these veneer failures were associated with roughness in the veneering ceramic because of occlusal function or occlusal adjustment. Therefore, special attention has to be paid to the static and dynamic aspects of occlusion in zirconia-based restorations. Occlusal adjustments should be performed only with fine-grain diamonds under water irrigation followed by a thorough polishing sequence.
Reasons for veneering ceramic failure
As the veneering ceramic material (flexural strength, approximately 90–120 MPa) is weak compared with the high-strength core material (900–1200 MPa), the veneering ceramic seems to be prone to failure at low loads during masticatory function. The use of higher-strength veneering ceramic was proposed to reduce the incidence of veneer chippings/fractures. However, attempts to improve the microstructure and mechanical properties of veneering ceramics with the development of glass-ceramic ingots for pressing veneering ceramics onto zirconia frameworks did not result in an increased reliability of the veneering ceramic. In addition, identical chip-failure patterns were observed. Although a high density of the veneering layer has been expected with the press technique, spherical porosities were noted within the veneer layer and also at the interface. In clinical trials on zirconia FDPs, chipping fractures have also been reported with pressable veneering ceramics, and the chipping problem does not seem to be solved.
Moreover, the CTE is frequently discussed as a contributing factor for veneer failure. However, the problem of chipping may not be limited only to the mismatch of CTE; it seems more complex. Residual stresses in bilayer crowns and FDPs are associated with the possibility of thermal gradients being developed in these structures during cooling. For zirconia veneer all-ceramic systems, the low thermal conductivity of the zirconia (approximately 3 Wm/K) results in the highest temperature difference and, therefore, very high residual stresses. In addition, thick layers of veneering ceramics on zirconia cores are highly susceptible to generating high tensile subsurface residual stresses, ultimately resulting in unstable cracking or chipping. Slow cooling of the restoration above the glass transition temperature of the porcelain could prevent the development of high tensile subsurface residual stresses in the porcelain, which may result in unstable cracking or chipping. Most manufacturers now recommend the approach of a reduced cooling rate after final firing or glazing, and even an additional 6 minutes has shown to be effective.
Zirconia framework design
The lack of a uniform support of the veneering ceramic because of improper framework design has been discussed as a possible reason for chipping fractures. Remarkably little scientific clinical data on optimal design of zirconia-supported restorations have been published. With the introduction of CAD/CAM technologies in dentistry, excessive veneer layer thickness (>2.5 mm) was created because of the uniform layer thickness of the copings for crowns and bar-shaped connectors for FDPs. Improved customized zirconia coping design derived from the conventional porcelain fused to metal technique has been recommended to provide adequate support for the veneering ceramic. A dual-scan procedure of the die and full-contour wax pattern has been merged to fabricate the desired framework. Preliminary in vitro studies showed that cohesive fractures within the veneering ceramic could not be avoided with the improved support, but the size of the fractures was significantly decreased and failure initiation was shifted toward higher loads. In clinical observation, conflicting results on the framework design modification have been described. Hence, the effect of framework design modifications on residual stress states needs to be better elucidated.
Minimizing Zirconia Core Failures
Some fundamental aspects affecting the clinical performance of zirconia as a framework material need to be addressed. Laboratory technicians and clinicians should follow the precise sequence steps in manufacturing zirconia-based restorations, with the knowledge that zirconia as a framework material is potentially damaged by surface modifications and improper laboratory and clinical handling techniques.
Grinding or sandblasting of surfaces with high (or mild/low) pressure ranges is implicated as a factor in inducing the formation of surface microcracking that could be detrimental to the long-term performance of the restorations and lead to unexpected failures. Several research groups have claimed an increased flexural strength in Y-TZP because of phase transformation after grinding and alumina abrasion. However, any temporary beneficial effect related to phase transformation induced by different surface treatment methods may be negated by fatigue-related crack growth phenomena and following heat treatments associated with veneer application. With respect to the highly deleterious effect on zirconia reliability, postsintering surface modifications of zirconia frameworks at the dental laboratory or under clinical circumstances should be avoided.
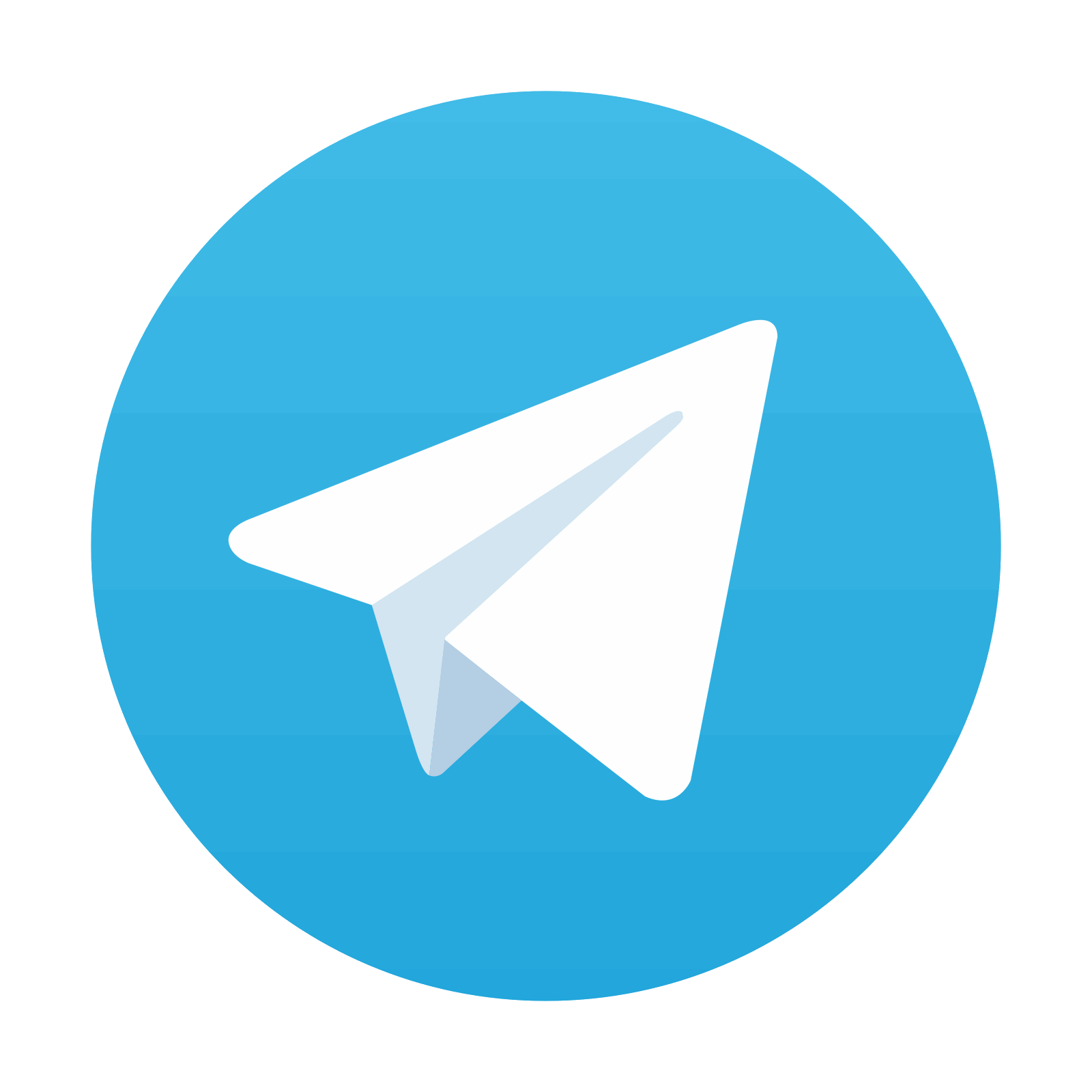
Stay updated, free dental videos. Join our Telegram channel

VIDEdental - Online dental courses
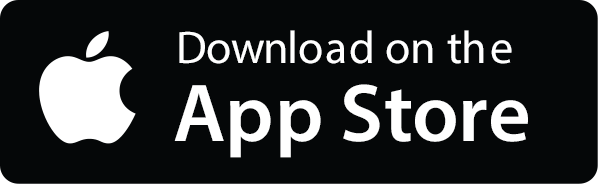
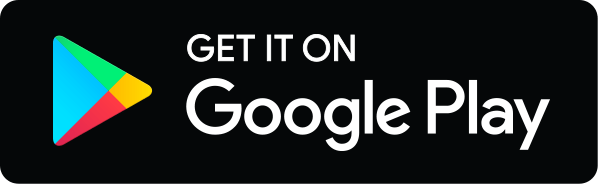