Abstract
Objectives
Bond strength between adhesive systems and the tooth structure is influenced by a large number of variables, which makes the comparison among studies virtually impossible. Also, failure often times propagates into the dental substrate or the composite, deeming the results questionable at best. In spite of the increased popularity gained by micro-tensile and micro-shear tests, in vitro evaluations using specimens with relatively large bonding areas remain frequent. This review focuses on aspects related to specimen geometry and test mechanics of “macro” shear and tensile bond strength tests.
Methods
Besides information drawn from the literature, the effect of some parameters on stress distribution at the bonded interface was assessed using finite element analysis (FEA).
Results
Bond strength tends to increase with smaller bonding areas and with the use of high elastic modulus composites. Stress concentration at the bonded interface is much more severe in shear compared to tension. Among shear methods, the use of the chisel shows the highest stress concentration. Within the limits suggested by the ISO/TS 11405, crosshead speed does not seem to influence bond strength values. Pooled data from currently available adhesives tested in either shear or tension showed 44% of adhesive failures, 31% mixed and 25% cohesive in the substrate (tooth or composite). A comparative bond strength study involving three adhesive systems revealed similarities between “macro” and “micro” counterparts regarding material ranking, whereas “macro” tests presented a higher incidence of cohesive failures.
Significance
Simplicity warrants “macro” bond strength tests an enduring popularity, in spite of their evident limitations. From a mechanical standpoint, knowing the stress distribution at the bonded interface and how it is affected by the materials and loading method used is key to explain the results.
1
Introduction
Over the years, clinicians have relied upon laboratory evaluations to choose which adhesive systems to use in their daily practice. Though the validity of bond strength tests to predict clinical performance of dental adhesives is questionable , recent evidence shows that clinical results can, to some extent, be estimated based upon laboratory results . Moreover, mechanical testing of bonded interfaces has provided some valuable information in terms of identifying substrate variables and helping define guidelines for application procedures .
Until the mid-nineties, shear and tensile bond strength tests were performed exclusively in specimens with relatively large bonded areas, usually 3–6 mm in diameter (approximately 7–28 mm 2 ). However, the validity of expressing bond strength in terms of nominal ( i.e ., average) stress has been questioned due to the heterogeneity of the stress distribution at the bonded interface . Moreover, cohesive failure of both the composite and the dental substrate is a common occurrence, precluding an accurate assessment of the interfacial bond strength . The need for new methods to overcome these limitations led to the use of specimens with small bonding areas ( i.e ., below 2 mm 2 ), in the so-called micro-tensile and micro-shear tests .
In spite of the increased popularity of the “micro” bond strength tests and the criticism endured by the conventional tensile and shear methods, the number of articles using “macro” tests published in recent years remains high, meaning that a lot of the available data on dental adhesion still comes from mechanical tests performed in specimens with large bonded areas ( Fig. 1 ). The preference for conventional shear and tensile tests is justified because they are easy to perform, requiring minimal equipment and specimen preparation. However, though a lot of information can be found on specimen geometry and other testing variables for “micro” bond strength tests , the same is not true for the “macro” tensile and shear tests.

Reviews published in the past evidenced a concern with the bonding substrate and specimen storage conditions, only minimally discussing the variables of the mechanical test itself . The International Organization for Standardization (ISO) technical specification ISO/TS 11405 , published in 1994 and last revised in 2003, reflects this tendency, describing with greater detail the characteristics and preparation of the tooth substrate for the bonding procedure, and leaving aspects such as bonding area, testing assemblies or loading conditions more vague. As a result, a wide variety of experimental protocols is found among researchers, with evident effect on the outcomes . A systematic review with meta-analysis identified composite type, bonding area, testing mode (shear, tensile or micro-tensile) and crosshead speed as factors that significantly influence bond strength, along with several others related to the substrate, specimen storage conditions and thermocycling . The type of device used for load application was also shown to affect the results .
The purpose of this article was to review aspects related to specimen geometry and test mechanics that may influence “macro” shear and tensile bond strength results. Finite element analysis (FEA) was used to assess the effect of selected variables on the stress distribution at the bonded interface. Specimen failure mode was discussed based on data retrieved from the recent literature. Also, a brief comparison between “macro” and “micro” bond strength tests was performed using experimental data obtained with three adhesive systems.
2
A survey of recent bond strength studies
In order to verify how researchers are currently performing tensile and shear bond strength tests, 100 studies published between 2007 and 2009 (shear: 74, tensile: 26) were surveyed . Unsurprisingly, no consensus was found in any of the parameters observed.
Most studies used bonding areas between 3 and 4 mm in diameter (57%), while 4% did not include this information. These numbers do not differ from those reported 12 years ago, where the mean diameter among 50 studies was 3.97 mm and 6% of the studies omitted this information . Pre-formed rods were used in 31% of the tensile tests and none of the shear tests. Composite height varied between 2 and 5 mm. A description of the testing method was included in 66% of the studies. For shear loading, the knife-edge rod was used in half of the studies describing the experimental assembly utilized. All studies reported the crosshead speed, with 0.5 and 1.0 mm/min being the most frequent values (46 and 41%, respectively), also in agreement with a recent survey .
Specimen failure mode analysis was present in 64% of the studies, performed either under low magnification (10–50×) using stereomicroscopes (47%) or with the use of scanning electronic microscopy (17%). Regarding sample size, 10 specimens per group were used in 59% of the studies, while 15% used between five and eight specimens and 26% used between 11 and 25 specimens per group. The highest coefficient of variation found in each study averaged 36 ± 14% (minimum: 10%, maximum: 75%). This average is within the range mentioned in the ISO/TS 11405 as expected for these methods (20–50%).
2
A survey of recent bond strength studies
In order to verify how researchers are currently performing tensile and shear bond strength tests, 100 studies published between 2007 and 2009 (shear: 74, tensile: 26) were surveyed . Unsurprisingly, no consensus was found in any of the parameters observed.
Most studies used bonding areas between 3 and 4 mm in diameter (57%), while 4% did not include this information. These numbers do not differ from those reported 12 years ago, where the mean diameter among 50 studies was 3.97 mm and 6% of the studies omitted this information . Pre-formed rods were used in 31% of the tensile tests and none of the shear tests. Composite height varied between 2 and 5 mm. A description of the testing method was included in 66% of the studies. For shear loading, the knife-edge rod was used in half of the studies describing the experimental assembly utilized. All studies reported the crosshead speed, with 0.5 and 1.0 mm/min being the most frequent values (46 and 41%, respectively), also in agreement with a recent survey .
Specimen failure mode analysis was present in 64% of the studies, performed either under low magnification (10–50×) using stereomicroscopes (47%) or with the use of scanning electronic microscopy (17%). Regarding sample size, 10 specimens per group were used in 59% of the studies, while 15% used between five and eight specimens and 26% used between 11 and 25 specimens per group. The highest coefficient of variation found in each study averaged 36 ± 14% (minimum: 10%, maximum: 75%). This average is within the range mentioned in the ISO/TS 11405 as expected for these methods (20–50%).
3
Variables of influence related to specimen design
3.1
Bonding area
The choice of bonding area used in “macro” tensile and shear tests is often made based on the substrate area available. The ISO/TR 11405 does not identify a specific value, but it does mention a clear delimitation of the bonding area as an important requirement and shows a diagram of a split mould with a 3-mm diameter hole. The relationship between bonding area and strength has received more attention with the development of micro-tensile and micro-shear tests. For specimens with rectangular bonding areas between 0.25 and 11.65 mm 2 , tensile bond strength to dentin was shown to decrease as bonding area increased, following a logarithmic function . A similar trend was observed in enamel, with areas between 0.5 and 3.0 mm 2 . Another study showed that specimens with circular cross-section between 1.1 and 3.1 mm 2 (1.2 and 2.0 mm in diameter, respectively) presented an inverse linear relationship between bonding area and strength when tested either in tension or shear .
Such relationship between bonding area and strength is explained by fracture mechanics, initially derived from a series of experiments and mathematical deductions performed by Griffith , from which he concluded that the strength of a solid elastic body is governed by the presence of microscopic flaws. A few decades later, Irwin defined the parameters involved in crack propagation. Briefly, failure of the bonded interface occurs when a crack propagates from a critical size flaw found in an area subjected to high tensile stresses. The larger the bonding area, the higher is the probability of a flaw of critical size being present and, consequently, the lower is the specimen’s bond strength.
Very few studies evaluated the influence of bonding area on “macro” strength tests. The shear bond strength of a two-step etch-and-rinse system (Single Bond, 3M ESPE) did not vary significantly in specimens with diameters between 2 mm (3.1 mm 2 , 20 MPa) and 5 mm (19.6 mm 2 , 15 MPa), but it was statistically higher for specimens with 1 mm diameter (0.8 mm 2 , 47 MPa) and lower for those with 6 mm diameter (28.3 mm 2 , 9 MPa) . Another study testing two three-step systems under tensile loading, Scotchbond Multi-Purpose (3M ESPE) and Optibond (Kerr), showed statistically higher bond strength for 2-mm diameter specimens (10.3 MPa and 15.7 MPa, respectively) compared to 4-mm diameter (7.0 MPa and 11.1 MPa, respectively) . Though “macro” shear and tension tests show a trend for increased bond strength values with the use of small bonding areas similar to what was observed in micro-tensile specimens, the evidence in the literature is not sufficient to support a definite statement in terms of the influence of bonding areas on strength values. Therefore, the comparison among studies using different bonding areas must consider the specimen size when interpreting the results.
3.2
Elastic modulus of the resin composite
The use of stiffer composites may significantly increase bond strength values. A recent study observed a weak but statistically significant correlation between dentin shear bond strength and composite flexural properties . A similar trend was observed in an earlier study evaluating the tensile bond strength to dentin of an adhesive system associated with different composites .
The influence of composite elastic modulus on stress distribution at the bonded interface was studied by finite element analysis (FEA) using three-dimensional models representing a 3-mm diameter, 2-mm high composite cylinder ( ν = 0.25) bonded to a dentin disk ( E = 18 GPa, ν = 0.3) through a 50-μm thick adhesive layer ( E = 2 GPa, ν = 0.3). A load was applied to the composite either perpendicularly or parallel to the bonded interface in order to produce a nominal stress of 16 MPa. Due to the symmetry in geometry and loading conditions, only half of the model was represented ( Fig. 2 ). MSC.PATRAN (MSC Software Corp., Santa Ana, CA, USA) was used for pre- and post-processing, and MSC.Marc was used as processor.
In agreement with experimental data, stress concentration at the bonded interface decreased as composite modulus increased from 6 to 12 GPa, which is explained by the reduction in modulus mismatch between both materials. This effect, however, is much less pronounced for tensile loading ( Table 1 ), as previously reported . Also, the effect of composite elastic modulus on bond strength seems to be dependent upon the adhesive system used .
Composite E (GPa) | Shear loading | Tensile loading | ||
---|---|---|---|---|
σ max | τ max | σ max | τ max | |
6 | 159 | 123 | 18.5 | 7.1 |
9 | 131 | 114 | 17.8 | 6.6 |
12 | 114 | 105 | 17.3 | 6.3 |
4
Variables of influence related to test mechanics
4.1
Type of loading
Nominal bond strength values carry an underlying assumption that stresses are uniformly distributed across the bonded interface. However, as displayed in Fig. 3 , in the shear test stresses close to the loading area are much higher than the nominal shear value (16 MPa). Moreover, the choice of testing assembly has great influence on stress distribution. The use of a knife-edge chisel causes severe stress concentration at the load application area, whereas the wire loop shows a better stress distribution at the edge of the bonding area . Stress concentration with the use of the chisel may explain the small areas of composite cohesive failure close to the loading point observed experimentally . Also, it may explain the statistically lower bond strengths when the chisel is compared against testing assemblies using larger contact areas between the composite and the loading device, such as the Single-Plane Shear Test Assembly (SPSTA) , the Ultradent notched rod and the wire loop .
Another important aspect of shear tests is that closer to the interface tensile stresses are actually higher than shear, suggesting that the former is responsible for failure initiation, as previously stated . For the knife-edge chisel and the wire loop methods, shear stresses start to prevail over tension at 0.3 mm from the load application area, while for the flat rod tensile stresses predominate up to 1.2 mm ( Fig. 4 , left). Therefore, the term “shear bond strength” would more appropriately refer to the loading mode, rather than the nature of the stress responsible for bonding failure.
The distance between the point of load application and the bonded interface in shear tests also affects stress distribution. When load is applied up to 1 mm from the interface, tensile and shear stresses increase towards the bonded interface, explained by the Saint Venant principle ( i.e ., a generalized stress concentration in areas close to the load application point) . This trend for higher stress concentration with smaller distances is supported by experimental evidence showing lower bond strength when the load was applied at the interface as opposed to a 0.5 mm distance from the dentin substrate . The ratio between maximum shear and maximum principal stress decreases as load application moves away from the interface, indicative of an increase in bending moment ( Fig. 4 , right). When load is applied at distances beyond 1 mm from the interface (not shown), the increase in tensile stress as load application moves away from the bonded interface becomes even more evident .
In the tensile test, stresses are far more homogeneous across the interface than in shear and, therefore, maximum principal stress values are much closer to the nominal strength. However, the area subjected stress levels close to the maximum is much larger than in the shear test ( Fig. 5 ). The height of the composite cylinder in the tensile test does not influence stress distribution or magnitude if kept above 2 mm .
4.2
Crosshead speed
Due to the viscoelastic nature of polymers and composites, it could be expected that bond strength tests would present some strain rate sensitivity. Experimental observations revealed that composite yield strength and elastic modulus increase at higher strain rates , possibly due to secondary molecular processes. At high strain rates, the polymer chains become stiffer and molecular mobility is reduced . Determining the actual strain rate (unit: mm/mm s or s −1 ) requires an extensometer and depending upon the specimen geometry it may not be feasible. Therefore, specimen loading is usually expressed in terms of crosshead speed, in mm/min.
Studies evaluating the influence of crosshead speed on “macro” shear and tensile bond strength are few and show contradictory results. Coincidentally, two of them used the same adhesive system and composite (Single Bond and Z100, 3M ESPE). However, bonding areas, specimen storage times and test assemblies were different. One study used the Single-Plane Shear Test Assembly (SPSTA) on specimens with 5-mm diameter bonding areas, finding no statistically significant differences among crosshead speeds between 0.5 and 10 mm/min . The other study used a knife-edge steel rod to test specimens with 3-mm diameter bonding area and found statistically higher bond strengths for those loaded at 1.0 and 5.0 mm/min compared to 0.5 and 0.75 mm/min .
A similar scenario is observed with two studies that evaluated the effect of crosshead speed on tensile bond strength. Both used similar bonding areas (3.6 and 4.0 mm in diameter) and while one did not report differences among crosshead speeds ranging from 0.5 to 5.0 mm/min , the other found statistically higher bond strengths for crosshead speeds of 5.0 and 10.0 mm/min compared to 0.1, 0.5 and 1.0 mm/min .
Such inconsistencies found for both shear and tensile tests may be explained by differences among testing assemblies and/or the brittle nature of dental adhesives and composites. The comparison of crosshead speeds among testing assemblies with different compliance levels is problematic and less meaningful than comparing load rates. Also, it has been verified that at small strains, the strain rate variation had a negligible effect on the measured stress values of amorphous polymers . Nevertheless, it is important to emphasize that among the studies described, only one reported differences in bond strength within the crosshead speed range proposed in the ISO/TS 11405 (0.75 ± 0.30 mm/min).
5
Incidence of cohesive failures
As mentioned above, reporting bond strength in terms of nominal stress values is questionable due to the heterogeneous stress distribution and also due to the occurrence of cohesive failures both in the dental substrate and the resin composite. Defining categories for classification of failure modes of debonded specimens is a complicated task and, in some instances, the limit between mixed and cohesive failure becomes merely subjective. Fig. 6 shows the failure mode distribution observed in 37 studies recently published according to the bonding substrate and the loading mode . Overall, 44% of the specimens failed exclusively along the bonded interface, while 31% presented mixed failures and 25% presented predominantly ( i.e ., more than 75% of the bonding area) cohesive failure either at the composite or the dental substrate.
Rather than an indication of strong bonding, cohesive failure is explained by the mechanics of the test and the brittleness of the materials involved. In the shear test, tensile stress concentration in dentin near the crack tip causes the failure to propagate into the substrate. Versluis et al. verified both experimentally and using a failure accumulation computer model a tendency for dentin failure to increase at lower crosshead speeds, thicker adhesive layers and moving the point of load application away from the bonded interface. Dentin pull-out was also associated with high bond strength values, although the correlation for individual specimens was weak.
A recent study evaluating the tensile bond strength of five resin cements to dentin reported a strong positive correlation between strength values and the area of cohesive failure in resin observed in mixed mode failures, evaluated using scanning electron microscopy . Bonding area has also been associated with the incidence of cohesive failures. Specimens with rectangular cross-sectional areas larger than 7.17 mm 2 built using a self-etching primer system (Clearfil Liner Bond 2, Kuraray) and loaded in tension showed exclusively cohesive failures in dentin. Between 2.31 and 7.17 mm 2 , both cohesive and adhesive failures were observed .
6
A comparison between macro and micro bond strength tests
In order to verify how “macro” bond strength tests compare to their “micro” counterparts in terms of material ranking, incidence of cohesive failures and data scattering, a three-step (Adper Scothbond Multi-Purpose), a two-step etch-and-rinse (Adper Single Bond 2) and a self-etch system (Adper SE Plus), all from the same manufacturer (3M ESPE, St. Paul, MN, USA), were tested for bond strength to superficial bovine dentin. Except for the micro-tensile specimens, teeth were embedded in PVC cylinders using self-cure acrylic, with the dentin surface kept 2 mm above the embedding material. Dentin roughness was standardized using a 600-grit SiC paper. For macro shear and tensile tests ( n = 10), a 3-mm diameter area was delimited with adhesive tape. After adhesive application following manufacturers’ directions, a 3-mm height truncated cone was built using a microhybrid composite (LLis, FGM Produtos Odontológicos, Joinville, Brazil) with the smaller base (3-mm diameter) contacting the adhesive layer. Micro-shear specimens ( n = 5) were built by inserting the composite into segments of Tygon tubing (Saint-Gobain Performance Plastics, Akron, OH, USA), four per tooth, with internal diameter of 0.76 and 0.4 mm in height, and photo-activating it in contact with the adhesive-coated dentin. For the micro-tensile specimens ( n = 5), teeth crowns were sectioned perpendicularly to the exposed dentin surface and the resulting dentin area received the adhesive application. Such modification in dentin tubule orientation was necessary to increase the dentin thickness available for specimen sectioning. A 4-mm high composite block was built onto the dentin surface. All specimens were stored in distilled water at 37 °C for 24 h prior to testing. For the micro-tensile test, stick-shaped specimens 0.8–1.0 mm 2 (five per tooth) were cut using a diamond wafering blade immediately prior to testing. For macro- and micro-shear testing, a 200-μm knife-edge chisel placed in contact with the dentin surface was used to debond the composite truncated cones/cylinders. Tensile test used a clamp that contacted three-quarters of the truncated cone circumference. Articulated joints were used to assure that the bonded interface was perpendicular to the loading axis. For micro-tensile testing, Geraldeli’s jigs were used . All tests were carried out at a crosshead speed of 0.5 mm/min. Specimen failure mode was assessed under a stereomicroscope at 10–30× magnification. Results were submitted to one-way ANOVA/Tukey test at a pre-set global significance level of 5%.
Bond strength results are displayed in Fig. 7 . As discussed above, the reduction in bonding area corresponded to an increase in bond strength values. “Macro” tests displayed a similar range of values, between 5.6 and 11.5 MPa. Both shear tests ranked the adhesive systems similarly, while for the tensile tests there was an inversion between the two systems with the highest bond strengths. Coefficients of variation ranged between 28 and 36% for shear, 21 and 40% for micro-shear, 32 and 38% for tension and 25 and 64% for the micro-tensile test. The higher scattering displayed by the self-etch system in both “micro” tests may be explained by the association of lower bond strength with a more technique-sensitive specimen preparation.
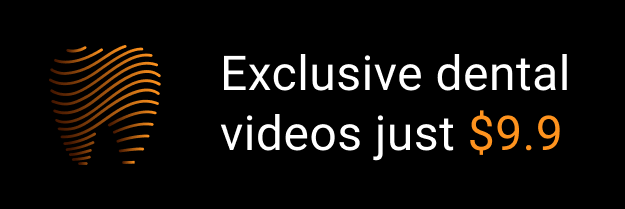