Abstract
Objective
The aim of this study was to evaluate the influence of in vitro pulpal pressure and cavity type on the tooth–composite bonding interface by means of acoustic emission (AE) analysis.
Methods
Classes I and II cavities on extracted third molars were prepared and assigned to four groups of seven teeth each: (1) direct composite restoration without simulated pulpal pressure (SPP) in class I cavity, (2) direct composite restoration with SPP in class I cavity, (3) direct composite restoration without SPP in class II cavity, (4) direct composite restoration with SPP in class II cavity. The teeth were restored with Filtek Z250 composite and Adper Scotchbond Multi-Purpose adhesive system (3M ESPE, St. Paul, MN, USA). AE events were recorded for 2000 s during light-curing. Groups 2 and 4 were subjected to 20 cm H 2 O hydrostatic pressure throughout the procedures. The data were analyzed with two-way ANOVA. After the AE test, teeth were sectioned longitudinally in mesio-distal direction, the tooth–composite interface was examined using SEM.
Results
SPP in Groups 2 (4.57 ± 1.40) and 4 (3.43 ± 1.13) yielded significantly higher AE events number than those of Groups 1 (3.43 ± 1.51) and 3 (1.71 ± 0.95) where the SPP was not applied ( p < 0.05). The number of AE events of class I cavity in Groups 1 and 2 were significantly higher than those of class II cavity in Groups 3 and 4 ( p < 0.05). SEM examination showed that all groups had intact enamel–composite interface, while micro-gaps were observed at the dentin–composite interface, mainly at the pulpal floor of the cavity. The class I cavities with SPP in Group 2 showed wider gaps more frequently than class II cavities without SPP in Group 3.
Significance
The SPP and class I cavity with high C-factor triggered more AE events, confirming its negative impact on the bonding interface.
1
Introduction
Despite the many advantages of dental composites, polymerization shrinkage, a major drawback, inevitably occurs when monomers are converted into polymers. This shrinkage, accompanied by an increase in elastic modulus as the highly cross-linked polymer network forms, causes stress to develop within the composite. When this shrinkage stress exceeds the bond strength between the tooth and composite, interfacial de-bonding occurs at the tooth–composite interface. Consequently, polymerization shrinkage stress may contribute to the failure of composite restorations due to associated problems such as microgap formation, cuspal deflection, enamel microcracking, marginal discoloration, post-operative hypersensitivity, secondary caries and pulpal inflammation .
The quality of the bonded interface between the tooth and composite can be assessed by microtensile bond strength testing , SEM imaging , dye penetration studies and confocal microscopy . However, these methods irretrievably alter the samples being tested, and only allow post evaluation of the interface between the tooth and restoration after debonding has occurred as a result of polymerization shrinkage stress. Hence, these methods are unable to provide temporal information on when and how frequently debonding occurs .
Pulpal pressure is considered to be one of the most important factors affecting bonding of composite to the tooth . Dentinal fluid driven by pulpal pressure provides excessive moisture to the exposed dentin in a prepared cavity, which in turn may be a cause of inadequate bonding at the interface. Numerous studies reported that overly wet dentin conditions are responsible for the reduction in bond strength at the tooth–composite interface . However, there is to date no published study that has analyzed the time-based distribution of the tooth–composite debonding under pulpal pressure during composite restoration.
When a material is subjected to a sufficient external force, elastic waves are generated as a result of micro-damage, cracking, or rearrangement of grain boundaries within the material. Likewise, when shrinkage stress produced by the polymerization of the composite is high enough to cause debonding at the tooth–composite interface, elastic waves would also be generated. The acoustic emission (AE) technique is capable of detecting these elastic waves in real-time using an ultrasonic sensor, offering non-destructive evaluation of the defect or microstructural breakdown. Recently, studies have been performed to evaluate the debonding behavior at the tooth–composite interface during composite restoration in real time by means of AE analysis . The studies showed very distinct debonding patterns, depending on the types of composites, adhesives, and cavities.
This study was conducted to evaluate the influence of simulated pulpal pressure (SPP) and cavity type on the tooth–composite interfacial debonding during composite restoration by analyzing AE signals. The null hypothesis of this study was that pulpal pressure or cavity type would not influence the debonding behavior at the tooth–composite interface.
2
Materials and methods
2.1
Specimen preparation
Twenty eight intact and caries-free extracted third molars were stored in 0.5% chloramine-T solution. The roots were horizontally sectioned at 3 mm below the CEJ, and the pulp was removed carefully using tissue forceps and a file. To seal the exposed dentin, the sectioned root surface was etched with 37% phosphoric acid (Etchant; 3M ESPE, St. Paul, MN, USA) for 15 s, rinsed with water for 15 s, and then blot dried. After applying the primer, the bonding resin of an etch-and-rinse, three step adhesive (Adper Scotchbond Multi-Purpose Adhesive; 3M ESPE) was then applied and light cured for 10 s.
A hole with a diameter of 1 mm was made at the center of a slide glass using a diamond bur, and then was sandblasted to roughen the surrounding glass. After etching the sandblasted surface with 4% hydrofluoric acid (Porcelain Etchant; BISCO, Schaumburg, IL, USA) for 1 min, followed by rinsing and drying, silane (Monobond S; Ivoclar-Vivadent, Schaan, Liechtenstein) was applied for 1 min. The bonding resin of the adhesive (Adper Scotchbond Multi-Purpose Adhesive; 3M ESPE) was then applied and light cured for 10 s.
A metal tube with a diameter of 0.9 mm (internal diameter of 0.7 mm) was then inserted into the pulp chamber of the tooth through the hole in the glass slide. A flowable composite (Denfil Flow, shade A2; Vericom Laboratories Ltd, Anyang, Korea) was applied around the edge of the tooth and light cured for 20 s from each side of the glass slide to fix the tooth-slide glass–metal tube together as one unit ( Fig. 1 a).
All specimens were stored in 100% humidity for 24 h before being tested, while connecting the metal tube to a polyethylene tube to a distilled water container positioned at the same level as the specimen.
The specimens were assigned to four groups of seven teeth according to the cavity type and whether the simulated pulpal pressure (SPP) was applied:
-
Group 1: Direct composite restoration without SPP in class I cavity.
-
Group 2: Direct composite restoration with SPP in class I cavity.
-
Group 3: Direct composite restoration without SPP in class II cavity.
-
Group 4: Direct composite restoration with SPP in class II cavity.
The teeth were then prepared with either class I ( n = 14; MD-length, 5 mm; BL-width, 4 mm; depth, 2 mm) or class II cavities ( n = 14; occlusal cavity: MD-length, 5 mm; BL-width, 4 mm; depth, 2 mm. Proximal box: MD-length, 1 mm; BL-width, 5 mm; depth, 3 mm) using a flat end cylindrical diamond bur, as shown in Fig. 1 b. The cavities were etched with phosphoric acid for 15 s, rinsed with water and blot dried. Primer and bonding agent (Adper Scotchbond Multi-Purpose Adhesive; 3M ESPE) was then applied to all surfaces and light cured for 10 s.
Throughout the procedures from cavity preparation, acid etching, rinsing and drying, adhesive application and composite filling to AE measurement during composite curing, all specimens were connected to the water container, and then pulpal pressure was simulated in Groups 2 and 4 by applying a hydrostatic pressure of 20 cm water, while 0 cm hydrostatic pressure was applied in Groups 1 and 3.
2.2
Acoustic emission (AE) analysis during composite curing
As in our previous study , an AE sensor (M204A, Rectuson, Sungnam, Korea) was attached with grease to the slide glass 1 cm away from the tooth ( Fig. 1 a). After the cavity was filled with composite (Filtek Z250, shade A2, 3M ESPE) in bulk and a 20 s baseline reading was obtained. Then the composite was light cured from the occlusal surface for 40 s at 750 mW/cm 2 (Elipar S10 LED Curing Light; 3M ESPE). Subsequent to light curing, the tooth was covered with wet gauze to prevent cracking from dehydration. AE signals generated as a result of debonding at the tooth–composite interface were recorded for 2000 s after the initiation of light curing. The signals from the AE sensor were amplified (A1002, Rectuson, Sungnam, Korea) (2500×) and stored on a computer using a data acquisition board (USB-6361, National Instrument, Austin, TX, USA). The measurement parameters were: 2 MHz sampling rate, 2 ms duration and 70 mV threshold. AE data were analyzed with AE parameters such as event number, event time and amplitude.
2.3
SEM examination of the tooth–composite interface
After the AE test, teeth were sectioned longitudinally in a mesio-distal direction using a low-speed diamond saw (Isomet, Buehler, Lake Bluff, IL, USA) to produce a 1 mm thick specimen. The sectioned surfaces were polished on a 1200-grit paper and acid-etched for 15 s to remove the smear layer. Vinyl polysiloxane impressions of the sectioned surfaces were made and epoxy replicas poured in order to examine the tooth–composite interface with a SEM (S-4000, Hitachi, Tokyo, Japan).
2.4
Statistical analysis
The data were analyzed using two-way ANOVA ( α < 0.05) with SPSS software to investigate the effect of SPP and cavity type on the number of AE events.
2
Materials and methods
2.1
Specimen preparation
Twenty eight intact and caries-free extracted third molars were stored in 0.5% chloramine-T solution. The roots were horizontally sectioned at 3 mm below the CEJ, and the pulp was removed carefully using tissue forceps and a file. To seal the exposed dentin, the sectioned root surface was etched with 37% phosphoric acid (Etchant; 3M ESPE, St. Paul, MN, USA) for 15 s, rinsed with water for 15 s, and then blot dried. After applying the primer, the bonding resin of an etch-and-rinse, three step adhesive (Adper Scotchbond Multi-Purpose Adhesive; 3M ESPE) was then applied and light cured for 10 s.
A hole with a diameter of 1 mm was made at the center of a slide glass using a diamond bur, and then was sandblasted to roughen the surrounding glass. After etching the sandblasted surface with 4% hydrofluoric acid (Porcelain Etchant; BISCO, Schaumburg, IL, USA) for 1 min, followed by rinsing and drying, silane (Monobond S; Ivoclar-Vivadent, Schaan, Liechtenstein) was applied for 1 min. The bonding resin of the adhesive (Adper Scotchbond Multi-Purpose Adhesive; 3M ESPE) was then applied and light cured for 10 s.
A metal tube with a diameter of 0.9 mm (internal diameter of 0.7 mm) was then inserted into the pulp chamber of the tooth through the hole in the glass slide. A flowable composite (Denfil Flow, shade A2; Vericom Laboratories Ltd, Anyang, Korea) was applied around the edge of the tooth and light cured for 20 s from each side of the glass slide to fix the tooth-slide glass–metal tube together as one unit ( Fig. 1 a).
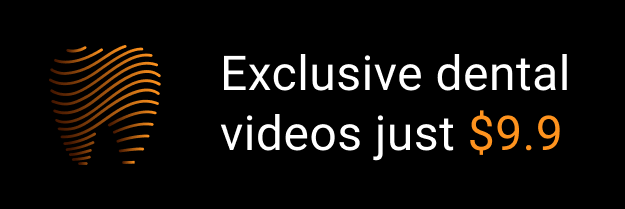