Introduction
A protocol was introduced to achieve accurate bracket placement in vivo, which consisted of operative procedures for precise control, and a computer-aided design and computer-aided manufacturing–guided bonding device. To evaluate the accuracy of this protocol, a 3-dimensional assessment was performed.
Methods
Ten consecutive patients were enrolled. Strictly following the protocol, from December 2017 to March 2018, brackets were placed on the teeth of each patient using the device. To evaluate the accuracy, deviations of positions and orientations for bracket placement were measured. Each patient was followed up after 3 months regarding bracket failures.
Results
The guided bonding device was used in all cases, and a total of 205 brackets were successfully bonded and evaluated. Except for 15.12% brackets with torque deviation over 2°, the deviations in mesiodistal, buccolingual, vertical, rotation, and angulation were below the clinical acceptable range (0.5 mm in translation or 2° in orientation) for all brackets. In the 3-month follow-up, there was no bracket failure in any patient.
Conclusion
This protocol transferred the planned bracket position from the digital setup to patient’s dentition with generally high positional accuracy.
Highlights
- •
This protocol was effective for accurate bracket placement in vivo.
- •
The protocol provided a simple way to assess the fitness between the device and the dentition.
- •
The protocol provided visibility to assess the bracket position and access to adjust the bracket placement intraoperatively.
- •
The protocol used direct bonding, which benefited bonding strength.
- •
Three features eliminated undercut and assisted in removal of the device after bonding.
Effective and efficient orthodontic treatment is based on the concept that ideal bracket placement will correct tooth positions in all 3 spatial planes. Orthodontists have striven to improve the accuracy of the bracket bonding for years. Since the introduction, in 1972, by Silverman et al, of transfer trays of indirect bonding, technique has improved with regard to design and fabrication; for example, polyvinyl siloxane trays, vacuum-formed trays, combination of polyvinyl siloxane and vacuum-formed trays, 3-dimensional (3D) printed trays, and transfer jigs. Among these approaches, there are 3 major common steps in the fabrication of a traditional transfer tray: bonding brackets on a stone model, forming the transfer tray, and then removing the transfer tray with brackets from the stone model. , In this lengthy process, much experience and skillful operation are required by the technician to reduce errors in each step of the process. The high sensitivity of the technique makes it difficult to popularize in clinical practice.
Intraoperatively, there are 2 critical steps needed to succeed in indirect bonding. First, seat the transfer tray completely on the patient’s dentition. Second, assess and control the bracket position intraoperatively. However, the elastic property of most transfer trays makes it difficult to differentiate among incomplete seating, complete seating, and over seating of the transfer tray for each single tooth when individualized finger pressure is applied. Moreover, because the brackets are partially or completely covered by the transfer tray, there is a lack of visibility, making it difficult to tell whether the bracket is in the predetermined position, as well as limiting access to adjust the bracket position or remove the excess adhesive immediately. Thus, transferring the bracket position to the patient’s dentition with accuracy is challenging for orthodontists with limited experience, especially on dentitions with various malocclusion and intraoral complications. To overcome the above drawbacks, development of a simple and efficient way of indirect bonding with accuracy is warranted, and precise intraoperative control is equally important to achieve accurate bracket placement based on a well-fabricated tray.
In this prospective study, a protocol was introduced to achieve accurate bracket placement. This protocol consisted of operative procedures for precise control, and a computer-aided design and computer-aided manufacturing–guided bonding device. In addition, a 3D assessment was performed to evaluate the accuracy of bracket placement.
Material and methods
Ten consecutive orthodontic patients (6 women and 4 men) were enrolled. All subjects had permanent dentitions and full clinical crown heights were available. Subjects with the following indications were excluded: (1) teeth that were not planned for bracket bonding at the start of treatment, (2) teeth with defects that could affect bracket placement, and (3) teeth with inadequate space that limited bracket placement owing to the initial malocclusion. The bracket placement was performed in each patient by the same orthodontist using the guided bonding device from December 2017 to March 2018 in West China Hospital of Stomatology, Sichuan University, Chengdu, China. The Helsinki Declaration was followed during the performance of this study. The project was under the approval of the local institutional ethical committee and every participant signed an informed consent form.
Digitized dentition of each patient was acquired using an intraoral scanner (3Shape TRIOS, Copenhagen, Denmark). Using a 3Shape OrthoAnalyzer 2015, preadjusted edgewise brackets with 0.022-inch slot (Clarity Adhesive Coated Advanced Brackets, 3M Unitek, Monrovia, Calif) were virtually bonded on incisors, canines, and premolars, and buccal tubes (Shinye Orthodontic Products, Hangzhou, China) were placed on the first molars. Then, the digitized models with brackets, also termed simulative dentitions, were exported in stereolithography format.
Using the simulated dentitions, the guided bonding device was designed in Freeform software (version 12.0; Geomagic, Morrisville, NC). It consisted of 3 parts: (1) an L-shaped guides that fit the occlusal and distal edge of the tie-wings on the bracket, (2) a splint that covers the occlusal surface completely or partially, and (3) connecting rods that join the other 2 parts ( Fig 1 ). The device was then fabricated using a 3D printer.

Precise control of bracket placement
Fitness between the device and the stone model: The guided bonding device was placed on the stone model. Position deviation and fitness were compared from the buccal and lingual sides against the virtual design on the computer. If any deviation, misfit, or space was detected, the orthodontist would grind the region in contact with deep grooves or walls between neighboring teeth. If the misfit was still detected, the orthodontist would forgo the guiding device and refer to traditional direct bonding ( Fig 2 ).

Fitness between the device and the dentition: After the dentitions were isolated using a plastic mouth opener with a cheek retractor, the teeth surfaces were cleaned with 95% alcohol. The buccal surfaces were then etched with 37% phosphoric acid (Eco-Etch, Ivoclar Vivadent, Schaan, Liechtenstein) for 20 seconds, rinsed with water for 30 seconds to ensure complete removal of the etchant, and air-dried until they appeared dull and frosty.
Subesequently, the sterilized-guided bonding device was seated over the upper dentition. Position deviation and fitness were visually checked again. If no deviation, misfit, or space was detected, the orthodontist would put gauze balls in the lingual and occlusal sides of the device. Then, the patient would be asked to bite through the gauze balls to fix the device on the dentition. If not, the orthodontist would forgo the guiding device and refer to traditional direct bonding.
Control of bracket placement in 3 directions: After the primer (Transbond Moisture Insensitive Primer, 3M Unitek, Monrovia, Calif) was applied to the teeth using a brush, the bracket with adhesive was placed on the tooth surface using the forceps by pressing as with direct bonding, and excessive adhesive around the bracket was removed. This process ensures the buccolingual position of the bracket. The position of the bracket was then adjusted to fit the L-shaped guide, ensuring the mesiodistal and vertical positions of the bracket. After all brackets were correctly placed, the light-curing lamp was used on each tooth.
Removal of the device: The gauze balls were removed first. As the undercut was eliminated because (1) the splint only had contact with the occlusal surface of the dentition, (2) the L-shaped guides only had contact with the distal and occlusal sides of the bracket, and (3) a certain distance existed between the L-shaped guides and the tooth surface, the device could be directly pried away from the dentition with a probe.
For the lower dentition, the same operation (steps 2 to 4) was performed. Each patient was followed up after 3 months with regard to failure of the brackets.
Assessment of accuracy for bracket placement
After bracket bonding, the dentitions with brackets, termed postoperative dentitions, were dried and made antireflective using a scanning preparation spray (Cerec Optispray, Sirona Dental Systems). Then, they were scanned and digitized using an intraoral scanner (3Shape TRIOS). By selecting the same region (teeth, except for the buccal surface with brackets), each tooth on the simulative dentitions was registered to the postoperative dentitions in Geomagic Studio 2013 (version 2013; Geomagic, Morrisville, NC).
Each bracket of the simulative dentitions was constructed with a local coordinate system, termed a simulative bracket ( Fig 3 , A ). By selecting the same region on the simulative bracket and postoperative bracket, the simulative bracket with the local coordinate system was registered to the position of postoperative bracket. Then, the postoperative bracket was represented by the duplication of the simulative bracket (colored in red) with a local coordinate system (colored in yellow) in the position of the postoperative bracket. By comparing the position of the 2 local coordinate systems (colored in blue and yellow), the position deviations in mesiodistal, buccolingual, and vertical orientations, and the orientation deviations in torque, angulation, and rotation were automatically computed.

Statistic analysis
Statistical analyses were performed using SPSS 16.0 (SPSS Inc, Chicago, Ill) and MedCalc 15.0 (MedCalc Software, Mariakerke, Belgium). To evaluate the reproducibility of measuring deviations of positions (mesiodistal, vertical, and buccolingual) and deviations of orientations (torque, angulation, and rotation), 20 brackets were randomly selected. And the measurements for each bracket were performed by the same investigator after an interval of 2 weeks. Bland-Altman plots were used to assess the quality of reproducibility.
For the assessment of accuracy for bracket placement, mean position deviations (mesiodistal, buccolingual, and vertical) (n = 615, 205 brackets), and mean orientation deviations (torque, angulation, and rotation) of brackets between the simulative and postoperative groups are presented (n = 615, 205 brackets). Shapiro-Wilk test showed that the variables were not normally distributed. To compare the deviations above with a clinical acceptable error (0.5 mm in translation or 2° in orientation), Wilcoxon matched-pairs signed rank test was applied for non-normally distributed variables. A level of á = 0.05 was set for significance.
In addition, the directionality and frequency of error resulting from the bracket transfer during guided bonding were calculated for each tooth type (incisors, canines, premolars, and molars) and the complete dataset.
The sample size was determined according to previous studies. , With an observed sample size of n = 205, a power analysis for Wilcoxon matched-pairs signed rank test (2-tailed) conducted a posteriori using G*Power 3.1.9.3 indicated 100% power to detect a small effect size (Cohen’s d = 0.4) at a significance level of 0.05.
Results
In total, 205 brackets (from 18 dentitions, 10 patients) were bonded using the device. Before bonding, misfit was found in the region of crowded anterior teeth in 2 cases. After grinding the walls between neighboring teeth, all devices fit the stone model well. Intraoperatively, no misfit was found regarding the placement of the guided bonding device on the dentitions. All bracket placements were guided by the L-shaped guides. No difficulty was found in removal of the device in any case.
The guided bonding device provided accurate bracket placement. The reproducibility of the measurement was verified. The error of measuring position deviation ranged from −0.010 to 0.010 mm, with a mean (standard deviation [SD]) of −0.001 (0.004) mm. The error of measuring orientation deviation ranged from −0.30° to 0.28°, with a mean (SD) of −0.012° (0.109°). In the Bland-Altman plots, most values ranged within a mean of ± 1.96 (SD), which confirmed the reproducibility of measuring position deviations and orientation deviations ( Fig 4 ).

The position and orientation deviations between simulative and postoperative groups were presented in Table I . The position deviations of brackets in the in mesiodistal, vertical, and buccolingual orientations were much less than 0.5 mm for all brackets, with a mean value (SD) of 0.009 (0.091) mm ( P <0.001), 0.060 (0.131) mm ( P <0.001), and −0.087 (0.047) mm ( P <0.001), respectively. The orientation deviations in torque, angulation, and rotation of bracket were much less than 2° for all brackets, with a mean value of 0.286° (1.276°) ( P <0.001), 0.061° (0.806°) ( P <0.001), and −0.015° (0.903°) ( P <0.001), respectively.
Tooth type | n ∗ | Dimension | |||||||||||
---|---|---|---|---|---|---|---|---|---|---|---|---|---|
Mesiodistal (mm) | Buccolingual (mm) | Vertical (mm) | Torque (°) | Angulation (°) | Rotation (°) | ||||||||
Mean | SD | Mean | SD | Mean | SD | Mean | SD | Mean | SD | Mean | SD | ||
Incisor | 71 | 0.013 † | 0.079 | 0.123 | 0.064 | −0.088 | 0.041 | −0.371 | 0.742 | 0.061 | 0.660 | 0.014 | 0.821 |
Canine | 36 | 0.016 | 0.097 | 0.033 | 0.126 | −0.083 | 0.041 | 0.343 | 1.152 | 0.087 | 0.912 | −0.007 | 0.909 |
Premolar | 62 | 0.001 | 0.099 | −0.031 | 0.152 | −0.089 | 0.049 | 0.794 | 1.359 | 0.078 | 0.823 | −0.064 | 0.986 |
Molar | 36 | 0.007 | 0.094 | 0.125 | 0.087 | −0.091 | 0.054 | 0.690 | 1.473 | 0.060 | 0.900 | 0.047 | 0.906 |
Total | 205 | 0.009 | 0.091 | 0.060 | 0.131 | −0.087 | 0.047 | 0.286 | 1.276 | 0.061 | 0.806 | −0.015 | 0.903 |
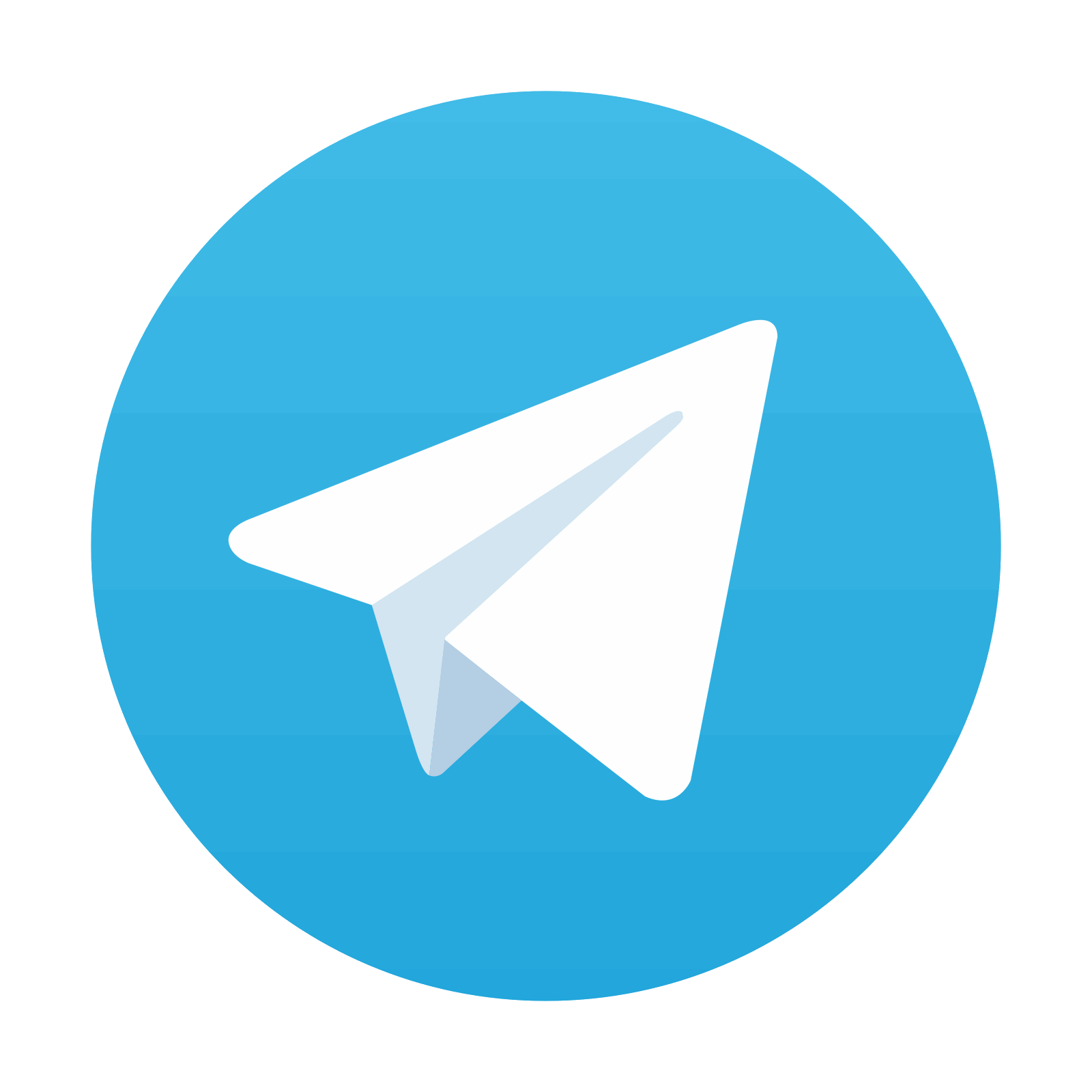
Stay updated, free dental videos. Join our Telegram channel

VIDEdental - Online dental courses
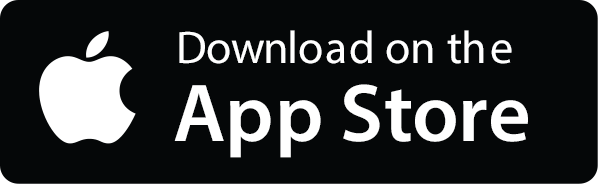
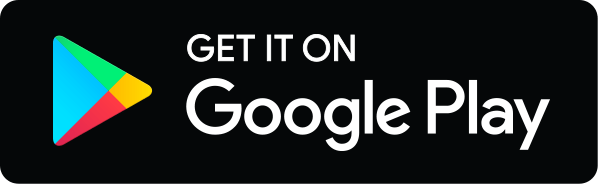
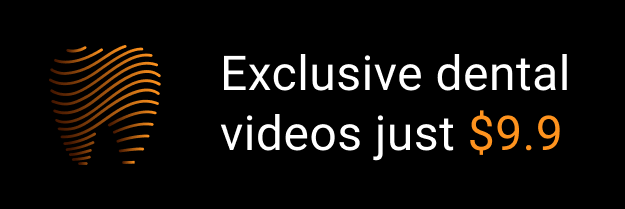