Introduction
This study aimed to investigate whether a digital light processing (DLP) printer could perform efficiently and with adequate accuracy for clinical applications when used with different settings and variations in the orientation of models on the build plate.
Methods
Digital impressions of the oral environment were collected from 15 patients. Subsequently, digital impressions were used to make 3-dimensional printed models using the DLP printing technique. Three variables of the printing technique were tested: placement on the build plate (middle vs corner), thickness in the z-axis (50 microns vs 100 microns), and hollow vs solid shell. After being printed with different printing techniques and orientations on the same printer, a total of 240 maxillary and mandibular arches were measured. These variables generated 8 printing combinations. Tooth and arch measurements on each model type were compared with each other. Intraobserver reliability of the repeated measurement error was assessed using intraclass correlation coefficient.
Results
All mean differences among the printing variations were statistically insignificant. The Bland-Altman plots verified a high degree of agreement among all model sets and printing variations. In addition, the measurements were highly reproducible; this was demonstrated by the high intraclass correlation coefficient for all measurements recorded.
Conclusions
The DLP printer produced clinically acceptable models in all areas of the build plate, with hollow and solid model shells, and at its high-speed setting of 100 microns. The applications of the DLP printer tested should be a viable option for printing in a clinical environment at a high-speed setting while filling the build plate and printing with less resin.
Highlights
- •
The most efficient utilization of a digital light processing printer for clinical applications was tested.
- •
Different settings and variations in the orientation of models on the build plate were tested.
- •
Variables were placement on the build plate, thickness in the z-axis, and hollow vs solid model design.
- •
The digital light processing printer produced clinically acceptable models for all variables tested.
Success in orthodontics can be achieved with proper diagnosis and treatment planning. Diagnosis is primarily based upon factors taken from dental models, including spacing, crowding, tooth size, arch form, and dimensions, along with tooth-arch discrepancies. This success can be limited by the accuracy of the records. Although the majority of record-keeping has changed from paper to digital records, the transition from plaster casts to digital impressions and printed models is not as widespread. As the necessity for evidence-based dentistry increases, new forms of records and models need to be analyzed for accuracy and reproducibility in order for clinical application to be justified.
Many studies have been performed to compare the accuracy of digital dental models acquired from different methods of extraoral scanning (ie, desktop scanners for scanning models or impressions). , However, only a few studies have examined the accuracy and reproducibility of dental models produced from different 3-dimensional (3D) printing techniques and styles of printing. Previous studies have had limited sample populations and have left out dimensional measurements of the dental arches. , Only 1 study has evaluated the accuracy of the JUELL 3D printer (Park Dental Research Corporation, New York, NY) in comparison with stone models and a polyjet 3D printer at the most accurate printing settings and reported no clinical differences. To date, there have been no studies conducted to evaluate the accuracy of different printing techniques and orientations with a digital light processing (DLP) printer. In general, the DLP technique is accepted to be more efficient and faster than the stereolithography technique, which is also commonly used for in-office printing. This is possible, because it uses an arc lamp, liquid crystal display panel, or projection source to cure an entire layer of resin in the x-y axis at one time instead of laser tracing the image.
With the advent of 3D printing technology being relatively new, there is limited literature on the accuracy of these printed models. , , , Current research has shown clinically acceptable models with high accuracy for these printers. Most of the studies performed on 3D printing of orthodontic models have had some limitations. All except 3 of the previous studies have excluded arch dimension measurements. , , If 3D printers are to find a place in a practitioner’s daily workflow, the accuracy of the arch dimension measurements needs to be evaluated, which is crucial if the printers are used for fabrication of orthodontic appliances. In addition, except for the studies by Hazeveld et al and Brown et al , previous studies have had small sample sizes of 10 patients or less. , , , , ,
The advent of 3D printing is changing the way dentistry and medicine are practiced. If a 3D printer is to be clinically acceptable in the health care field, its accuracy, precision, and reproducibility need to be thoroughly tested. The transition to a 3D model alternative will enable the daily office flow to become more efficient and will appeal to patients as up-to-date dental care. If the DLP printer can perform with clinically acceptable accuracy at different settings and model orientations, a more efficient and economical approach may be possible. It was hypothesized that the DLP printer would print 3D models with reproducible and consistent accuracy while filling the build plate, printing at a high-speed setting, and using less resin via printing with hollow models.
Materials and methods
Ethical approval for this study was obtained by the University of Oklahoma Institutional Review Board for the Protection of Human Subjects (no. 8366) on November 27, 2017.
A power analysis was done based on similar precursor studies. , A total of 15 patients, or 30 arches, were required for this study in order to attain an 80% probability of generating a 95% confidence interval with a margin of error 0.15 mm for tooth measurements and 0.5 mm for arch dimension measurements.
The data collection protocol followed the guidelines outlined by Brown et al. The printing of additional models also followed the protocol used by Brown et al. Patients were randomly selected from a pool of retention patients who received orthodontic treatment at the University of Oklahoma Graduate Orthodontic Clinic. A patient was considered in retention if his/her orthodontic appliances were removed six-18 months before collection of records for this study. All patients had previously completed comprehensive orthodontic treatment with all permanent teeth present first molar to the first molar in both dental arches.
Digital impressions were taken at the 1-year retention appointment for all patients between December 2016 and February 2017. Digital impressions were recorded using a single CEREC Omnicam intraoral scanner (Dentsply Sirona, York, Pa). All aspects of record collection followed the manufacturer’s recommendations.
All digital models generated with the intraoral scanner were converted to stereolithography (STL) file format using additional 3D Imaging Software (Dolphin Imaging & Management Solutions, Chatsworth, Calif). The STL files were cleaned and prepared for 3D printing using Netfabb additive manufacturing and design software (Autodesk, San Rafael, Calif). The prepared STL file for each patient was printed using a DLP technique (JUELL 3D Flash OC; Park Dental Research Corporation, New York, NY). All models were printed in a horizontal orientation with a horseshoe-shaped base.
The study models were printed using variations in printing techniques and different orientations. These variations included, (1) a model set in the middle (M) of the plate vs a maxillary model in all 4 corners (C) on the plate; (2) solid (S) vs hollow (H) shelled models; and (3) models printed at 50 (50) and 100 (100) microns in the z-axis. The combination of these variables allowed for a total of 8 different printing combinations (100 SC, 100 HC, 100 SM, 100 HM, 50 SM, 50 HM, 50 SC, 50 HC). Each of the 30 models was printed using these printing combinations.
A total of 120 maxillary and 120 mandibular arches were measured. These were 30 total arches, with each being printed using the 8 printing combinations types. All measurements of the physical models were obtained using a calibrated digital caliper (Orthopli, Philadelphia, Pa), and the teeth measured to the nearest 0.01 mm directly from the models. However, the arch measurements were measured using the digital caliper from a 1:1 scale photocopy of the occlusal views. For all teeth, first molar to the first molar in both dental arches, tooth measurements recorded included: (1) the mesial-distal widths from point contact to point contact (clinical crown width) and (2) the incisal-gingival heights from gingival zenith to cusp tip or incisal edge (clinical crown height). Incisal-gingival measures were used to describe the clinical crown heights for both anterior and posterior teeth. Arch dimension measurements for both arches included: (1) intercanine width from cusp tip to cusp tip, (2) intermolar width from mesiolingual cusp tip to mesiolingual cusp tip, and (3) arch depth from the midline of the central incisors to a perpendicular line crossing through the mesial contacts of the first molars ( Fig ). Three calibrated researchers (LV, BF, and SS) measured and recorded the data. A singular examiner was assigned to gather all data for each of the respective measurements for consistency of landmark identification. One researcher (LV) measured mesial-distal, 1 researcher (BF) measured incisal-gingival, and 1 researcher (SS) measured arch dimensions for all models.

Statistical analysis
Bland-Altman plots were used to evaluate the agreement of the mesial-distal, incisal-gingival, intercanine, intermolar, and arch depth measurements among the different 3D printed models. These comparisons included mean differences as well as limits of agreement for the 4 model types. A total of 20% of each model type was measured a second time with a 2-week interval between measurements. The intraclass correlation coefficient was used to assess the intraobserver reliability of the repeated measurement error.
Results
A total of 6720 tooth measurements were recorded from the 240 maxillary and mandibular arches along with an additional 720 arch dimension measurements for a final total of 7440. Measurements on the printed model combinations were found to be highly reproducible with a high intraclass correlation coefficient for all measurements based on the repeated measurement error ( Table I ). Only the arch depth of the 40 repeated arch measurements for the 50 HC models was slightly larger.
Model ∗ | Mesial-distal | Incisal-gingival | Intercanine | Intermolar | Arch depth |
---|---|---|---|---|---|
100 HC | 0.04 | 0.02 | 0.07 | 0.08 | 0.11 |
100 HM | 0.09 | 0.01 | 0.04 | 0.05 | 0.04 |
100 SC | 0.08 | 0.01 | 0.05 | 0.05 | 0.04 |
100 SM | 0.08 | 0.02 | 0.03 | 0.09 | 0.06 |
50 HC | 0.06 | 0.02 | 0.06 | 0.06 | 0.22 |
50 HM | 0.06 | 0.02 | 0.08 | 0.08 | 0.05 |
50 SC | 0.08 | 0.02 | 0.06 | 0.04 | 0.18 |
50 SM | 0.06 | 0.02 | 0.08 | 0.05 | 0.04 |
∗ The repeated measurement error equals 1 minus the intraclass correlation coefficient.
High agreement between all model sets in the mesial-distal, incisal-gingival, intercanine, intermolar, and arch depth measurements was found among all the model sets ( Tables II-IV ). The limits of agreement and the P values for the mean differences vs 0 are also presented in these tables. The mean differences for all model comparisons did not significantly differ from 0. However, mean differences for the intercanine widths, although not statistically significant, were on the higher side of clinically acceptable for the 50 SM vs 50 SC, 100 SM vs 100 SC, and 100 HM vs 100 HC. The arch depth was also on the higher side of clinically acceptable, although not statistically significant, for comparison of the 50 SM vs 50 SC and the 100 HM vs 100 HC. Finally, the intermolar widths were on the higher side for the 100 HM vs 100 HC and the 100 HC vs 100 SC as well as 100 HC vs 50 HC, and the 100 HM vs 50 HM.
Measurement | Mean differences (mm) | Limits of agreement (mm) | P |
---|---|---|---|
Agreement between (1) 50 SM and (2) 50 SC | |||
Mesial-distal | 0.002 | –0.15 to 0.15 | 0.972 |
Incisal-gingival | –0.002 | –0.06 to 0.06 | 0.975 |
Intercanine | –0.21 | –0.61 to 0.02 | 0.607 |
Intermolar | 0.04 | –1.2 to 1.29 | 0.958 |
Arch depth | –0.22 | –0.77 to 0.33 | 0.609 |
Agreement between (1) 100 SM and (2) 100 SC | |||
Mesial-distal | –0.02 | –0.02 to 0.16 | 0.755 |
Incisal-gingival | 0 | –0.06 to 0.06 | 0.994 |
Intercanine | –0.35 | –0.84 to 0.14 | 0.381 |
Intermolar | –0.07 | –0.96 to 0.83 | 0.932 |
Arch depth | –0.14 | –1.15 to 0.87 | 0.748 |
Agreement between (1) 50 HM and (2) 50 HC | |||
Mesial-distal | –0.004 | –0.17 to 0.16 | 0.951 |
Incisal-gingival | –0.004 | –0.13 to 0.12 | 0.947 |
Intercanine | –0.13 | –0.52 to 0.26 | 0.74 |
Intermolar | –0.19 | –1.46 to 1.09 | 0.813 |
Arch depth | –0.08 | –1.08 to 0.93 | 0.851 |
Agreement between (1) 100 HM and (2) 100 HC | |||
Mesial-distal | –0.001 | –0.07 to 0.69 | 0.984 |
Incisal-gingival | 0.00 | –0.05 to 0.05 | 0.998 |
Intercanine | –0.29 | –0.72 to 0.13 | 0.465 |
Intermolar | –0.26 | –1 to 0.48 | 0.728 |
Arch depth | –0.29 | –1.33 to 0.75 | 0.498 |
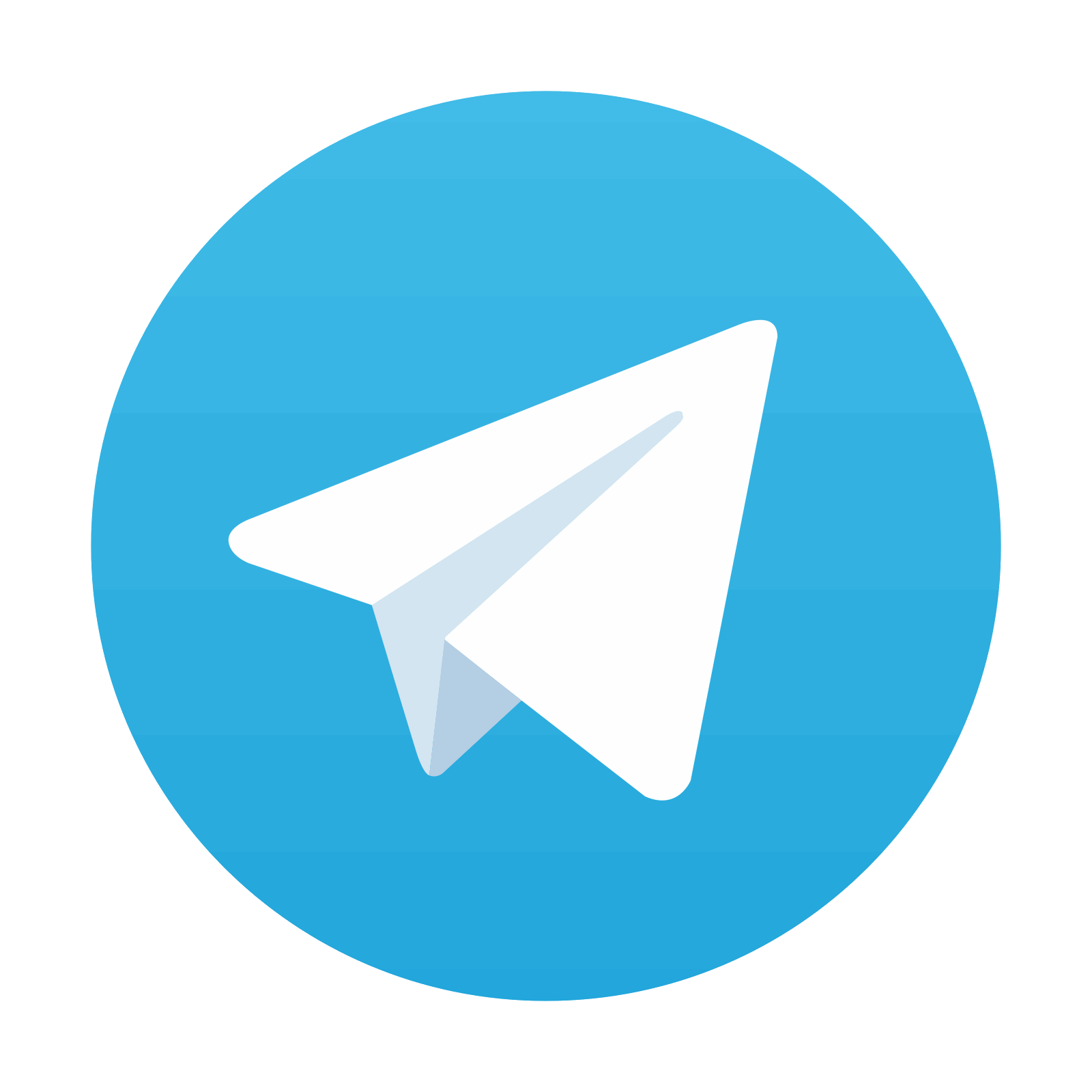
Stay updated, free dental videos. Join our Telegram channel

VIDEdental - Online dental courses
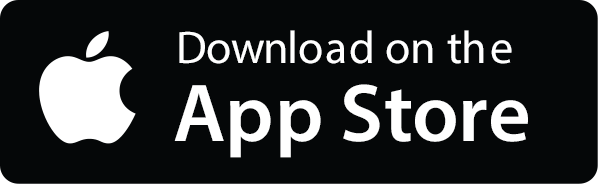
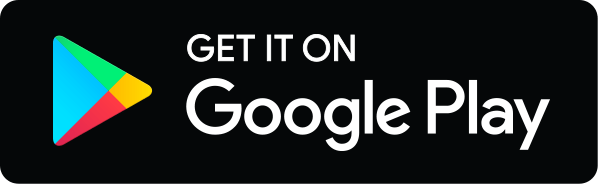
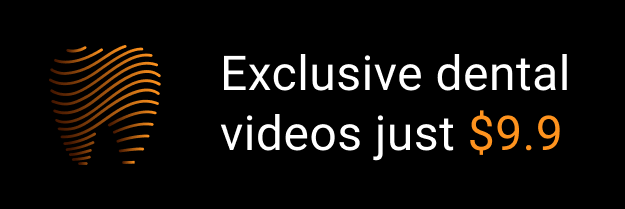