Abstract
With rapid advances in modern dentistry, high-energy output instruments ( e.g. , dental lasers and light polymerizing units) are increasingly employed in dental surgery for applications such as laser assisted tooth ablation, bleaching, hypersensitivity treatment and polymerization of dental restorative materials. Extreme high temperature occurs within the tooth during these treatments, which may induce tooth thermal pain (TTP) sensation. Despite the wide application of these dental treatments, the underlying mechanisms are far from clear. Therefore, there is an urgent need to better understand heat transfer (HT) process in tooth, thermally induced damage of tooth, and the corresponding TTP. This will enhance the design and optimization of clinical treatment strategies. This paper presents the state-of-the-art of the current understanding on HT in tooth, with both experimental study and mathematical modeling reviewed. Limitations of the current experimental and mathematical methodologies are discussed and potential solutions are suggested. Interpretation of TTP in terms of thermally stimulated dentinal fluid flow is also discussed.
1
Background
Heat transfer (HT) in human tooth is a common process in both daily life and clinical dentistry . With advances in modern dentistry, instruments with high-energy output ( e.g. , dental lasers , light polymerizing units (LPUs) and high-speed hand-pieces (HSHPs) ) have been widely employed in dental treatments. Typical clinical examples of these treatments are laser assisted tooth ablation , caries detection and prevention , bleaching and hypersensitivity treatment . Table 1 lists the currently available therapies and the corresponding intrapulpal temperature rise (IPTR).
Temperature rise (°C) | References | |
---|---|---|
Laser assisted tooth ablation | 2.3–24.7 | |
Laser assisted caries prevention | 1.2–4.0 | |
Bleaching (without light/laser assisted) | 0.1–1.1 | |
Bleaching (with light/laser assisted) | 1.1–16.0 | |
Polymerization of dental restorative materials (DRMs) | 2.9–7.8 | |
HSHPs cavity preparation (without water, high load) | 16.4–19.7 | |
HSHPs cavity preparation (without water, low load) | 7.1–9.5 | |
HSHPs cavity preparation (with water, high load) | 2.2–5.9 | |
10.0–11.7 | ||
HSHPs cavity preparation (with water, low load) | −1.8 (drop in temperature) to 5.0 |
Heat generated within the tooth during the above-mentioned treatments can cause thermally induced damage of tooth “hard” components (enamel and dentine) and “soft” components (dental pulp) . Temperature rise may also induce tooth thermal pain (TTP) . The lack of detailed knowledge concerning these tooth thermal behaviors has hindered the further design and optimization of treatment strategies for clinical applications. Therefore, there is an urgent need to investigate the thermal behavior of human teeth.
1.1
Structure of human tooth
Human tooth is a hard tissue with layered composite structure ( e.g. , enamel, dentine, cementum and dental pulp layers ) of complex geometry. Enamel is a highly mineralized layer consisting of 96% mineral, with water and organic material accounting for the remaining 4%. Dentine is a mineralized connective tissue layer with an organic matrix of collagenous proteins , composed of ∼70% inorganic materials, ∼20% organic materials, and ∼10% water by weight. There are dentinal tubules (DTs) radiating from the pulp cavity to the exterior cementum or dentine–enamel junction (DEJ) ; see Fig. 1 .

Human tooth is also a sensory tissue. The pulp is a soft connective tissue containing nerve fibers with diameter varying from 1 to 10 μm . There are also sensory nerve endings in DTs , which penetrate approximately 100–150 μm into the tubules from the wall of pulp chamber . These pulpal nerve terminals play an important role in sensing thermal stimulus .
1.2
What induces HT in tooth?
HT in tooth occurs in both daily life and dental surgery. The thermal environment of teeth during daily life varies over a wide range of temperatures (−5 to 76.3 °C) , Table 2 .
Temperature (°C) | Hot beverage | Hot food |
---|---|---|
Highest a | 76.3 | 53.6 |
Mean maximum b | 46.4 | 41.6 |
Calculated extreme c | 61.4 | 50.2 |
a Highest temperature measured in one volunteer between the lower incisors.
b Mean maximum temperature ± standard deviation recorded by each electrode for all volunteers.
c Calculated extreme temperature obtained by adding two standard deviations to the mean maximum temperature measured in vivo .
Since its first introduction as a tool for patient care in 1964 , laser has been found to be an exciting and rewarding technology in dental surgery ( e.g. , dental ablation , cosmetic tasks , dental caries prevention and hypersensitivity treatment ) due to advantages such as directivity, pulsed mode ability, and monochromaticity . However, there is a major concern with the overheating of enamel and dentine during tooth-laser interaction, which causes carbonization, melting and cracking of the enamel and dentine, as well as inflammation and necrosis of the pulp .
Dental restorative processes are used to decrease the polymerization periods of resin composite and minimize its polymerization shrinkage. During these processes, teeth are exposed to the radiation of LUPs ( e.g. , halogen polymerization lamps or semiconductor diode light) and experience substantial temperature increase of 10–18 °C within the resin and adjacent tooth , 7.5–29 °C at DEJ , and 2–9 °C at pulpal chamber wall .
Treatments with HSHPs are performed in dental clinics for applications such as cavity preparation and deceased tissue removal. Heat is generated by friction between the machinery ( e.g. , driller) and tooth, resulting in IPTR . This temperature rise depends on the pressure and speed of the hand-pieces as well as the cooling methods used .
1.3
Specialties of tooth thermal behavior
Unlike HT in engineering materials, the thermal behavior of tooth is mainly a heat conduction process coupled with tooth physiological processes ( e.g. , dentinal fluid flow (DFF) , pulpal blood flow (PBF) ). The thermophysical properties (TPs) of teeth vary between different layers ( e.g. , enamel and dentine) and depend on their microstructures. For instance, the thermal conductivity (TC) of human dentine decreases with increasing volume fraction of dentine tubules . The flow of dentinal fluid in the dentine tubules upon heating (or cooling) can also enhance HT within the pulp. In addition, PBF rate increases when the intrapupal temperature rises above 42 °C and decreases during cooling . The perfused blood plays an important role in the thermoregulation of pulpal soft tissue , working as a heat sink under heating and as a heating source when subjected to cooling.
1.4
Thermally induced damage
Abrupt changes in tooth temperature can cause TTP and damage in tooth hard components (enamel and dentine), soft pulp tissue and restorative interface ( e.g., interfacial debonding between the tooth and the DRMs) .
Enamel and dentine have different thermal and mechanical properties. For example, the thermal diffusivity (TD) and Young’s modulus of enamel are ∼2.5 and ∼4 times larger than that of dentine, respectively . The difference in these properties may result in thermal stresses and cracking within tooth when subjected to thermal stimulus , Fig. 2 . In addition, heat generated during laser treatment may result in carbonization, melting and cracking of the tooth structure . Thermal denature of dentine collagen often occurs in endodontics treatments, where the tooth root canal dentine may be exposed to a temperature of ∼300 °C . The denaturation temperatures of demineralized dentine matrix have been reported to be 65.6 °C, 148.5 °C, and 166.8–172.7 °C for demineralized dentine saturated with water, saturated with methanol, ethanol or acetone, and bonded with resin, respectively .

Dental pulp, which is responsible for the maintenance of tooth vitality, is vulnerable to heat. When the IPTR exceeds ∼5.5 °C, irreversible pulpal damage ( e.g., cell death in pulp) will be induced . However, pulp cells may survive such injuries . This may be due to the increased synthesis of heat-shock proteins (HSPs) . Amano et al. found that a heat stimulation of 42 °C decreases dental pulp cell viability, whereas the HSPs would help recovering the pulp cell vitality.
Current restorative techniques are based on the adhesive properties of DRMs , which work normally in the temperature range of −5 to 55 °C . Differences in TPs between tooth and DRMs facilitate the development of thermal stresses , with maximum stress developed on the bonding interfaces . Together with stresses caused by mastication, these stresses can induce degeneration of the bonding interface and hence reduce the life-span of dental restorations .
1.5
Applications of tooth HT
The study of HT in tooth and thermally related effects ( e.g., damage) is of great significance in modern dentistry, as summarized below.
- (i)
Precise temperature measurement in tooth may indicate the pulp vasculature and hence help in endodontic diagnosis .
- (ii)
In vitro investigation of HT in tooth under dental treatments can provide better approaches to evaluate the operation guidance, thereby reducing or preventing tooth damage so that a less empirical dental practice may be developed.
- (iii)
It can help in choosing and designing suitable DRMs with thermomechanical properties similar to toot, so that post-operative complications such as pain, marginal staining and caries may be avoided .
- (iv)
Tooth pain sensation is correlated with DFF .
A better understanding of HT phenomenon and thermally induced DFF in dental tubules will extend existing knowledge underlying TTP mechanisms and thus improve the relief methods for tooth pain sensation.
1.6
Outline of this review
In view of the lack of a thorough review of the current development in tooth HT, we present the state-of-the-art of existing studies on experimental characterization and mathematical modeling methodologies. The challenges associated with these methodologies and potential modifications essential for addressing these challenges are discussed. Interpretation of TTP in terms of heat stimulated DFF is also presented.
2
Experimental characterization of HT in tooth
Investigation of HT in tooth requires both experimental measurements and mathematical modeling . Mathematical models can supply estimates of information ( e.g. , temperature and thermal stress) over the entire continuum of interest, while experimental characterizations can validate the mathematical models and determine the various material properties ( e.g. , thermal, optical and mechanical) needed for theoretical modeling.
2.1
Measurement of tooth TPs
Knowledge of tooth TPs is crucial for accurate mathematical modeling and analysis of HT in tooth. The most important material properties for HT in tooth are tooth geometry, TC ( k , W m −1 K −1 ), specific heat ( c p , J kg −1 K −1 ), and TD ( α , m 2 s −1 ). In view of physics, TC indicates the ability of a material to conduct heat and is given as <SPAN role=presentation tabIndex=0 id=MathJax-Element-1-Frame class=MathJax style="POSITION: relative" data-mathml='k=(ΔQ/Δt)×(L/A×ΔT)’>k=(ΔQ/Δt)×(L/A×ΔT)k=(ΔQ/Δt)×(L/A×ΔT)
k = ( Δ Q / Δ t ) × ( L / A × Δ T )
where Δ Q (J) is the heat transmitted during time Δ t (s) through a thickness L (m), in the direction normal to a surface of area A (m 2 ), due to a temperature difference Δ T (°C). Specific heat is the energy required to increase a unit of temperature of a unit quantity of a substance. Thermal diffusivity <SPAN role=presentation tabIndex=0 id=MathJax-Element-2-Frame class=MathJax style="POSITION: relative" data-mathml='α≡k/ρcp’>α≡k/ρcpα≡k/ρcp
α ≡ k / ρ c p
is the ratio of TC to volumetric heat capacity, ρ (kg m −3 ) being the density of the material.
Traditional HT instruments and methods have been widely used to characterize the TPs of tooth components, such as the thermal couples , infrared camera (IR) technique , flash laser method , differential scanning calorimeter (DSC) , and traditional calorimeter cup . The reported data on human teeth are summarized in Table 3 , which shows significant differences between reported results. For example, TC of human dentine was reported to be ∼0.11 W m −1 K −1 in one study , but to be 0.96–0.98 W m −1 K −1 in others . Similarly, the reported TC of dentine covers a wide range of 0.36–0.88 W m −1 K −1 . Whilst one study reports the TD of enamel to be 2.27 × 10 −7 m 2 s −1 , its was measured to be ∼4.20–4.69 × 10 −7 m 2 s −1 by others .
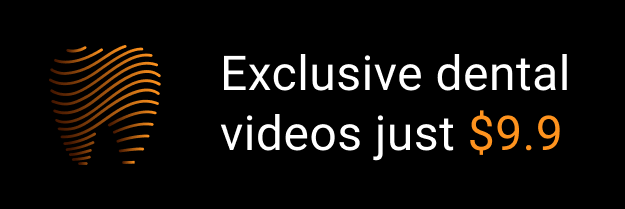