Abstract
Objective
Adhesion or cohesion includes an adherend, adhesive, and intervening interface. Adhesive joints may include one or more interfaces. Adhesion science focuses on understanding the materials properties associated with formation of the interfaces, changes in the interfaces with time, and events associated with failure of the interfaces.
Methods
The key principles for good interface formation are creation of a clean surface, generation of a rough surface for interfacial interlocking, good wetting of the substratum by the adhesive/cohesive materials, adequate flow and adaptation for intimate interaction, and acceptable curing when phase changes are required for final joint formation.
Results
Much more effort is needed in the future to carefully assess each of these using available testing methods that attempt to characterize the energetics of the interfaces. Bonding involves potential contributions from physical, chemical, and mechanical sources but primarily relies on micro-mechanical interaction for success. Characterization of the interface before adhesion, during service, and after failure would be much more useful for future investigations and remains as a great challenge.
Significance
Scientists should more rigorously apply techniques such as comprehensive contact angle analysis (rather than simple water wettability) for surface energy determination, and AFM in addition to SEM for surface texture analysis.
1
Introduction to adhesion science in dentistry
Everyone has an image of adhesion that includes glue, tape, barnacles sticking to boats, insects walking on ceilings, children’s stickers, and a host of dental materials examples. Adhesion involves the tendency of atoms or molecules to stick to each other. Strictly speaking, cohesion involves like materials sticking together, and adhesion involves dissimilar atoms or molecules sticking together. Both events are relevant to dental materials. This paper deals with both but will focus mostly on adhesive events.
Early records of cohesion techniques such as cold welding of gold and silver from the 1st and 2nd centuries BC are applicable to the fabrication of direct gold foil restorations, which were used in restorative dentistry. Cold welding is an example of cohesion, not adhesion, but the dental example involves both cohesion of the gold and adhesion (mechanical interlocking) into the prepared cavity. Another early example of adhesion which is used in dentistry today came from the 4th–7th centuries AD, enamelling for artwork and jewelry. This type of adhesion is used for ceramo-metal restorations. A non-dental example is when Sir Isaac Newton observed in the 17th Century that if he placed two transparent optical elements of the same refractive index together, there was no reflection at the point of contact. This principle formed the basis for building interferometers. The consequences of different types of interfaces will be discussed in the following sections.
2
Science of adhesion
It is important at this point to acknowledge that the science for creating adhesion or cohesion involves quite different energetic considerations than that science of interpreting their associated failures. The topic of adhesion includes (1) formation of adhesion-or-cohesion, (2) characterization of the adhesive-or-cohesive interfaces, (3) destruction of the interfaces (bond strength testing), and (4) failure analysis of interfaces. Our focus will be primarily on the first two topics, since it is clear that separation of a bonded joint is not simply the reverse of making that joint in the first place. Data on joint breaking strengths teach little about the surface properties initially supporting good bond formation.
2.1
Definitions for adhesion and cohesion
Adhesion involves molecular interactions at the interface between materials. Any event described as adhesion is really an assembly involving an “adherend” (or substratum) with an applied “adhesive” that creates an intervening “interface.” The combination is defined as an “adhesive joint.” Quite often a joint is more complex and includes two or more interfaces. In dentistry adherends can be quite varied (e.g., enamel, dentin, amalgam, composite, ceramic, cast metal, glass ionomer, etc.) and adhesives can involve single interfaces (e.g., sealants, ceramic bonded to metal) or multiple ones (composite bonded to dentin, ceramic restorations bonded to tooth structure). Any interface may include adhesion and/or cohesion .
2.2
Cohesion versus adhesion
Cohesion involves intermolecular attractions between like-molecules/atoms and is often reported as the cohesive strength of a material or tissue. For example, when measuring bond strength between dentin and an adhesive, we often examine the fracture surfaces to determine which of the materials actually failed and thus, determine whether the strength value measured is truly a measure of the bond strength, or is a measure of the cohesive strength of the adhesive, the adherend or some combination of the two.
Cohesion involves primary bonding and may include strong secondary bonding such as hydrogen bonding. It is a relatively strong force among polar molecules such as water, in which hydrogen bonding forms extensively. The propensity for water droplet formation on low energy surfaces is, in part, a result of cohesive forces in water. Higher energy surfaces better compete with water’s cohesive forces and thus the water droplets are flatter or spread completely. An example of cohesion inside of a restorative dental material is gold foil. The “sea of electrons” forms a strong bond from its overlapping metallic bonds among gold atoms. At the same time, the restoration is held in place within tooth structure by gross mechanical interlocking.
2.3
Types of adhesion
Adhesion or cohesion may be categorized by the type of physical, chemical, and/or mechanical bonding processes that contribute to the interfacial strength of the assembly. Remember that bonding occurs within the adherend, within the adhesive, and between the two at the interface. We are most often focusing on the bonding at the interface. Physical bonding forces are generally very weak. Chemical bonding is strong but also very difficult to produce in a dense manner across an interface. Mechanical bonding is the most effective means of creating strong joints. Each includes different subtypes as well. Each will be considered in more detail as follows.
Physical adhesion or bonding is always present even though it is weak. Thus, while van der Waals forces occur at every interface, they are often supplemented by significant contributions from stronger bonds that may be present, such as those from permanent dipoles in addition to the van der Waals induced dipoles. For example when water is present, such as water between two glass slides, the permanent dipoles allow for hydrogen bonding at the interface, but such bonding does not occur to polyethylene or other polymers which lack H-bonding partner sites .
Chemical bonding includes possibilities for covalent, ionic, metallic, and, in some cases, chelation bonding. There are numerous possibilities for creating chemical bonding. As previously noted, cohesion of gold to itself will create an interface that essentially disappears as the surface atoms become bonded. However, for other systems, trying to create bonds between unlike surfaces such as metal–ceramic and polymer–polymer interfaces, the situation is much more complicated and may result in very limited chemical bonding and much lower interfacial strengths.
There are many examples of creative ways to promote some chemical adhesion by using organo-silane coupling agents. Despite opportunities for chemical reactions or for chelation reactions, most of the moieties involved do not produce much chemical bonding at all. Rather they function to dramatically improve the wetting of phases and act by increasing the adaptation of materials along interfaces.
Chemical adhesion has been sought for decades in numerous dental materials applications. The results have largely been disappointing. Contaminants are hard to eliminate completely and interfere with the intimate adaptation needed to form bonds. At the same time, the theoretical bond densities that could form are quite small and therefore are normally overshadowed by mechanical bonding.
Mechanical interlocking is a common type of adhesion. This is certainly true for dental materials as well . There are several different variations on the theme, but all involve the adhesive penetrating into the adherend and becoming mechanically interlocked at some level. A classic non-dental example is a Velcro closure. A simple dental example is an amalgam restoration placed into cavities with undercuts to provide retention. Other examples cover the spectrum of interlocking, from dental cements that fill the roughness in castings and help retain them, to the penetration of monomers into etched enamel, which are then polymerized to form tags of several micrometers in size for retention. There is also permeation of monomers throughout the collagen fibril matrix in etched dentin to form the hybrid layer. Heterogeneity of the adherend microstructure contributes a variety of options for mechanical interlocking.
2
Science of adhesion
It is important at this point to acknowledge that the science for creating adhesion or cohesion involves quite different energetic considerations than that science of interpreting their associated failures. The topic of adhesion includes (1) formation of adhesion-or-cohesion, (2) characterization of the adhesive-or-cohesive interfaces, (3) destruction of the interfaces (bond strength testing), and (4) failure analysis of interfaces. Our focus will be primarily on the first two topics, since it is clear that separation of a bonded joint is not simply the reverse of making that joint in the first place. Data on joint breaking strengths teach little about the surface properties initially supporting good bond formation.
2.1
Definitions for adhesion and cohesion
Adhesion involves molecular interactions at the interface between materials. Any event described as adhesion is really an assembly involving an “adherend” (or substratum) with an applied “adhesive” that creates an intervening “interface.” The combination is defined as an “adhesive joint.” Quite often a joint is more complex and includes two or more interfaces. In dentistry adherends can be quite varied (e.g., enamel, dentin, amalgam, composite, ceramic, cast metal, glass ionomer, etc.) and adhesives can involve single interfaces (e.g., sealants, ceramic bonded to metal) or multiple ones (composite bonded to dentin, ceramic restorations bonded to tooth structure). Any interface may include adhesion and/or cohesion .
2.2
Cohesion versus adhesion
Cohesion involves intermolecular attractions between like-molecules/atoms and is often reported as the cohesive strength of a material or tissue. For example, when measuring bond strength between dentin and an adhesive, we often examine the fracture surfaces to determine which of the materials actually failed and thus, determine whether the strength value measured is truly a measure of the bond strength, or is a measure of the cohesive strength of the adhesive, the adherend or some combination of the two.
Cohesion involves primary bonding and may include strong secondary bonding such as hydrogen bonding. It is a relatively strong force among polar molecules such as water, in which hydrogen bonding forms extensively. The propensity for water droplet formation on low energy surfaces is, in part, a result of cohesive forces in water. Higher energy surfaces better compete with water’s cohesive forces and thus the water droplets are flatter or spread completely. An example of cohesion inside of a restorative dental material is gold foil. The “sea of electrons” forms a strong bond from its overlapping metallic bonds among gold atoms. At the same time, the restoration is held in place within tooth structure by gross mechanical interlocking.
2.3
Types of adhesion
Adhesion or cohesion may be categorized by the type of physical, chemical, and/or mechanical bonding processes that contribute to the interfacial strength of the assembly. Remember that bonding occurs within the adherend, within the adhesive, and between the two at the interface. We are most often focusing on the bonding at the interface. Physical bonding forces are generally very weak. Chemical bonding is strong but also very difficult to produce in a dense manner across an interface. Mechanical bonding is the most effective means of creating strong joints. Each includes different subtypes as well. Each will be considered in more detail as follows.
Physical adhesion or bonding is always present even though it is weak. Thus, while van der Waals forces occur at every interface, they are often supplemented by significant contributions from stronger bonds that may be present, such as those from permanent dipoles in addition to the van der Waals induced dipoles. For example when water is present, such as water between two glass slides, the permanent dipoles allow for hydrogen bonding at the interface, but such bonding does not occur to polyethylene or other polymers which lack H-bonding partner sites .
Chemical bonding includes possibilities for covalent, ionic, metallic, and, in some cases, chelation bonding. There are numerous possibilities for creating chemical bonding. As previously noted, cohesion of gold to itself will create an interface that essentially disappears as the surface atoms become bonded. However, for other systems, trying to create bonds between unlike surfaces such as metal–ceramic and polymer–polymer interfaces, the situation is much more complicated and may result in very limited chemical bonding and much lower interfacial strengths.
There are many examples of creative ways to promote some chemical adhesion by using organo-silane coupling agents. Despite opportunities for chemical reactions or for chelation reactions, most of the moieties involved do not produce much chemical bonding at all. Rather they function to dramatically improve the wetting of phases and act by increasing the adaptation of materials along interfaces.
Chemical adhesion has been sought for decades in numerous dental materials applications. The results have largely been disappointing. Contaminants are hard to eliminate completely and interfere with the intimate adaptation needed to form bonds. At the same time, the theoretical bond densities that could form are quite small and therefore are normally overshadowed by mechanical bonding.
Mechanical interlocking is a common type of adhesion. This is certainly true for dental materials as well . There are several different variations on the theme, but all involve the adhesive penetrating into the adherend and becoming mechanically interlocked at some level. A classic non-dental example is a Velcro closure. A simple dental example is an amalgam restoration placed into cavities with undercuts to provide retention. Other examples cover the spectrum of interlocking, from dental cements that fill the roughness in castings and help retain them, to the penetration of monomers into etched enamel, which are then polymerized to form tags of several micrometers in size for retention. There is also permeation of monomers throughout the collagen fibril matrix in etched dentin to form the hybrid layer. Heterogeneity of the adherend microstructure contributes a variety of options for mechanical interlocking.
3
Requirements for creating good adhesion
There are several sequential events that are required to form an effective adhesive/cohesive joint. These are discussed in detail as follows.
3.1
Clean surfaces
A principal requirement for strong adhesive bonds is that the surface be clean and therefore in a high energy state. Films of water, organic debris, and/or biofilms are always present in the clinical situation, and interfere with wetting and spreading. These cannot be removed by tooth brushing with dentifrices alone. Teeth that are prepared for dental restorative procedures have low energy surfaces because of contaminants and smear layer that remain on the surface. Acid etching removes most of the contaminants, produces surface roughness for micro-mechanical interlocking, and forms facets on the mineral crystals . Etched enamel is wet readily by monomers, allowing good penetration, and forms micro-mechanical bonds easily . Hydrophilic monomers can penetrate into surface cracks among or within the enamel crystals and thus help resist crack propagation after polymerization. The process also may displace any water layer on the prepared surface. Organic groups that enhance wetting include –OH, –SH, –COOH, and –NH 2 . Unfortunately the presence of F discourages wetting .
3.2
Surface roughness
Wettability is enhanced for most practical dental situations by the presence of microsurface roughness. Wettability is related to roughness by the Wenzel equation that states that:
r = cos 1 cos 2 ,
where r is the ratio of the contact angles of the smooth and rough surfaces, respectively. This equation predicts that for contact angles less than 90°, wetting is increased by surface roughness, but decreased for non-wetting materials with contact angles greater than 90°. The Wenzel effect has been confirmed for a number of dental materials investigations including polymer surfaces , cements , and composites .
3.3
Proper contact angle and good wetting
Adhesion requires intimate contact of the materials to be joined. Since the surface of a material is different from the interior, understanding of the surface characteristics of materials is essential to understanding and promoting adhesion. In the interior atoms are in equilibrium and the interatomic forces between adjacent atoms in the crystal structure are in balance. At the surface this is not true because there are no interatomic interactions on the external surface of the atoms. The surface free energy, γ , represents the difference between an atom on the surface and an atom in the interior . The affected surface layer is likely to be at least 5 atomic layers thick . Surface physics and chemistry are affected in adhesives or tissues .
For adhesion to occur the adhesive must wet the substratum. The most common method of observing wetting is measuring the contact angle. The contact angle is the internal angle in a droplet of liquid in contact with a solid. It represents the energetic equilibrium between the solid, liquid, and gas phases involved. In most cases, the gas phase is air, and one simply focuses on the liquid droplet and solid substratum because the gas phase at the operative microscopic solid/liquid/gas interface is always fully saturated with the liquid’s vapor.
Wetting is categorized from liquid (usually water, but not necessarily) contact angle as non-wetting (>90°), wetting (<90°), and spreading (∼0°), although all liquids do wet all solids to some extent; degree of wetting = degree of adhesion. The goal is always to select conditions that promote spreading, without going so low in liquid surface tension that the liquid’s cohesive strength is adversely diminished. The equation governing the energy balance in an interface is Young’s equation :
γ s l = γ s v + γ l v cos ,
where “ ” is the contact angle, and “ s ”, “ v ,” and “ l ” refer to the solid, liquid, and vapor phases, respectively. The “critical surface tension” of a solid is related to its surface free energy and is determined from a graph of liquid surface tension versus contact angle, when various liquids of known surface tension are plotted versus contact angle. The critical surface tension is the value obtained when the graph is extrapolated to a contact angle of 0°.
Contact angles of common liquid/material combinations are in Table 1 .
Teflon–Hg | 150 |
Teflon–water | 112 |
PE–water | 103 |
Skin–water | 90 |
Gold–water | 66 |
Pt–water | 40 |
Glass–water | <5 |
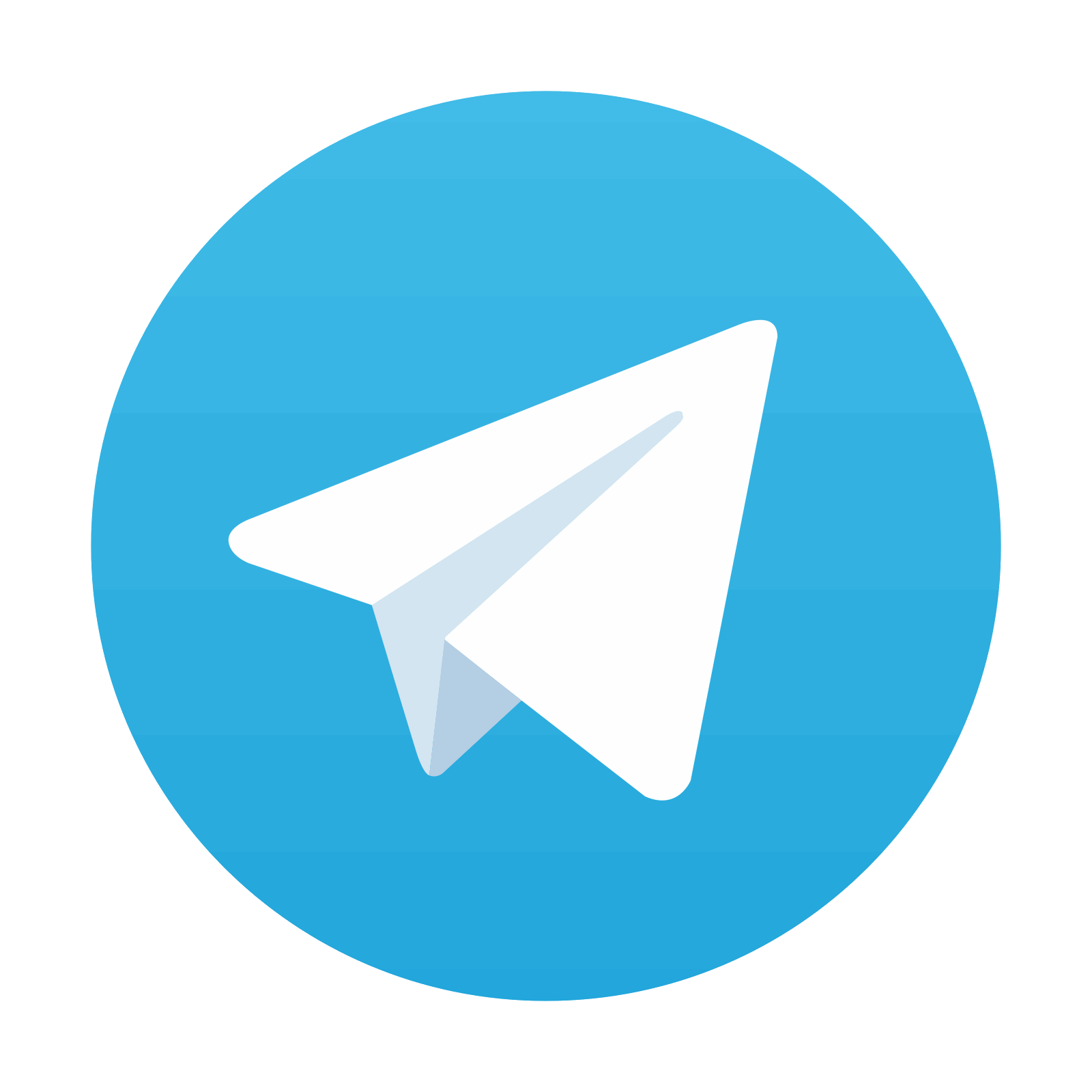
Stay updated, free dental videos. Join our Telegram channel

VIDEdental - Online dental courses
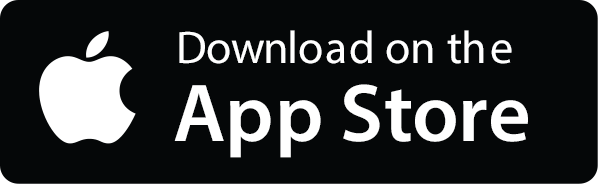
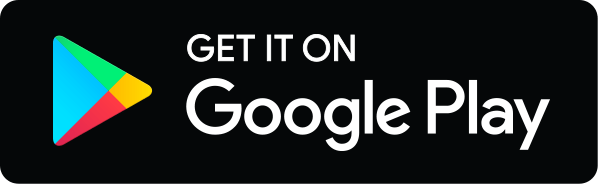
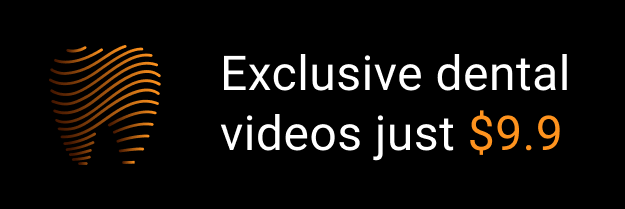