Abstract
Artificial bones are useful for tissue augmentation in patients with facial deformities or defects. Custom-made artificial bones, produced by mirroring the bone structure on the healthy side using computer-aided design, have been used. This method is simple, but has limited ability to recreate detailed structures. The authors have invented a new method for designing artificial bones, better customized for the needs of individual patients. Based on CT data, three-dimensional (3D) simulation models were prepared using an inkjet printer using plaster. The operators applied a special radiopaque paraffin wax to the models to create target structures. The wax contained a contrast medium to render it radiopaque. The concentration was adjusted to achieve easy manipulation and consistently good-quality images. After the radiopaque wax was applied, the 3D simulation models were reexamined by CT, and data on the target structures were obtained. Artificial bones were fabricated by the inkjet printer based on these data. Although this new technique for designing artificial bones is slightly more complex than the conventional methods, and the status of soft tissue should also be considered for an optimal aesthetic outcome, the results suggest that this method better meets the requirements of individual patients.
Autograft , allograft , and artificial bones have been used to reconstruct cranio-facial bone defects. Artificial bones do not require bone collection from healthy donor sites, in contrast to autografts. Artificial bones are free from the cross-contamination from donors associated with allografts. Various types of artificial bones have been developed for clinical . The requirements of artificial bones include easy handling, contours that fit defects well, appropriate mechanical strength, good biodegradability, and good bone-regeneration inducing capacity. No artificial bone meets all these requirements. Contours are important for aesthetic purposes, and are a key to success for clinical use in the cranio-facial region.
Although there have been reports of custom-made artificial bones and titanium trays , these were fabricated using computer aided design (CAD), which is unable to recreate the subtle shapes demanded by surgeons.
The authors present a novel method for designing and fabricating custom-made artificial bones, which precisely reflects mental images by surgical operators. They also report on the efficacy of the bones in clinical applications.
Materials and methods
Fabrication of 3D simulation models
Computed tomography (CT) of the cranio-facial portions of the patients was performed using Aquilion 16 (Toshiba, Tokyo, Japan) with 0.5 × 16 mm/rotation (120 kV, 300 mA) and 120 kV and 300 mA. The CT data were converted to DICOM data, and three-dimensional (3D) images were reconstructed using Mimics software version 11.2 (Materialise, Leuven, Belgium). The data were saved as Standard Template Library (STL) data. The final STL data were input into a 3D inkjet printer.
Inkjet printing is a rapid manufacturing technology that fabricates 3D models from CAD data. The process consists of forming layers (0.1 mm thick) by using a printer-like device to distribute an adhesive solution to bond the surface of a powder into the desired shape. The printer (Z406 3D colour printer: Z-Corporation, Burlington, MA, USA) ejects a liquid binder from the inkhead; a solid figure forms with the repetitive hardening of the powder on the flat surface. The powder used was plaster (ZP140, Z-Corporation). The binder agent was ZB56 Clear Binder (Z-Corporation). The manufacturing precision was ±<1.0 mm.
Modification of wax with contrast agents
For surgical operators to build structures on top of the plaster 3D models, the authors chose dental wax as a material. Wax is thermoplastic and is easy to manipulate repeatedly. Plaster does not appear appropriate because it is either too runny or too quick-setting for building complex structures. There would be too low a contrast between the 3D models and the built structures if plaster was used, even if it was mixed with contrast agents, because it is the same material with which the 3D models are made, making it difficult for built structures to be identified by CT. To optimize the contrast with both plaster and air, dental paraffin wax was modified by mixing it with four medical contrast agents (calcium sulphate dihydrate, barium sulphate, anatase-type titanium oxide, and rutile-type titanium oxide) at different ratios. The modified waxes were poured into moulds made of ZP140, and CT images were taken under the same conditions to obtain the CT values.
Simulation using 3D plaster models and special wax
To perform simulation using plaster models and special wax, the surgical operators melted wax and used spatulas to form artificial bones in the plaster models .
Custom fabrication of artificial bones
After simulation, CT was performed on the simulation models, the shapes of the custom-made artificial bones were extracted using Mimics editing software, and the final designs of the artificial bones were determined using Magics software (Mateliarise). The final data were sent to the same type of 3D inkjet printer described above. This time, the powder used was α-TCP (Taihei Chemical Industrial, Nara, Japan) with a mean particle diameter of 10 μm. Instead of the adhesive agent, a curing solution was used, which was a mixture of 4% sodium chondroitin sulphate (Seikagaku Corp., Kanagawa, Japan), 12% disodium succinate (Wako Pure Chemical Industries, Saitama, Japan), and 84% distilled water (Otsuka Pharmaceutical Factory, Otsuka, Japan) .
Results
Selection of contrast agents for special wax
The authors first attempted to make a special radiopaque wax containing contrast agents for the simulation. To extract designs of artificial bones from 3D simulation models using wax, it was necessary to differentiate plaster (3D models) from air and wax in the CT images taken after the simulation. For this purpose, the authors modified dental paraffin wax by mixing it with four medical contrast agents at different ratios, and evaluated the CT values, the rates of change in these values at the boundary between wax and plaster, and the dispersivity of the contrast agents. For good differentiation, the CT value of the special wax had to be midway between that of air and that of plaster. As for the rates of change at the boundary between wax and plaster, the bigger the rate of change, the clearer the boundary should be between wax and plaster, enabling accurate recreation of simulated contours. As for dispersivity, the wax needed to be mixed homogeneously with each contrast agent. If the contrast agents are not homogeneously dispersed, they tend to settle down by gravity, causing an increase in CT values towards the bottom of the well. The authors defined the dispersivity as the inverse of the CT value difference between the top and bottom portions of the wax in the well.
To evaluate these three parameters, the authors poured various modified waxes into moulds made of the same plaster for the 3D models and took CT images under the same conditions ( Fig. 1 ). The results are shown in Table 1 .
Agent | Compounding ratio | CT value | Rate of change in CT value (-/mm) | Dispersivity of contrast agent (×10 −3 ) |
---|---|---|---|---|
a. Wax only | – | 151 | 207 | 3.83 |
b. Wax:calcium sulphate | 10:3 | 359 | 253 | 5.18 |
c. Wax:calcium sulphate | 20:3 | 225 | 299 | 5.18 |
d. Wax:calcium sulphate | 40:3 | 189 | 252 | 6.99 |
e. Wax:titanium oxide (anatase) | 20:3 | 240 | 274 | 1.70 |
f. Wax:titanium oxide (anatase) | 40:3 | 166 | 252 | 1.68 |
g. Wax:titanium oxide (anatase) | 80:3 | 177 | 324 | 1.98 |
h. Wax:titanium oxide (rutile) | 20:3 | 266 | 311 | 2.97 |
i. Wax:titanium oxide (rutile) | 40:3 | 195 | 244 | 3.40 |
j. Wax:titanium oxide (rutile) | 80:3 | 175 | 341 | 5.15 |
k. Wax:barium sulphate | 80:3 | 199 | 239 | 1.75 |
l. Wax:barium sulphate | 160:3 | 184 | 276 | 2.59 |
CT values
The CT values of plaster and air were 518 and −1024, respectively, with a mean of −253. When the CT values of the modified waxes were compared with this mean value, the three closest in number were those of the wax containing rutile-type titanium oxide at 80:3, that containing anatase-type titanium oxide at 40:3, and the wax only.
Rates of change in CT values at the boundary
Figure 2 shows the method used to measure the rate of change in a CT value at the boundary between wax and plaster. When the authors compared the rates between plaster and the wax mixtures, the three with the highest rates were the wax containing rutile-type titanium oxide at 20:3, that containing rutile-type titanium oxide at 80:3, and that containing anatase-type titanium oxide, also at 80:3.
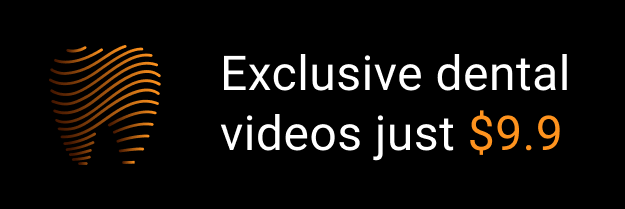