Understanding Laser Photobiomodulation
Antonio Luiz B. Pinheiro, Aldo Brugnera Júnior, Fátima A. A. Zanin
Scientific Basis
Research into the role of laser phototherapy, also known as low intensity and low power laser therapy, began in the late 1960s in eastern Europe being its earliest experimental application reported in 1968 by Endre Mester in Hungary who revealed that a ruby laser treatment accelerated healing of mechanical wounds and burns in mice.
Early laser reports were very enthusiastic and many times anecdotal, and offered no understanding of its mechanism. Since the 60s’ the volume of research into laser phototherapy has grown substantially and has focused on three areas to assess the value of laser phototherapy in wound repair: cellular function, animal studies and human trials.
Ewemeka (2005) commented that as the technology has evolved so have the acronyms used to describe the therapy. However, none of the commonly used terminologies, including low level laser therapy (LLLT) low energy laser therapy (LELT), low-intensity laser activated biostimulation (LILAB), low-power laser irradiation (LPLI), low power laser therapy (LPLT), and monochromatic infra red energy (MIRE) adequately accounts for this shift in technology. Moreover, so much confusion has arisen from describing the same treatment in so many ways that some now resort to the combined use of “low level laser therapy,” “low-energy,” “cold laser,” “low power,” and “soft laser” to ensure clarity. It was recommended to streamline things, minimize the on-going confusion, and stem further proliferation of acronyms as the technology continue to evolve. “Light therapy” or “phototherapy” should be used to describe noninvasive interventions involving therapeutic light, for example, situations involving cutaneous and subcutaneous tissue irradiation for tissue repair, light-based acupuncture, and transcutaneous irradiation for pain relief. “Photobiomodulation” or “Photo medicine” may be appropriate to describe these interventions, but they broadly cover invasive light therapy, such as direct irradiation of stomach ulcer, cardiac infarct, or fracture using a catheter as well. Similarly, “photo irradiation” may be appropriate to describe in vitro irradiation of cell cultures and tissues.
There are a considerable number of different types of laser used for medical purposes. For therapeutic purposes, we use radiation intensities so low that it is thought that any biological effects that occur are due to the direct effects of radiation rather than the result of heating. Energies delivered are typically about 10 joules per square centimeters, using lasers operating at powers of 50mW or less.
Laser phototherapy is a modality of clinical treatment, which results on non-thermal effects on the tissues, and its biological effects cannot be attributed to heating. The magnitude of its effects depends on the physiologic status of the cells or the clinical stage of the condition before irradiation. This may explain why positive photobiomodulation may not always be detectable. Some people suggested that the effect of heat generation on the irradiated tissue has occasionally been asserted that the “possible” effects of laser phototherapy were due to the laser heating the tissue. However we need to consider that one could just as well use a blanket, hot shower or a heat-lamp to get similar responses, but this do not happen at all. Heat, however, can of course be valuable many times, get positive response from tissues, but in different magnitudes and manners.
The HeNe laser was the first laser available and is reported to have beneficial effects in both wound healing and dentistry. The HeNe laser has the advantage that it emits red light, which is visible and therefore the blink reflex protects the eye from it. The GaAs and GaAlAs laser have been most commonly used for the treatment of pain and inflammation and in lower doses for wound healing as they have deeper tissue penetration than the He-Ne laser. These lasers have the disadvantage that their light is invisible and therefore eye protection is required.
Laser Phototherapy Pathways
Light is absorbed in irradiated tissue by a variety of Light is absorbed in irradiated tissue by a variety of chromophores that absorb photons and light is transformed into biochemical energy by multiple secondary reactions resulting on the restoration of cellular function, reduction in pain and inflammation, and enhancement of wound healing processes.
The analysis of the literature elsewhere leads to the understanding that the primary mechanisms of the laser phototherapy are related to the interaction between photons and molecules within the tissue, while the secondary mechanisms are related to the effect of the chemical changes induced by the primary effects.
There are several possible primary reactions that can be found on previous reports. When a photon is absorbed it can transfer its energy to an electron in being the photon energy high enough, it can change the energy state of the electron. Also triplet states can be involved. It has also been shown that excitation can occur by means of multiple photon action.
The literature has pointed out several factors which are affected by laser phototherapy and these includes: transforming growth factor-beta, platelet-derived growth factor, Ca2+, enhancement of ATP production, prostaglandin reduction, Improved cell wall permeability, and on reactive oxygen species (H202, O22+). These factors are not only increased, they are modulated according to the oxidative state of the cell. For instance cells in 2% fetal calf serum react best, those in 5% well and those in 10% hardly at all. This is a key for understanding the effect of light on living tissues. Some kind of deficiency has to be present. Laser phototherapy is optimal when the function of the cell, that is the redox situation, is sub-optimal. Healthy cells react little or do not react at all to light energy.
Photosensitivity of the cells to the laser is not of the type “everything or anything.” On the contrary, several degrees of responses may by triggered on cells that may be more or less sensitized, depending upon their physiologic status prior to the irradiation. It is not surprising that laser phototherapy may not show evident influence of show any effect at all on “healthy” subjects who are in physiologic balance.
There are several specific effects of the laser phototherapy which are widely reported on the literature and these includes altered nerve conduction; altered blood flow and neoangiogenesis; increased metabolism of endorphins, acetylcholine, serotonin, cortisol; enhanced ATP production; enhanced cell function (e.g. release of growth factors, cytokine reactions, replication); increased synthesis of DNA and proteins; and acceleration of healing. Secondary effects may be due to the fact that laser-excited form of ATP has different biochemical behaviour than non-excited form. It has an increased kinetics.
Tissue Optics
Light is isotropically scattered in all directions and, because of this, the effects of the light is independent of its direction due to the isotropic diffusion, which creates spherical equal intensity zones. Polarization propagation in biological tissues is a complicated process that is fundamental to tissue optics. Several parameters, such as size, shape, refractive index, concentration of the scattering particles, and incident polarization state play important role in the scattering of light. Usually when the light passes through biological tissues it follows a tortuous path and scatters many times. As a result of these diffuse multiple scattering, the direction, polarization and coherence are randomized. Mammalian tissues are a highly scattering medium for light with wavelengths between λ600-λ1300nm (Fig. 9.1).
Figure 9.1 • The nature of the irradiated tissue may reflect the properties of the laser light.
Approximately 5-7% of the light incident is reflected back to the environment because of the folds in the stratum corneum and the remaining is transmitted to the next layer, the epidermis, this phenomenon is known as surface scattering (Fig. 9.2).
Figure 9.2 • Diagram showing the backscattering of the incident light.
The epidermis also propagates and absorbs light mainly due to the presence of the melanin. Higher absorption occurs at shorter wavelengths. The level of absorption of melanin depends on the number of melanossomes per unit volume are in the epidermis. The scattering on this layer is mainly due to the presence of particles that are approximately the same size of the wavelength of light. The dermis also propagates and absorbs light. The scattering on this layer occurs mainly due to the presence of thick collagen fibers. The light gets scattered several times on this layer before being either absorbed or propagated to the next layer. The hypodermis is characterized by negligible absorption of light in the visible region of the spectrum. The presence of adipose deposits causes the back reflection of the incident visible light to the upper layers. Up to the epidermis, the layers act as optical barriers. UV light is absorbed by amino acids, nucleic acids, and melanin. Most of UV light is absorbed at these layers because of the melanin. Visible and near IR light are less absorbed and are better transmitted. There is a consensus that there is an optical window between λ600 and λ1300nm.
Anderson and Parrish (1981) found that the reflection of a totally perpendicular beam directed to the skin is equivalent to 4-7% of the incident radiation. 93-96% of the incident light penetrates the skin with varied refraction indexes. On the skin reflection increases with the use of greasy substances, fluids or even if the sebaceous secretion is not cleared. As the energy is not absorbed it does not results on biological tissues but can be used as a diagnostic tool.
Absorption depends on the wavelength. As the light penetrates into the tissue it suffers constant reflection and refraction, this phenomena of interaction between the light and heterogeneous tissues is called diffusion or scattering. Scattering, also called scatter, is the process by which small particles suspended in a medium of a different index of refraction diffuse a portion of the incident radiation in all directions. In scattering, no energy transformation results, only a change in the spatial distribution of the radiation. Along with absorption, scattering is a major cause of the attenuation of radiation by the atmosphere. Scattering varies as a function of the ratio of the particle diameter to the wavelength of the radiation.
Rayleigh scattering is the scattering of the light by particles smaller than the wavelength of the light and occurs when light travels in transparent solids and liquids, but is most prominently seen in gases. Rayleigh scattering of sunlight from particles in the atmosphere is the reason why the light from the sky is blue. Occurs due to the presence of particles or molecules smaller than 1/10 of the incident λ. It is a very weak scattering and does not change the photonic distribution.
In contrast to Rayleigh scattering or Dipole scattering, the Mie theory embraces all possible ratios of diameter to wavelength. It assumes a homogeneous, isotropic and optically linear material irradiated by an infinitely extending plane wave. It occurs when the size of the particles are near the same of the incident λ and results in a strong forwards scattering. If the size of the particle is greater than the incident λ, it becomes weak. On the skin, the Mie scattering is predominant mainly due to the large amount of collagen fibers. In other tissues the scattering varies according to their composition (Fig. 9.3).
Figure 9.3 • Diagram showing the forward scattering of the light within the tissue. The presence of chromophores will interfere with this phenomena.
Transmitted light is that which passes trough a tissue without attenuation. It may occur depending on the dimension of the target and the penetration of the incident wavelength. The light returns to the origin medium.
The Cell
The cells are very complex biological units that are responsible for the maintenance of the life on living subjects. The cell possesses several organelles that are responsible for all of its functioning and proliferation. Several studies have pointed out that light at several wavelengths may stimulate or inhibit cell functions.
On many cells there are specialized photoreceptors or photo reversible photo chromatic pigments such as chlorophyll, rodopsin, bacteriorodopsin, and phitochromes (A, B, C, D) that regulate their speed of oxidation at environmental temperature and it has been shown that when irradiated with λ650nm, the speed is greater and at environmental temperature and irradiated with λ725nm, the speed of oxidation decreased and that at 0° the speed was duplicated. Karu (1985) showed their presence on HeLa cells and that they influenced the synthesis of DNA and RNA using λ620 and λ760nm, being the phenomenon reversible.
There are also non-specific photoreceptors such as flavoproteins, phorphirins (catalase, citochrome, citochromoxidase); and proteins with cupper (tyrosine, superoxide dismutase, ceruloplasmine, asparagines) that interfere with the cell metabolism without the light. However, when irradiated with specific wavelength, they absorb photons and interfere on the cell metabolism.
Flavoproteins are important primary photoreceptors as they catalyze several induced effects. Because of the huge numbers of primary photoreceptors on the cells, several reactions photo induced by specific wavelengths may not be attributed to just one photoreceptor.
Photoreceptors
Cytochrome c Oxidase is a terminal enzyme of the mitochondrial respiratory chain and an ATP producer. NADH-dehydrogenase is a flavoprotein found at the beginning of the respiratory chain (Fig. 9.4). Some human photo chemically reactive proteins are the encephalopsin in the brain and the pinopsin in the pineal gland. One of the main effects of the absorption of visible light is the stimulation of the mitochondria, which results in increased cell energy and activation of nucleic acid synthesis. Infrared light also results in the same, being the process initiated by a response at the membrane level. Laser photobiomodulation seems to be influenced by common characteristic of polarization of both types of light (Fig. 9.4).
Figure 9.4 • The complex Cytochrome c Oxidase molecule.
The absorption of the laser light may result on two types of effects: photochemical or photo physical.
The photochemical effect is a response of action of the low power and long time of delivering visible light on the mitochondria that result on increased levels of ATP. The photochemical effect is non-coherent in nature as coherence is lost due to scattering on the skin before absorption by the chromophores (melanin, hemoglobin, phorphirins, citochrome c oxidase and others). The photochemical effect depends upon the wavelength, dose and emission mode.
The photonic absorption occurs due to the presence of intracellular biomolecules and may cause stimulation or inhibition of enzymatic activity and photochemical reactions. Photodynamic changes may occur on complex chains and basic molecules of several physiologic processes with therapeutic repercussion.
The photo physical effect induces changes on the electrical potential of the cell membrane, increasing ATP synthesis. Pain transmission occurs due to changes on the electrophysiological state of the membrane of the neuron. Photochemical effect of visible light on the redox chain of the mitochondria and the photo physical effect of IR light on the cell membrane, which triggers the same cascade of cellular photo responses.
Mitochondrial ATP generated after laser irradiation shows some peculiar behavior. It increases DNA and RNA synthesis on eukaryotes and prokaryotes; increases collagen and its precursors; increases the release of b-endorphins; induces qualitative and quantitative changes on levels of prostaglandins; increases immunosupression, increases the adhesion of macrophages and lymphocytes. Other phenomenon can be also detectable such as on enzymes, on the release of acetylcholine, histamine and changes on the activity of NADH, catalase, citochromooxidase, acid phosphatases, superoxide dismutase, succinate and dehydrogenases. There are also reports on the effects on secretory glands.
Laser photobiomodulation also activates the Adenosine triphosphatase (ATPase), and the conversion of ATP to Adenosine. Adenosine stimulates the conversion of cAMP to nitric oxide (NO) or the vascular endothelial growth (VEGH). Both, fibroblast growth factor (FGF) and vascular endothelial growth factor (VEGH) are known to be angiogenic factors and they promote new vessel growth in the same manner.
The Mitochondrion
Mitochondria are tubular or filamentous organelles and are the largest organelle in most cells following the nucleus (about 1 by 5-10 microns). They are accumulated in the more active areas of cells (e.g. Base of renal tubular cell or apex of intestinal cell). They are surrounded by a double membrane (i.e. two membranes, each a phospholipid bi-layer). The inner membrane has many invaginations called cristae. This results in two spaces, an inner (or intercristal) mitochondrial space and an outer or intra-crystal space.
The intercristal space contains the Matrix. Inside the matrix there are a few ribosomes, RNA, mitochondrial DNA, and electron-dense granules rich in cations. Also present are the large enzymes complexes required for fatty acid oxidation and the Kreb’s cycle. On the inner mitochondrial membrane, are located the proteins of oxidative phosphorylation and the electron transfer chain. About 75% of the membrane is protein (highest % content for any eukaryotic cell membrane). In a typical liver mitochondrion, the inner membrane has a surface area five times the outer. Mitochondria grow and divide to produce new mitochondria. They are also digested to re-enter the metabolite pool inside the cell. Some recent studies looking at numerous electron micrographs of the same cell suggest that the mitochondria may actually be part of a budding, continuous network all over the cell (like the Endoplasmatic Reticulum) rather than discreet organelles.
There are some arguments whose were used against to the hypothesis that the mitochondrion is the cellular mediator of photobiomodulation, and these included: mitochondria can’t be the answer since all they do is make ATP; they can’t respond to cell stress or injury; they can’t regulate cell shape; they can’t regulate cell death and survival; they can’t regulate inflammation; they can’t regulate wound healing; they can’t regulate cell differentiation; they can’t regulate blastoma formation; they can’t regulate cell movement and morphogenesis; they can’t regulate tissue differentiation; and they can’t regulate cellular aging. All those are wrong!
ATP is the most important factors in all metabolic cell reactions and is key molecules for most cell processes. It provides free energy by coupling anabolic with catabolic reactions, and acts as a transducer in most metabolic and signaling cellular pathways (Ress & Howard, 1991; Schilling et al, 2000). The ATP is mainly synthesized by mitochondria following a series of redox reactions in the electronic transport chain located in the inner membrane of the organelle (Friedrich & Bottcher, 2004). In aerobic conditions 36-38 molecules of ATP are formed from one molecule of glucose (Bianchet et al, 2000). In anaerobic conditions, the glucose molecule is fully oxidized in the cytoplasm to produce two molecules of ATP and pyruvate by a process called glycolisis (Passarella et al, 1984). The pyruvate can be further processed anaerobically to lactate by lactic acid fermentation (Robergs et al, 2004). This reaction is the source of ATP when the mitochondria are unable to synthesize ATP and when the cell has low oxygen concentration (Westerblad & Allen, 2003).
Electrons are food for mitochondria. All the food we eat is made of organic polymers such as proteins, cats, carbohydrates that are broken down by oxidation (burning) into the mitochondria to yield electrons that are the fundamental food of the cell and can be used for work.
The cells produce a signal when they are fed and this signal is a flickering trickle of H2O2 that lets the nucleus knows the cytoplasm is well. In the right cells and conditions, H2O2 bursts produce depolarization. Light may also be produced that can be detected by, and attract neighboring cells
Experimental measurement of mitochondrial energy production is a function of its membrane potential and it is his is measured with potentiometer dyes such as TMRM, JC1, and rhodamine 123 (Fig.9.5).
Figure 9.5 • CHO-K1 irradiated cells present their mitochondria with granular aspect. A migration can be observed for the perinuclear area. JC-1, 4.500x
Glicolysis energy production is measured by the release of lactate. The measurement of levels of ATP, ADP, Pi, and creatine phosphate doesn’t reveal the source of energy. But Mitochondria don’t just make ATP!
The energy generated by the mitochondria originates from oxidation of reducing NADH and FADH2 via respiratory chain. Its terminal enzyme, cytochrome c oxidase, reduces dioxygen to water with four electrons from cytochrome c and four protons taken up from the mitochondrial matrix, without formation of ROS. The cytochome c oxidase also pumps protons from the matrix into the intermembrane space, forming an electrochemical proton gradient across the inner mitochondrial membrane, which is used by the ADP synthesis for the synthesis of ATP (Kanderbach et al, 2000). The cytochrome c oxidase in mammalians is formed by a large number of 10 nuclear-coded subunits and three catalytic mitochondrial subunits (Kanderbach et al, 1983).
In 1984 Passarella et al mentioned that the use of He-Ne laser light could change the optical and biochemical properties of both NADH and RNA and increase mitochondrial membrane potential, proton gradient and mitochondrial ATP (70%) synthesis and correlated these changes with the mitochondrial electron transfer chain and that the extra ATP synthesis was due to a laser-induced extra proton motive force.
Several kinds of evidence have shown that mitochondria are sensitive to irradiation with visible laser light (Figures 9.6, 9.7)
Figure 9.6 • Non irradiated CHO-K1 cells showing alterations in the cellular integrity; diffuse chromatin; with no mitotic activity. DAPI, 4.500x
Figure 9.7 • CHO-K1 irradiated cell showing mitotic activity and well preserved chromatin. DAPI, 4.500x
The effects of laser light on these organelles resulted on increased ATP using λ415 (Kato et al, 1981), λ602 (Vekshin & Mironov, 1982), λ632.8 (Passarella et al, 1984), λ650 and λ725nm (Gordon & Surrey, 1960). Wavelengths of λ477, λ511 and λ554nm (Kato et al, 1981) did not affected ATP synthesis. The consumption was activated by λ365 and λ436nm, but not at λ313, 546 and λ577nm (Vekshin & Mironov, 1982). The membrane potential and proton gradient are increased with λ632.8nm (Passarella et al, 1984). Laser light also causes changes in the mitochondrial optical properties and modifies some NADH-linked dehydrogenase reactions (Passarella et al, 1983). Increased rates of ADP-ATP exchange (Passarella et al, 1988) and of RNA and protein synthesis in the mitochondria were also reported previously (Greco et al, 1989). The use of λ630 (Hilf et al, 1986), λ632.8 (Karu et al, 1995) and λ820nm (Herbert et al, 1989) is able to increase the amount of ATP in different types of cells (Herbert et al, 1989; Passarella et al, 1988; Karu et al, 1995). Visible and NIR laser increase ATP synthesis (Passarellla et al, 1984, Karu et al, 1985) and Adenosine nucleotides are also affected by visible and NIR laser (Amat et al, 2004; Gagliardi et al, 1997). Within one hour of irradiation with He-Ne human peripheral blood lymphocytes show marked increase in the accessibility of total nuclear chromatin (Fedoseyeva et al, 1988), which correlates to intensified synthesis of both total and nuclear RNA and activational rearrangements of nucleoli (Manteifel et al, 1992) and chromatin (Manteifel et al, 1994).
Amat et cols found an activation threshold at 6J/cm2 and />
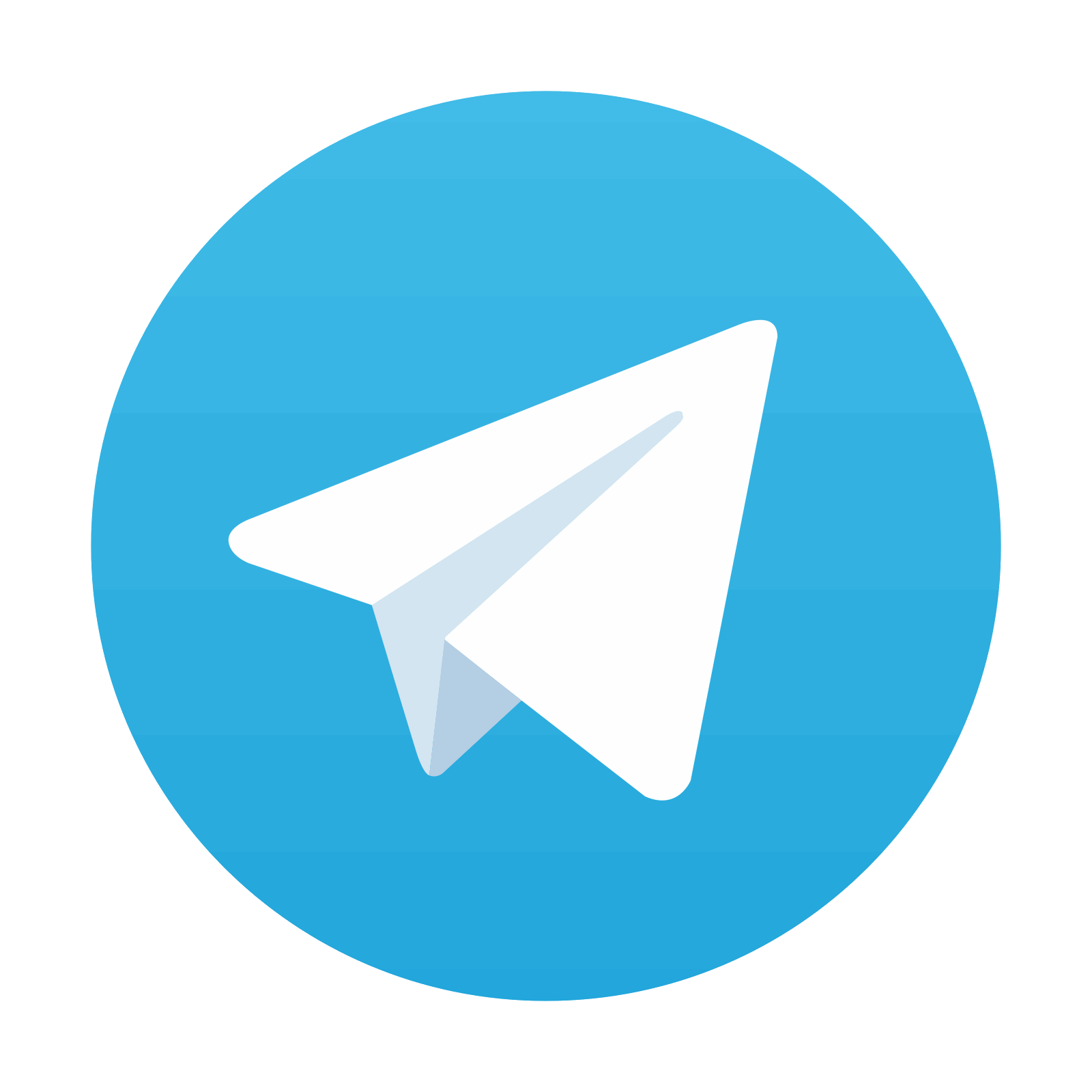
Stay updated, free dental videos. Join our Telegram channel

VIDEdental - Online dental courses
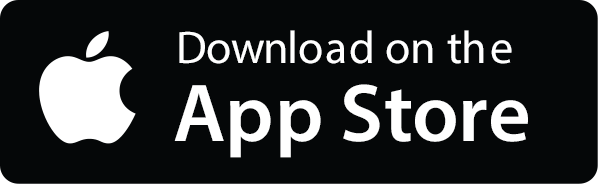
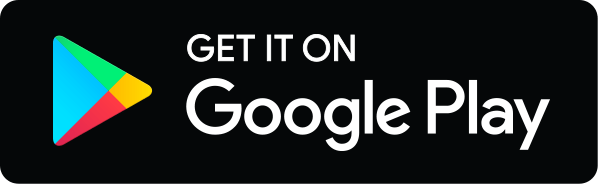