Stress Treatment Theorem for Implant Dentistry
The Key to Implant Treatment Plans
Carl E. Misch
Dentistry is a unique aspect of medicine, blending science and art form. Some aspects of the dental field emphasize the art form, as in dental esthetics, which deals with tooth color and shape to enhance a patient’s smile and overall appearance. In partially or completely edentulous patients, dental prostheses are responsible for dental esthetics (Figures 8-1 to 8-3). However, dental laboratory technicians are largely responsible for the final esthetic result, and they do not have the title of doctor. The primary reason the term doctor is applied to the dental profession is not a result of the art form; it is because of the dental sciences.
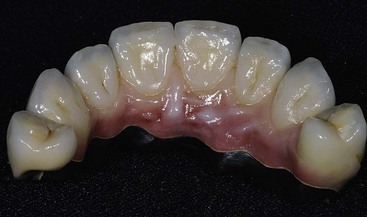
The dental sciences may be separated into a biological component and a biomechanical component. For dentists in general, the biological aspects of oral health are emphasized in our education. This makes sense because the most common complications related to the natural dentition are primarily of biological origins, with periodontal diseases, caries, and endodontic problems as examples.1–3 In fact, the doctorate we receive in dentistry is really a doctorate of biological sciences.
A combination of biological and biomechanical factors is responsible for the failure of tooth-supported fixed prostheses. For example, the four most common complications for three-unit fixed prostheses are (1) caries, (2) endodontic problems, (3) an unretained prosthesis, and (4) porcelain fracture.4,5 The biological complications occur with greater frequency (11%–22%) compared with the biomechanical (7%–10%), but both aspects should be understood by clinicians. Yet most often as dentists, we have few lectures on the biomechanics of dentistry, with the exception of orthodontics related to tooth movement.
Implant dentistry primarily involves the replacement of teeth. When implant complications are reported, the vast majority of problems are related to the implant sciences.6 But unlike natural teeth, the biological aspects of implant dentistry have fewer complications. For example, the development of a direct bone–implant interface is very predictable and largely biological. Most recent reports indicate the surgical phase of implants form a successful interface more than 95% of the time regardless of the implant system used.7–10 However, biomechanical-related problems may affect more than 30% of the implant restorations.6
Natural Tooth versus Implant Support Systems
Compared with an implant, the support system of a natural tooth is better designed to reduce the biomechanical forces distributed to the tooth and restoration and the crestal bone region. The periodontal membrane, biomechanical design, nerve and blood vessel complex, occlusal material, and surrounding type of bone blend to decrease the risk of occlusal overload to the tooth system11 (Table 8-1).
TABLE 8-1
Tooth versus Implant Support Systems
c. Implant is always rigid (mobility is failure)
d. Lateral force increases strain to bone
e. No fremitus
f. Radiographic changes at crest (bone loss; not reversible)
a. Short force duration (increased force impulse)
b. Round cross-section and designed for surgery
c. Elastic modulus five to 10 times that of cortical bone
d. Diameter related to existing bone
a. No precursor sign of slight occlusal trauma
b. Occlusal awareness of two to five times less (higher maximum bite force functional)
c. Functional bite force four times higher
4. Occlusal material porcelain (metal crown)
Periodontal Complex versus Direct Bone Interface
The presence of a periodontal membrane around natural teeth significantly reduces the amount of stress transmitted to the bone, especially at the crestal region.12 The displacement of the periodontal membrane dissipates the energy to the fibrous tissue interface (periodontal ligament) surrounding natural teeth and acts as a viscoelastic shock absorber, serving to decrease the magnitude of stress to the bone at the crest and to extend the time during which the load is dissipated (thereby decreasing the impulse of the force).13 The force transmission is so efficient and within ideal strain conditions for bone that a thin layer of cortical-like bone (cribriform plate) forms around the tooth. When the tooth is lost, the cortical plate lining disappears, demonstrating this is not an anatomic structure but is a result of an ideal strain interface to the bone.
Compared with a tooth, the direct bone interface with an implant is not as resilient. No cortical lining is present around the implant, which indicates that the forces are not dissipated ideally around the interface. Instead, the energy imparted by an occlusal force is not dissipated away from the crestal region but rather transmits a higher intensity force to the crestal contiguous bone interface.
The mobility of a natural tooth can increase with occlusal trauma. This movement dissipates stresses and strains otherwise imposed on the adjacent bone interface or the prosthetic components. After the occlusal trauma is eliminated, the tooth can return to its original condition with respect to the magnitude of movement.14 Mobility of an implant also can develop under occlusal trauma. However, after the offending element is eliminated, an implant does not return to its original rigid condition. Instead, its health is compromised, and failure of the entire implant system is usually imminent.
A lateral force on a natural tooth is dissipated rapidly away from the crest of bone toward the apex of the tooth. The healthy, natural tooth moves almost immediately 56 to 108 microns (primary tooth movement) and pivots two thirds down toward the tapered apex with a lateral load13,15 (Figure 8-4). This action minimizes crestal loads to the bone. An implant does not exhibit a primary immediate movement with a lateral load. Instead, a more delayed movement of 10 to 50 microns occurs, which is related to the viscoelastic bone movement16 (Figure 8-5). In addition, this action does not pivot (as a tooth) toward the apex but instead concentrates greater forces at the crest of surrounding bone. Therefore, if an initial lateral or angled load (e.g., premature contact) of equal magnitude and direction is placed on an implant crown and a natural tooth, the implant system (crown, cement or screw retention, abutment screw, marginal bone, implant–bone interface) sustains a higher proportion of the load that is not dissipated to the surrounding structures.
The dentist uses mobility ratings to evaluate the quality of a natural abutment. A tooth with a Miller index mobility of 0 is considered “stronger” than a tooth with mobility of 2. Implants exhibit no clinical mobility compared with teeth. Phrases such as “solid as a rock” were used originally to describe their rigid fixation. As a result, the dentist may consider the implant a stronger abutment than a tooth, especially when the literature has implied that distal cantilevers off four anterior implants can be used to restore an entire arch.17 However, when considering stress factors, mobility is an advantage. The natural tooth, with its periodontal ligament, constitutes a near perfect optimization system to handle biomechanical stress. In fact, the stress is handled so well that bacteria-related disease is the weak link. An implant system handles stress poorly (capturing the stress at the crest of the ridge) and is unable to increase mobility without failure, so biomechanical stress is the weakest link in the system.
The natural teeth may have an occlusal adjustment by using fremitus, the act of lightly placing the fingers against the buccal of the teeth as they are lightly tapped together. The implant crowns do not have fremitus as they occlude together, which makes it more difficult to adjust the occlusal contact intensity.
A radiograph of a natural tooth that has occlusal trauma will have a widened periodontal space and a thicker lamina dura (cribriform plate). A radiograph of an implant crown with occlusal trauma has no changes in the bone around the implant. However, crestal bone loss may be a consequence of the excessive load because the forces are concentrated on the marginal bone. The complete bone–implant interface may also break down, and the implant may become mobile and surrounded by fibrous tissue.
Biomechanical Design
When two bodies collide in a small interval of time (fractions of a second), large reaction forces develop. Such a collision is described as impact. When the collision is between a weight and a platform supported by a spring, the spring absorbs some of the intensity of the force (because of the deformation of the spring), and the impact force is reduced. This is similar to the collision with teeth, which are supported by a periodontal complex. When a similar force (mass and velocity) collides with a rigid platform, the intensity of the force (implant force) is greater. This is similar to a collision with an implant system, which has a direct implant–bone interface11 (Figure 8-6).
An implant system receives a greater impact force than a natural tooth because it is not surrounded by a periodontal complex. The fact that the implant is more rigid actually means that the implant system receives greater force and is more at biomechanical risk than a natural tooth. The implant system includes the occlusal porcelain on the crown (which may fracture), the cement or screw that retains the prosthesis (which may debond or loosen), the abutment screw that contains the components (which may loosen), the crestal marginal bone (which may be lost from pathologic overload), the complete implant–bone interface (which may result in mobility and failure), and the implant and prosthetic components (which may result in fracture) (Box 8-1).
An analogy of the difference in impact force between an implant and a tooth is hitting a nail with a steel hammer (a rigid structure) compared with a rubber hammer (a mobile structure). The more rigid hammer transmits a higher intensity force and drives the nail farther into the wood rather than having the energy partially dissipated through deflection of the rubber hammer.
The width of almost every natural tooth is greater than the width of the implant used to replace the tooth. The greater the width of a transosteal structure (tooth or implant), the lesser the magnitude of stress transmitted to the surrounding bone.11 The anterior teeth are narrower than the posterior teeth because the forces are greater in the posterior regions. An implant width is most often decided by the available bone width and is more narrow than a natural tooth in the region. In addition, the implant width is most often the same in all regions of the mouth.
The cross-sectional shape of the natural tooth at the crest is biomechanically optimized to resist lateral (buccolingual) loads because of the bending fracture resistance (moment of inertia) of the tooth and the direction of occlusal forces. Hence, mandibular anterior teeth are greater in size in the faciolingual directions (to resist protrusive forces), and canines have different cross-sections than other anterior teeth and sustain lateral loads in more directions. In contrast, implants are round in cross-section, which is less effective in resisting lateral bending loads and consequently increase stress concentration in the crestal region of the jaws.
The design of the natural tooth root and surface area is related to amount of biomechanical stress. Molars have greater dimensions than premolars (greatest bite forces in molar region), and the maxillary molars have greater surface area than the mandibular counterparts to compensate for the difference in surrounding bone. The length and design of the implant often is decided by the existing bone volume rather than the amount and direction of force. Hence, the greatest-sized (surface area) implants are often inserted into the anterior mandible followed by the anterior maxilla and the posterior mandible, and the smallest-length implants are often inserted into the posterior maxilla. The design of the implant is most often the same regardless of its length or width.
The elastic modulus of a tooth is closer to bone than any of the currently available dental implant biomaterials. Titanium is more than 10 to 20 times more stiff than cortical or trabecular bone. The greater the flexibility (modulus) difference between two materials (metal and bone or tooth and bone), the greater the potential relative motion generated between the two surfaces upon loading.11 In addition, the greater the elastic modulus difference, the greater the stress concentrations where they first meet (the crest of the ridge). Hence, under similar mechanical loading conditions, implants generate greater stresses and strains to the overall bone and at the crest of bone compared with a natural tooth.18
Sensory Nerve Complex versus Direct Bone Interface
The precursor signs of a premature contact or occlusal trauma on natural teeth are usually reversible and include hyperemia and occlusal or cold sensitivity.14 This condition often results in the patient’s seeking professional treatment to reduce the sensitivity, and usually this condition is treated by an occlusal adjustment and a reduction in force magnitude, which concomitantly decreases stress magnitude. This treatment most often reduces the hyperemia and the symptoms associated with this condition. If the patient does not have an occlusal adjustment, the tooth often further increases in mobility to dissipate the occlusal forces. If the patient still fails to seek professional treatment for the increased mobility, the tooth may migrate orthodontically away from the cause of the occlusal stress. Hence, the early warning signs and symptoms of excessive biomechanical load on natural teeth are often reversible or decreased as a result of mobility and tooth movement.
The initial reversible signs and symptoms of trauma on natural teeth do not occur with endosteal implants. An absence of soft tissue interface between the implant body and bone results in the greatest portion of the force being concentrated around the transosteal implant–bone region.11 The magnitude of stress may cause bone microfractures; place the surrounding bone in the pathologic loading zone, causing bone loss19; or cause mechanical failure of prosthetic or implant components (e.g., porcelain fracture, abutment screw loosening).6
Unlike the reversible signs and symptoms exhibited by natural teeth, implant bone loss or unsecured restorations most often occur without any warning signs. Abutment screw loosening most often is a symptom of biomechanical stress beyond limits of the system. Marginal bone loss around the implant occurs without symptoms. The loss of crestal bone around the implant is not reversible without surgical intervention and results in a decreased implant support and increased sulcus depth around the abutment. As a result, unless the density of bone increases after loading or the amount or duration of force decreases, the bone loss condition may progress until implant loss because the implants cannot move orthodontically away from the offending force.
The natural teeth and their periodontal ligament provide proprioception and early detection of occlusal loads and interferences. As a result, an occlusal premature contact greater than 20 microns may alter the path of mandibular closure to decrease the noxious elements of the premature, angled force.20 In addition, the jaw of a dentate patient almost stops before the food is penetrated and the maximum chewing force is applied. This is why a piece of bone in meat may shear off a cusp tip because the jaws did not reduce their velocity before the contact with the piece of bone. Implant prostheses do not have as much occlusal awareness as teeth during function. As a result, the bite forces used in mastication or parafunction can be of greater magnitude, and the path of closure is not altered with a premature contact.
Several studies confirm that implant prostheses have less occlusal awareness and higher bite forces than natural teeth. Trulsson and Gunne compared three patient groups holding a peanut between the teeth for 3 seconds and then biting through the peanut.21 The natural teeth group had no problem holding the peanut or biting through it afterward. The denture group of patients experienced greater problems holding the peanut without its falling or becoming dislodged. The implant group had no problem holding the peanut in place, but they bit through the peanut with a force fourfold greater than the natural dentition group. The four times higher force in the implant patients is generated to the implant system, not the soft tissue of the denture group or the periodontal complex of the natural dentition. Hence, the decrease in proprioception of implant patients can lead to a higher bite force during functional loading.
Other studies confirm that teeth have more occlusal awareness than implant prostheses. Jacobs and van Steenberghe22,23 evaluated occlusal awareness by the perception of an interference. When teeth oppose each other, an interference is perceived at approximately 20 microns. An implant opposing a natural tooth detects an interference at 48 microns, which is therefore more than twice as poor. An implant crown opposing an implant crown perceives the interference at 64 microns, and when a tooth opposes an implant overdenture, the awareness is 108 microns (five times poorer than teeth opposing each other). Mericke-Stern et al. measured oral tactile sensitivity with steel foils.24 The detection threshold of minimal pressure was significantly higher on implants than on natural teeth (3.2 vs. 2.6 foils). Similar findings also were reported by Hammerle et al. in which the mean threshold value for implants (100.6 g) was 8.75 times higher than that of natural teeth (11.5 g).25 As a consequence of decreased quantity and quality of occlusal awareness, a premature contact does not trigger an adaptation response (Figure 8-7).
The proprioceptive information relayed by teeth and implants also differs in quality awareness. Teeth deliver a rapid, sharp pain sensation under high pressure that triggers a protective mechanism. However, implants deliver a slow, dull pain that triggers a delayed reaction, if any.26 Implant occlusal sensitivity is uncommon and signifies more advanced complications.
Occlusal Material
The natural tooth can show clinical signs of increased stress such as enamel wear facets, stress lines, lines of Luder (in amalgam fillings), cervical abfraction, and pits on the cusps of teeth.20 An implant crown rarely shows clinical signs other than fatigue fracture. As a result, fewer diagnostic signs are present to warn the practitioner to reduce the stress on the support system. The enamel on a tooth will wear when repeated lateral forces or premature contacts are introduced on the system and may reduce the angle of force for the premature contact. A porcelain occlusal implant crown does not wear and reduce the noxious force when a lateral premature contact is present.
Surrounding Bone
The tooth slowly erupts into occlusion and is present in the mouth from childhood. The surrounding bone has developed in response to the biomechanical loads. Note that there is no organized cribriform plate around the deciduous or permanent tooth until it is loaded. The permanent teeth are gradually introduced a few at a time while other teeth are present and bear the load. Hence, periodontal tissues organize gradually to sustain increasing loads, including those brought to bear by an attached prosthesis. The only progressive bone loading around an implant is performed by the restoring dentist in a much more rapid time frame and intense magnitude of load.
When implants or teeth are subjected to repeated occlusal loads, microscopic stress fractures, work hardening, and fatigue may result. Fatigue fractures are related to the amount of stress and the number of cycles of load.11 Unlike the natural tooth system, the implant components, coping screws, or cement cannot adjust or repair to these conditions and ultimately fracture. The implant needs to perform its service for scores of years, increasing the cycles in the fatigue curve and the risk of long-term complications. As a result, forces from occlusion may result in subtle changes but may cause more serious long-term problems for survival as a result of bone or implant component complications compared with natural teeth (Table 8-2).
TABLE 8-2
Natural Tooth versus Implant Characteristics Under Load
Criterion | Tooth | Implant |
Connection | PDL | Functional ankylosis |
Impact force | Decreased | Increased |
Mobility | Variable Anterior teeth more than posterior teeth |
None |
Movement Apical Lateral |
Shock breaker effect of PDL Intrude quickly 28 µm 56–108 µm |
Stress captured at crest No initial movement (3 µm) No initial movement |
Diameter | Large | Small |
Cross-section | Not round | Round |
Modulus of elasticity | Similar to cortical bone | 10–20 times greater than trabecular bone |
Signs of hyperemia | Yes | No |
Orthodontic movement | Yes | No |
Fremitus | Yes | No |
Radiographic changes | PDL and cortical bone thickening | Crestal bone loss |
Progressive loading | Eruption sequence (crestal bone loss) | Shorter loading period |
Wear | Enamel wear facets, localized fatigue and stress fracture, cervical abfraction, and pitting on occlusal cusps | Minimal wear |
Tactile sensitivity | High | Low |
Occlusal awareness (proprioception) | High detection of premature contacts | Low; higher loads to premature occlusal |
PDL, Periodontal ligament.
Biomechanical parameters are excellent indicators of the increased risk because they are objective and can be measured. As a result, ways to decrease biomechanical stress are a constant concern to minimize the risk of implant system complications.18 The dentist can determine which condition presents greater risk and by how much the risk is increased. In other words, if a clinical condition creates an increased biomechanical stress to the implant–prosthetic system, the dentist should implement mechanisms to decrease the stress. Remember, the implant system is composed of the occlusal porcelain, the cement or screw that retains the restoration, the abutment screw that retains the implant components, the crestal marginal bone around the implant, the complete bone–implant interface, and the implant bodies themselves. Higher stresses may lead to complications in any aspect of this system18 (Box 8-2).
Any complex engineering structure will fail at its “weakest link,” and dental implant structures are no exception. A general concept in engineering is to determine the causes of complications and develop a system to reduce the conditions that cause the problems. Natural teeth most often have biological complications. Implants may also have biological problems. However, unlike natural teeth, the most common causes of implant-related complications are centered around biomechanical stress (Box 8-3). Thus, the overall treatment plan should (1) assess the greatest force factors in the system and (2) establish mechanisms to protect the overall implant–bone–prosthetic system.
Failure of Natural Tooth–Supported Prostheses
The most common failures of a fixed prosthesis supported by natural teeth are intermediate- to long-term failure and are often related to biological factors. For example, a three-unit fixed partial denture replacing one posterior tooth has a 5-year survival rate above 95%. The 10-year failure rate is approximately 30%, and the 15-year failure rate is almost 50%. The primary cause of natural tooth–supported prostheses is caries of the abutment teeth. Hence, failures are after many years and primarily related to bacteria plaque as a result of inadequate patient oral hygiene (biological related failure).
Early Loading Failure
The surgical and initial healing phase for implants is primarily related to the biological aspects of healing and is very predictable. The implant prosthesis may have a higher intermediate- to long-term survival rate compared with natural teeth because implants do not decay and do not require endodontic treatment7–10 (Figure 8-8). Although the initial healing of the implant has very high success rates, an implant may fail shortly after it is loaded with the prosthesis. Before failure, the implant appears to have rigid fixation, and all clinical indicators are within normal limits. However, after the implant is loaded, the implant becomes mobile, most often within the first 18 months (Figure 8-9). This has been called early loading failure.27 Hence, the implant and prostheses may fail early, but a natural tooth restoration has more of a long-term failure rate. The primary cause of early loading failure is related to biomechanical factors for the bone–implant interface, and this failure may approach rates more than five times greater than initial healing rates. The causes of early loading failure are important to understand, so a method may be established to limit this complication (Figure 8-10).
Cellular Biomechanics
Bone remodeling at the cellular level is controlled by the mechanical environment of strain.28 Strain is defined as the change in length divided by the original length, and the units of strain are given in percentages. The amount of strain in a material is directly related to the amount of stress applied.11 Occlusal stress applied through the implant prosthesis and components can transmit stress to the bone–implant interface.18 The amount of bone strain at the bone–implant interface is directly related to the amount of stress applied through the implant prosthesis (the greater the stress, the greater the strain). Mechanosensors in bone respond to minimal amounts of strain, and microstrain levels 100 times less than the ultimate strength of bone may trigger bone remodeling, which may result in bone loss29 (Figure 8-11).
One of the earliest remodeling theories for a direct relationship between stress and the magnitude of bone remodeling (including loss) was proposed by Kummer in 1972.30 More recently, Frost reported on the cellular reaction of bone to different microstrain levels.19,31 When bone does not have enough strain, it resorbs (acute disuse window). When bone has an ideal strain, it remains organized, mineralized, and the ideal load-bearing bone (adapted window). Bone fractures at 10,000 to 20,000 microstrain units (1%–2% deformation). However, at levels 20% to 40% of this value (4000 units), bone cells may trigger cytokines to begin a bone resorption response (pathologic overload window) (Figure 8-12). It is interesting to note that cytokines in the bone–implant interface tissue obtained from failed hip replacement devices leading to bone loss have been reported in humans.32 It is logical that a similar condition may exist around a dental implant. In other words, excessive bone strain may not only result in physical fracture but may also cause bone cellular resorption.
Strain determines the cellular reaction of bone. When bone has ideal strain, the bone remains organized and load bearing. When the strain is greater, it may be in a pathologic overload zone, which causes bone loss. Therefore, the hypothesis that occlusal stresses beyond the physiologic limits of bone may result in strain in the bone significant enough to cause bone resorption is supported from a cellular biomechanics standpoint.
Engineering Principles
The relationship between stress and strain determines the modulus of elasticity (stiffness) of a material.11 The modulus conveys the amount of dimensional change in a material for a given stress level. The modulus of elasticity of a tooth is similar to cortical bone. Dental implants are typically fabricated from titanium or its alloy. The modulus of elasticity of titanium is five to 10 times greater than that of cortical bone and may be 20 times greater for trabecular bone. Hence, when 50 units of stress is applied to titanium, the strain (change in shape) of the material is less than when applied to bone (Figure 8-13). The biomechanical strain difference between titanium and bone with a direct implant–bone interface may be in the pathologic overload zone for bone with greater stress magnitudes. When the stress magnitude is reduced, the biomechanical difference between titanium and bone is less and more likely in the adapted window zone for bone (Figure 8-14).
When the microstrain of the bone is more ideal, the adapted window strain zone may allow the bone to remain integrated to the implant (Figure 8-15). Isidor allowed eight implants to integrate in monkey jaws.33,34 Crowns were attached to eight of the integrated implants with excessive premature occlusal contacts. Over a 14-month period, six of eight implants failed (Figure 8-16). In these same animals, eight integrated implants with no occlusal loads had strings placed in the marginal gingiva to increase the amount of plaque retention. None of these implants failed over the following 18 months. In this animal model, biomechanical occlusal stress was a greater risk factor for early implant failure than the biological component of bacterial plaque (Figure 8-17).
Early loading failure is most often related to the amount of force applied to the prosthesis35 or the density of the bone around the implants, both biomechanical factors.36,37 For example, a literature review found these early implant loading failures may occur 16% of the time in the softest bone types or at similar rates when the implants are less than 10 mm long.6 These two failure groups are typically caused by biomechanical factors. Soft bone is too weak for the occlusal forces applied to the implants, and short implants have less surface area and higher stresses at the bone–implant interface.38,39 No reports in the literature correlate such high incidence extreme with early implant failure rates related to biological complications. It should be noted that biomechanical-related causes of failure are more often influenced by the dentist than biological causes of failure (Figure 8-18).
Early loading failure is worse for the implant clinician than when a surgical failure occurs because the patient may blame the restoring dentist. Although this is bad enough, in addition, the restoring dentist spent two to five appointments restoring the implant and has a laboratory expense. The implant surgery may require a bone graft as a consequence of the failure. A bone graft is often less predictable than the integration of an implant in adequate bone. Hence, the overall treatment is often more difficult than the original reconstruction. This is not an ideal scenario because it occurs in a patient who already has experienced a failure.
Occlusal Overload on Prosthetic Components
Fatigue Fractures and Complications
The most common source of biomechanical stress to an implant system occurs during occlusal function. Most biomechanical complications do not occur as a result of a single force event, such as a car accident. Instead, they occur over time. Materials follow a fatigue curve, which is related to the number of cycles and the intensity of the force.11 There is a force so great that one cycle causes a fracture (e.g., a hammer hitting a glass window). However, if a lower force magnitude repeatedly hits an object (and is greater than the endurance limit), it will still fracture (Figure 8-19). A wire coat hanger that is bent does not break the first time, but repeated bends will fracture the material—not because the last bend was more forceful but because of fatigue. Indeed, when a patient says he soaked his bread in coffee before he began to chew it and the porcelain–abutment screw–cement seal–cantilevered prostheses fractured, it may have been “the straw that broke the camel’s back” that caused the problem. The most common complications of implants and prostheses are related to biomechanical conditions related to fatigue.6
Prostheses and Component Fractures
In a 2009 retrospective analysis by Kinsel and Lin of porcelain failures of metal ceramic crowns and fixed partial dentures supported by implants, porcelain fractures ranged from 0% to 53% of patients and were directly related to force factors40 (Figure 8-20). For example, whereas 35% of patients with bruxism (and 19% of the implant crowns) experienced porcelain fracture with implant-supported prostheses, 17% of patients without bruxism had at least one porcelain fracture of porcelain units fractured. When implant prostheses opposed a denture, no fracture was observed. When implant prostheses opposed each other, 16% of the dental units experienced a fracture of porcelain. The higher forces in the implant system (including the occlusal porcelain) were related to a dramatic increase in biomechanical complications. Note that the incidence of porcelain fracture, even in patients without higher force conditions, are greater than observed with natural teeth.
Implant overdentures have problems of attachment fracture or complication (30%); removable prostheses may fracture (12%); and in implant-supported fixed prostheses, acrylic resin veneers may fracture (22%). Metal framework fractures also have been reported in an average of 3% of fixed complete prostheses and overdenture restorations may fracture with a range of 0% to 27%.6
Prosthesis screw fracture has also been noted in both fixed partial and complete fixed prostheses, with a mean incidence of 4% and a range of 0% to 19%.6 Abutment screws are usually larger in diameter and therefore fracture less often, with a mean incidence of 2% and a range of 0.2% to 8% (Figure 8-21). Implant body fracture has the least incidence of this type of complication, with an occurrence of 1%. This condition is reported with more frequency in long-term fixed prostheses. For example, in a 15-year report, implant body fracture was the most common condition that led to failure of the implant system41 (Figure 8-22).
Uncemented prostheses are the third most common cause of fixed prostheses failure on natural teeth.4,5 This condition is more common with implant abutments because they are more rigid and higher forces are transmitted to the cement interface. Uncemented restorations (or worse, when one or more crowns become uncemented and some abutments are still retained) occur most likely when chronic loads are applied to the cement interface or when shear forces are present (as found with cantilevers). Cement strengths are weakest to shear loads. Zinc phosphate cement may resist a compressive force of 12,000 psi but can only resist a shear force of 500 psi. A shear load is applied to the cement when a cantilever is present.
Screw Loosening
Abutment screw loosening has been detected in an overall average of 6% of implant prostheses.6 Single-tooth crowns exhibited the highest rate of abutment screw loosening and in early screw designs and concepts averaged 25%. Recent studies indicate this ratio has been reduced in single crowns to an overall 8% average, with multiple-unit fixed prostheses at a 5% average and implant overdentures at 3%. Screw loosening may cause considerable complications. A loose screw may contribute to crestal bone loss because bacteria are able to harbor in the open interface. When an abutment screw becomes loose on a cemented crown, the crown may need to be cut off the abutment to gain access to the abutment screw (Figure 8-23).
The greater the stress applied to the prostheses (single tooth vs. overdentures), the greater the risk of abutment screw loosening. Cantilevers increase the risk of screw loosening because they increase the forces to the implant system in direct relationship to the length of the cantilever.42 The greater the crown height attached to the abutment, the greater the force applied to the screw and the greater the risk of screw loosening (or fracture)43 (Figure 8-24).
The height or depth of an antirotational component of the implant body also can affect the amount of the force applied to the abutment screw. The higher (or deeper) the hex height, the less stress applied to the screw and a corresponding lower risk for abutment screw loosening.43 The platform dimension upon which the abutment is seated is even more important than the hex height dimension. Larger-diameter implants, with larger platform dimensions, reduce the forces applied to an abutment screw and change the arc of displacement of the abutment on the crest module.43 For example, in a report by Cho et al., abutment screw loosening over a 3-year period was almost 15% for the 4-mm implant diameter but less than 6% for the 5-mm implant diameter.44 Therefore, methods to decrease stress to the abutment screw may be used to decrease the incidence of complications related to screw loosening.
As a consequence of these biomechanical complications, the evaluation, diagnosis, and modification of treatment plans related to stress conditions are of considerable importance. Therefore, after the implant dentist has identified the sources of additional force on the implant system, the treatment plan is altered in an attempt to minimize their negative impact on the longevity of the implant, bone, and final restoration.
Marginal Bone Loss
Crestal bone loss has been observed around the permucosal portion of dental implants for decades. It has been described after exposure and loading of successfully osseointegrated implants regardless of surgical approaches. It can range from loss of marginal bone to complete failure of the implant.45,46
Adell et al. were the first to quantify and report the marginal bone loss around machined screw–type implants (Nobel Biocare).17 The report also indicated greater magnitude and occurrence of bone loss during the first year of prosthesis loading, averaging 1.2 mm during this time frame, with a range of 0 to 3 mm. (Implants with more than 3 mm of bone loss were not included in the report.) This report measured bone loss from the first thread as the 0-mm baseline, not from the original level of crestal bone at insertion, which was 1.8 mm above this baseline point. Thus, the actual first-year crestal bone loss averaged 3.3 mm around the implants observed (Figure 8-25).
The initial transosteal bone loss around an implant forms a V– or a U-shaped pattern, which has been described as ditching or saucerization around the implant.47 The current hypotheses for the causes of crestal bone loss have included biological causes of reflection of the periosteum during surgery, preparation of the implant osteotomy, the position of the microgap between the abutment and implant body, bacterial invasion, and the establishment of a biological width. Biomechanical factors may also contribute to crestal bone loss and include micromovement of the abutment components and factors of biomechanical stress48–50 (Box 8-4).
Marginal crestal bone loss may influence esthetics because the height of the soft tissue (e.g., free gingival margin and interdental papilla) is directly related to the marginal bone. If the tissue shrinks as a consequence of the bone loss, the emergence profile of the crown elongates, and the papilla may disappear next to the adjacent tooth or implant (Figure 8-26). If the soft tissue does not shrink, the increase in pocket depth may be related to the presence of anaerobic bacteria and periimplantitis and cause additional bone loss or exudate.
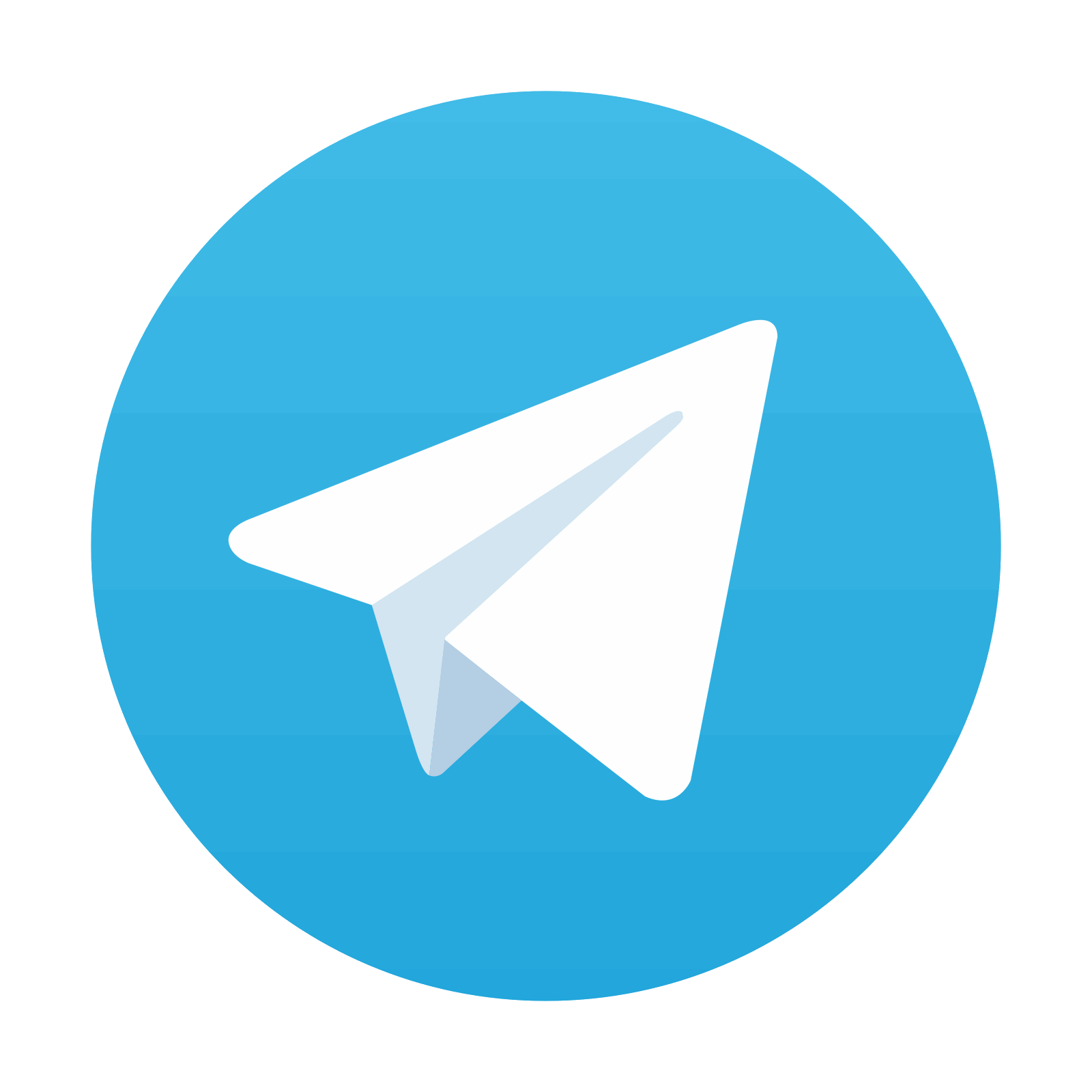
Stay updated, free dental videos. Join our Telegram channel

VIDEdental - Online dental courses
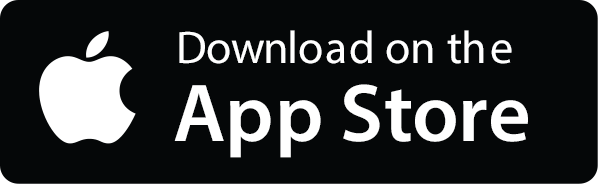
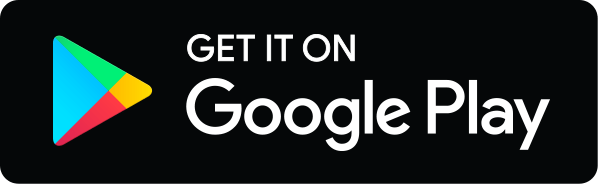