Genetics
After studying this chapter, the student will be able to:
1. Define each of the words listed in the vocabulary for this chapter.
2. State the purpose of mitosis.
3. State the purpose of meiosis.
4. Explain what is meant by the Lyon hypothesis and give an example of its clinical significance.
5. Explain what is meant by a gross chromosomal abnormality and give three examples of syndromes that result from gross chromosomal abnormalities.
6. List the four inheritance patterns described in this chapter.
7. Explain what is meant by X-linked inheritance.
8. State the inheritance pattern and describe the oral manifestations and, if appropriate, the characteristic facies for each of the following: cyclic neutropenia, Papillon-Lefèvre syndrome, cherubism, Ellis–van Creveld syndrome (chondroectodermal dysplasia), mandibulofacial dysostosis (Treacher Collins syndrome), osteogenesis imperfecta, hereditary hemorrhagic telangiectasia (Osler-Rendu–Parkes Weber syndrome), Peutz-Jeghers syndrome, white sponge nevus (Cannon disease), hypohidrotic ectodermal dysplasia, hypophosphatasia, and hypophosphatemic vitamin D–resistant rickets.
9. State the inheritance pattern, the oral or facial manifestations, and the type and location of the malignancy associated with each of the following syndromes: Gardner syndrome; nevoid basal cell carcinoma syndrome (Gorlin syndrome); multiple mucosal neuromas, medullary carcinoma of the thyroid gland, and pheochromocytoma syndrome (multiple endocrine neoplasia type 2B [MEN 2B]); and neurofibromatosis of von Recklinghausen.
10. State the location and malignant potential of the intestinal polyps in Peutz-Jeghers syndrome and Gardner syndrome.
11. List the four types of amelogenesis imperfecta.
12. Briefly compare and contrast dentinogenesis imperfecta, amelogenesis imperfecta, and dentin dysplasia, including the inheritance patterns, the clinical manifestations, and the radiographic appearance of each.
General term used to refer to the material (deoxyribonucleic acid [DNA]) that forms the chromosomes.
Chromosomes
The hereditary units that are transmitted from one generation to another are called genes. They are found on chromosomes, which are located in the nucleus of the cell. Using a microscope, one can see chromosomes clearly only when the nucleus and cell are dividing (Figure 6-1). At other times the genetic material is dispersed in the nucleus (see Figure 6-1). Each cell of the human body, with the exception of mature germ cells (ova and spermatozoa), has 46 chromosomes. Half of these chromosomes are derived from the father, and the other half from the mother.
Normal Cell Division
Mitosis
All cells in the body, with the exception of ova and spermatozoa, are called somatic cells. Cellular division is achieved by mitosis during a part of the life span of the somatic cell, called the mitotic cycle. The function of mitosis is to create an exact copy of each chromosome and, through division of the original cell, distribute an identical set of chromosomes to each daughter cell. After each cell division is completed and before the next division can occur, the cell enters the gap 1 (G1) phase, which is followed by the S phase, in which replication of the DNA takes place. The gap 2 (G2) phase follows the S phase and ends when mitotic division begins. The cell cycle is illustrated in Figure 6-2.
Stages of Mitosis
Mitosis is composed of four stages: (1) prophase, (2) metaphase, (3) anaphase, and (4) telophase. In each of these four stages, the chromosomes are distributed in a specific arrangement. In metaphase the chromosomes stain intensely and are arranged almost symmetrically at both sides of the center, or equatorial plane, of the cell. The appearance of a metaphase chromosome resembles the letter X (Figure 6-3), having a pair of “long arms” also known as q arms (see Figure 6-3, 3) and a pair of “short arms” also known as p arms (see Figure 6-3, 1). The size of the chromosome at metaphase and the length of the long and short arms vary from chromosome to chromosome. The constriction present in all chromosomes, which joins the short and long arms, is called the centromere (see Figure 6-3, 2). During metaphase chromosomes are actually formed by two identical vertical halves, each composed of either left or right short and long arms and half of the centromere. Each of these identical halves is called a chromatid (see Figure 6-3, 4). At metaphase each chromatid contains one molecule of DNA; therefore the DNA content of each chromosome is doubled (Figure 6-4). When cell division takes place, each chromosome splits vertically at the centromere; 46 chromatids (which now become chromosomes) form one daughter cell, and the other 46 chromatids form a second daughter cell. During prophase the chromosomes are lining up toward metaphase; in anaphase and telophase the chromatids are in the process of splitting.
Meiosis
First Meiosis
Before the first meiosis in the primitive germ cells, a replication of DNA occurs that is similar to that observed in the S phase of somatic cells. After replication the members of each pair of chromosomes line up next to each other in an intimate point-by-point relationship (Figure 6-5, A). This pairing does not occur in mitosis. After pairing, the two chromosomes establish actual contact at different locations. These contacts are known as chiasmata (meaning X shaped) and determine points of crossing over (Figure 6-5, B). This crossing over achieves the exchange of chromosome segments between a chromatid of one chromosome and a chromatid of the other chromosome of a pair (between homologous chromosomes) (Figure 6-5, C). This special aspect of the first meiosis takes place at metaphase. After metaphase of the first meiotic division, the chromosomes separate from each other, but no splitting of the centromere occurs. The chromosomes remain intact, and each member of the pair migrates to one of the new cells, each of which contains 23 chromosomes but twice the final amount of DNA. During this migration chromosomes of the paternal and maternal lines segregate at random, thus ensuring diversity of the species by creating a new combination of chromosomes.
Molecular Composition of Chromosomes
Deoxyribonucleic Acid
Chromosomes contain deoxyribonucleic acid (DNA). DNA contains the basic code or template that carries all genetic information. The basic unit of DNA is called a nucleotide, which is formed by a nitrogen-containing base, a five-carbon sugar (deoxyribose), and a phosphate. Four bases are found in DNA: adenine (A), guanine (G), thymine (T), and cytosine (C). These chains of polynucleotides are coiled to form a structure called a double helix (Figure 6-8). In DNA the base adenine is always bound to the base thymine, and guanine is always bound to cytosine. This is a consistent arrangement and is identical in all species from bacteria to humans, with just a few exceptions. Therefore the ratio of adenine to thymine (A/T) is always equal, and the same is true for the ratio of guanine to cytosine (G/C). In humans G/C pairs are about four times more frequent than A/T pairs. In Figure 6-8, the polynucleotide chains run vertically in opposite directions. Therefore a sequence of adenine, guanine, and cytosine (AGC) is always matched by the opposing sequence of thymine, cytosine, and guanine (TCG).
In Figure 6-8, the horizontal steps of the polynucleotide chains are nucleotides. Each pair of nucleotides is joined by a hydrogen bond, indicated by the dotted lines This arrangement is repeated horizontally to form the polynucleotide double spiral staircase (or helix) appearance of DNA (see Figure 6-8).
Ribonucleic Acid
Types of Ribonucleic Acid
In the production of a protein (Figure 6-9) the mRNA carries the genetic code for the formation of that protein to the ribosomes. The tRNA brings amino acids to the ribosomes from the cellular cytoplasm. The amino acid sequence forms proteins according to the genetic code, and these proteins exit the ribosomes as they are formed.
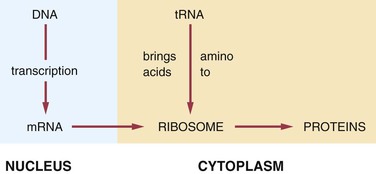
Genes and Chromosomes
Chromosomal Abnormalities
Gross chromosomal alterations can be observed in a karyotype. A karyotype (Figures 6-10 and 6-11) is a photographic representation of a person’s chromosomal constitution. Clinicians can create a karyotype by culturing cells from blood, skin, or other tissues. One method uses peripheral blood by placing it in a test tube with heparin to avoid coagulation and centrifuging it. After centrifugation the white blood cells (leukocytes) are deposited at the bottom of the tube. The leukocytes are removed and placed in a culture medium containing phytohemagglutinin, which is a substance that enhances mitosis. Because chromosomes are best observed when cell division is arrested at metaphase, colchicine is added to the culture after 72 hours of culture at 37° C to stop mitosis at metaphase. This also prevents the centromere from dividing. A hypotonic solution is then added to the culture to make the cells swell. The cells are then fixed (preserved) and stained and observed under the microscope. Examples of well-defined mitoses are chosen and photographed. The photograph is enlarged, and each chromosome is cut out of the photographic print. When stained, the chromosomes have a bandlike appearance that allows an accurate identification of each. These cutouts are then pasted on a special chart to construct the karyotype.
Gross Chromosomal Abnormalities
Alterations in Number and Structure of Chromosomes
• Euploid: A complete second set of chromosomes, the total number being 92. This is incompatible with life
• Polyploid: Three (triploid) or four (tetraploid) complete sets of chromosomes. This has been described occasionally in humans and is incompatible with life
• Aneuploid: Any extra number of chromosomes that do not represent an exact multiple of the total chromosome complement (e.g., trisomy [a pair with an identical extra chromosome] and monosomy [a missing chromosome from a pair])
Examples of structural abnormalities include the following:
• Deletion: The loss of part of a chromosome
• Translocation: A portion of a chromosome is attached to another chromosome
• Inversion: A portion of a chromosome is upside-down
• Duplication: A chromosome is larger than normal; the extra segment is identical to a segment of the normal chromosome
Clinical Syndromes Resulting From Gross Chromosomal Abnormalities
Trisomy 13
Trisomy 13 is characterized by multiple abnormalities in various organs. Seventy percent of live-born infants die within the first 7 months of life. Characteristic clinical findings include bilateral cleft lip and palate, microphthalmia (small eyes) or anophthalmia (no eyes), superficial hemangioma of the forehead or nape of the neck, growth retardation, severe mental handicap, polydactyly of hands and feet (supernumerary digits), clenching of the fist with the thumb under the fingers, rocker-bottom feet, heart malformations, and several anomalies of the external genitals. The facial appearance is quite striking because of the cleft lip, cleft palate, and ocular abnormalities (Figure 6-12).
Turner Syndrome
Patients with Turner syndrome have a female phenotype, and in the majority of cases the karyotype has the normal 44 autosomal chromosomes and only one X chromosome. A normal female would have two X chromosomes: one from the mother and one from the father. Most cases of Turner syndrome are the result of nondisjunction of the X chromosome in the paternal gamete. Clinically, these women are of short stature and have webbing of the neck and edema of the hands and feet (Figure 6-13). They frequently exhibit a low hairline on the nape of the neck. The chest is broad with wide-spaced nipples. The aorta frequently is abnormal, and body hair is sparse. The external genitals appear infantile, and generally the ovaries are not developed; therefore these individuals have primary amenorrhea (abnormal temporary or permanent cessation of the menstrual cycle). Smears taken from the oral mucosa demonstrate the lack of Barr bodies.
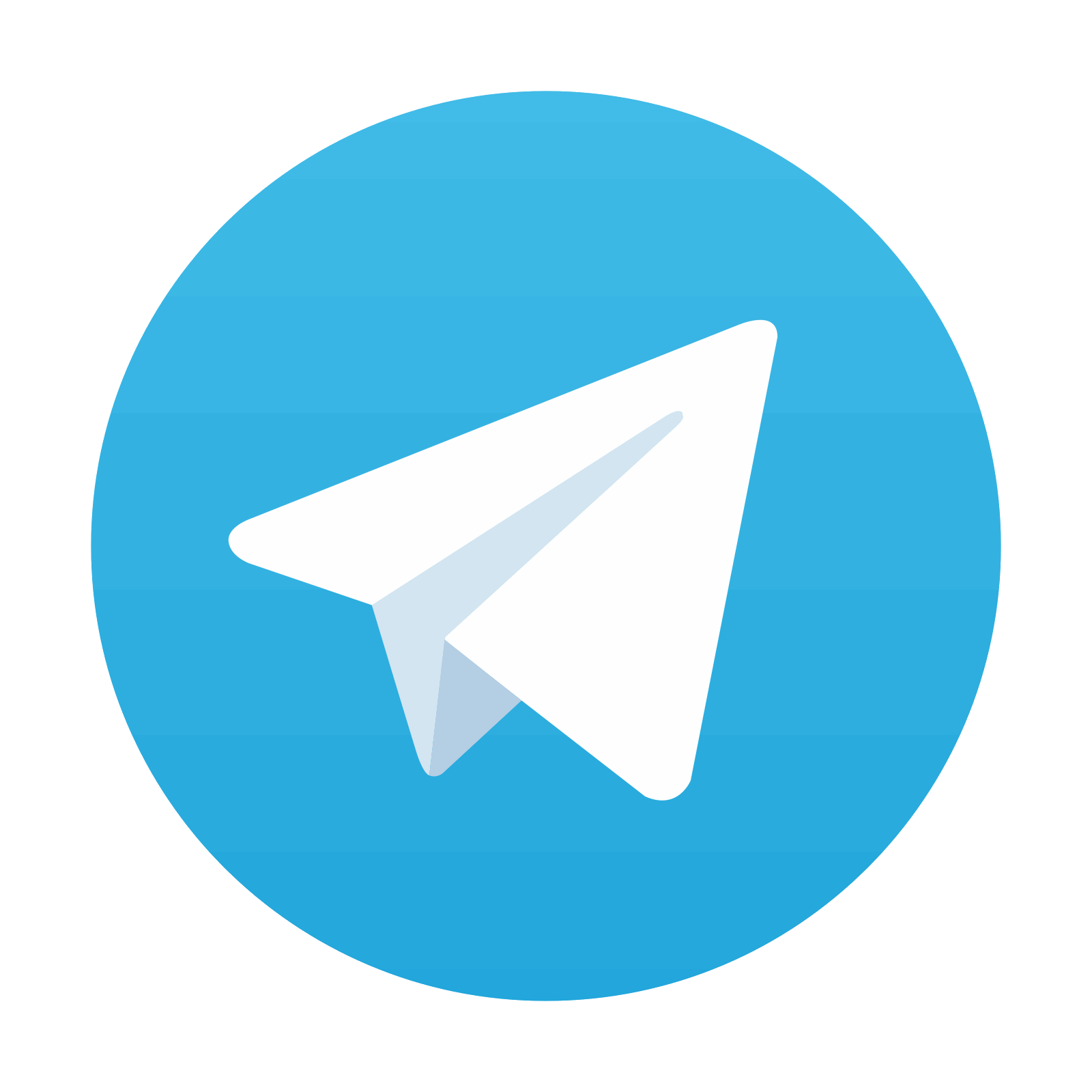
Stay updated, free dental videos. Join our Telegram channel

VIDEdental - Online dental courses
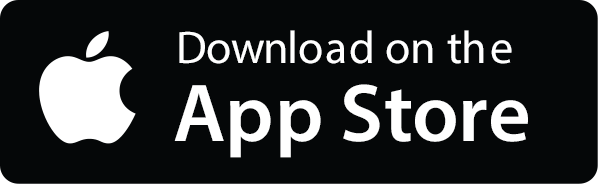
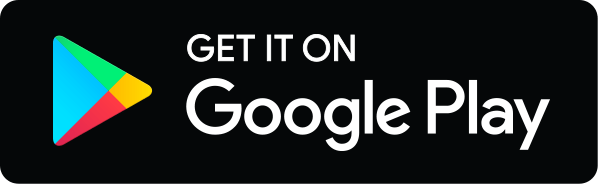