5 Regenerative Laser Periodontal Therapy
The increased inflammatory response to plaque accumulation in susceptible hosts increases overgrowth of opportunistic microbiota.1 Furthermore, environmental and systemic risk factors, such as smoking and diabetes, can worsen the expression of periodontal disease.2 Smokers and diabetic patients may respond poorly to conventional periodontal treatment. Therefore, clinicians have been in search of new techniques to treat advanced periodontal diseases in individuals with risk factors who may respond poorly to periodontal therapy.
Objectives of Periodontal Treatment
The immediate goal of periodontal treatment is to prevent, arrest, control, or eliminate periodontal disease. The ideal goal would be to promote healing through regeneration of lost form, function, esthetics, and comfort. When the ideal cannot be achieved, the pragmatic goal of therapy would be to repair the damage resulting from disease. The ultimate goal of therapy is to sustain the masticatory apparatus, especially teeth, or their analogs, in a state of health.3
The clinical objectives of periodontal treatment therefore include the following:
According to Haffajee et al.,4 the elimination of a residual true pocket of 6 mm or greater in probing depth after active periodontal treatment is an important goal of periodontal therapy and aims at arresting further disease progression. Also, it is known that deep periodontal pockets remaining after treatment play a significant role in predicting future periodontal destruction.5 Claffey et al.6 suggest that advanced periodontitis patients with multiple residual probing depths (≥6 mm at reevaluation) have a greater risk of developing sites with additional attachment loss than patients with few such residual pocket depths.
On a biological/histological level, the objective of periodontal treatment is to slow down epithelial migration from the gingival margin into the periodontal pocket to promote new connective tissue attachment (CTA) instead of a long junctional epithelium.7
In an animal study, Caton et al.8 observed that even with different periodontal treatment modalities, the expected result (formation of new CTA) could not be achieved. The four regenerative treatments were root planing and soft tissue curettage; Widman flap surgery without bone grafting; Widman flap surgery with the placement of frozen autogenous red bone marrow and cancellous bone; and beta-tricalcium phosphate in intrabony defects. All the results showed healing by a long junctional epithelium extending to or close to the same level as before treatment.
In another animal study, Caton and Nyman9 examined the effect of the modified Widman flap procedure on the CTA level and supporting alveolar bone. Treatment of periodontal pockets using this procedure produced no gain in CTA and no increase in crestal bone height. In angular bony defects, a certain degree of “bone fill” was noted. However, this bone repair was never accompanied by new CTA. Therefore, despite significant efforts, researchers are still testing treatment modalities and new materials to achieve the goal of preventing periodontal healing by formation of long junctional epithelium.
Initial Therapy
The following are important steps of the initial periodontal therapy:
It is important to keep in mind that the critical determinant of the success of periodontal therapy is not the choice of treatment modality, but the detailed thoroughness of the root surface debridement and the patient’s standard of oral hygiene. Evidence supports SRP as an essential and effective component of therapy for the majority of inflammatory periodontal diseases.3 (See Chapter 3 for a complete discussion on lasers as an adjunct therapy for this phase of treatment.) If periodontal health cannot be achieved by initial therapy, a surgical approach can be considered.
Periodontal Surgery
Rationale
As mentioned earlier, in assessing risk factors for individual teeth in periodontal patients, the presence of sites with a residual true periodontal pocket depth of 6 mm or deeper after active treatment is considered a strong factor in predicting future periodontal destruction.4 Therefore an important goal of surgical periodontal therapy is to obtain a reduced pocket depth in order to arrest further disease progression.6 In periodontal health, a pocket is referred to as sulcus and ranges from 1 to 3 mm in depth. Based on this guideline, one of the objectives of periodontal surgery is to provide access for proper instrumentation and cleaning of the root surface when root areas are difficult to reach. Selection of available surgical methods should be based on their potential to facilitate removal of subgingival deposits and self-performed plaque control, enhancing the long-term preservation of the periodontium.
Resective Surgery
Resective periodontal surgery is a technique used for pocket elimination. The objective is to facilitate debridement of root surfaces through access. Resective surgery is indicated for the removal of excessive soft periodontal tissue on the gingival margin (gingivectomy) in pseudopockets, or when there is no loss of attachment (as in gingivitis). It is also used to correct true periodontal pockets (with loss of attachment) in periodontitis when both the pocket epithelial lining and the inflamed connective tissue are removed to promote reattachment of connective tissue fibers to the root surface (see Chapter 4).
An undesirable consequence of periodontal treatment, either surgical or nonsurgical, is recession of the gingival margin after healing. In severe cases of periodontitis, this may lead to poor esthetics in the anterior area of the dentition. It is often associated with surgical procedures of bone recontouring for the eradication of bone defects. Therefore, researchers have sought other techniques to avoid or reduce the problems caused by recession. By applying regenerative surgical procedures the lost periodontal attachment in bone defects can be restored.10
Regenerative Periodontal Therapy
Periodontal regeneration surgery is selected to obtain (1) an increase in the periodontal attachment of a severely compromised tooth; (2) a decrease in deep pockets to a more maintainable range; and (3) a reduction of the vertical and horizontal component of furcation defects. Current approaches, however, remain technique sensitive, and clinical success requires application of meticulous diagnostic and treatment strategies.11
As stated in the proceedings of the 1996 World Workshop in Periodontics, the attachment of the tooth is considered to have been regenerated when new cementum with inserting collagen fibers has formed on the detached root surface. Regeneration of the periodontal supporting apparatus (periodontium) also includes regrowth of the alveolar bone. Procedures to restore lost periodontal support have also been described as “reattachment” or “new attachment” procedures. Present regenerative techniques lead to significant amounts of regeneration at localized sites on specific teeth. However, if complete regeneration is to become a reality, additional stimuli to enhance the regenerative process are likely needed and could be accomplished with combined procedures.12
Early Studies and Objectives
Clinical success of regenerative procedures in periodontology, including cases with significant growth of new alveolar bone, may histologically show an epithelial lining along the treated root surface instead of deposition of new cementum.13
The search for techniques and materials to promote regeneration in the periodontium began as early as the 1970s, when researchers formulated the following hypothesis: if epithelium and gingival connective tissue were to be excluded from the healing surgical site, progenitor cells migrating from the periodontal ligament would have the potential to form new connective tissue attachment.14 However, almost all available human histological evidence to date demonstrates healing by a long junctional epithelium with no or minimal CTA.3
The rationale for achieving this goal is to remove the crestal epithelium so as to stop the migration of the epithelial tissue cells into the pocket. In so doing, regeneration could then occur at the expense of the periodontal ligament, with insertion of new fibers to the root surface. Thus, removal of sulcular/pocket epithelium has been the foundation of several other treatment modalities, including subgingival curettage, the excisional new attachment procedure (ENAP), and the modified Widman flap procedure. All have the objective of setting up an environment for new CTA.15–18
Laser Types
Investigations into a predictable method of epithelial exclusion applied the unique characteristics of the laser wound in periodontal therapy. Lasers can be excellent adjuncts to achieve this goal, and several laser wavelengths have been studied. Evidence of delayed epithelialization found in carbon dioxide (CO2) laser wounds, for example, was determined in a series of animal and human studies. Animal trials had shown the ability of the CO2 laser to retard epithelial downgrowth after periodontal surgery for up to 14 days,19 confirming that the CO2 laser can remove epithelium from the gingiva without causing underlying damage to the connective tissue.20,21 An early human study performed with the CO2 laser evaluated whether de-epithelialization with this type of laser at flap surgery and at 10-day intervals over the first 30 days of healing had the potential to enhance CTA formation. The de-epithelialization was repeated on the test side at 10, 20, and 30 days postsurgically. This interval was determined based on the knowledge that regeneration of epithelial tissue occurs in 10 to 14 days, when it begins to grow into the pocket, lining the soft tissue wall of the new sulcus. The laser-treated side in one patient showed a fill with CTA and with some repair cementum, which was not seen in controls.22 This preliminary finding in humans led to other laser studies.
The use of a pulsed CO2 laser to de-epithelialize the gingival flaps is an attempt to exclude epithelial cells from the healing wound21 and has been used with and without the benefit of GTR membranes. Other results from human studies and case reports combined with animal trials indicate a positive benefit in wound healing because of the laser de-epithelialization technique.23
Another human study compared conventional periodontal surgery combined with CO2 laser and conventional periodontal surgery alone with respect to epithelial elimination and degree of necrosis of mucoperiosteal flaps. The results confirmed that (1) a more complete removal of sulcular epithelium was obtained by CO2 laser than by scalpels, and (2) the CO2 laser technique effectively removes the oral and sulcular epithelium from a gingival flap without damaging the viability of the flap during wound healing.21
Some believe that this technique has shown significantly better results than those obtained through conventional osseous grafting alone and appears to be comparable to the results reported for GTR procedures with barrier membranes. This concept provides a paradigm shift from the conventional use of GTR therapy by acknowledging the difficulty in controlling epithelium during the early wound-healing process. It also allows a more comprehensive therapy for periodontal disease that addresses the generalized nature of the disease, with multiple lesions being treated concurrently in an economical manner. The patient presenting with generalized advanced periodontal disease could have several defects treated definitively in one quadrant using the laser de-epithelialization technique, without the need for multiple membrane therapies.23
Understanding the benefits of the laser light in delaying epithelial growth into the periodontal sulcus during the healing process requires consideration of the wound-healing mechanism after laser use. With the pulsed CO2 laser, for example, researchers have found that this wavelength can create a rather unique wound in the gingival tissues. It is not a burn, but rather an instantaneous vaporization of the intracellular fluid and resultant disintegration of the cell structure.24 The laser wound on skin, mucous membrane,25 and gingiva causes a delay in re-epithelialization because of factors such as reduced inflammatory response and less wound contraction.26 To explain further the delay in CO2 laser wound epithelialization, previous studies have proposed the following combination of events:
The repair process of coagulation necrosis produced by pulsed CO2 laser irradiation with relatively low fluence does not disturb this repair process, but rather promotes its steady progress and subsequent tissue remodeling.31 As for the application on skin, the pulsed CO2 laser is capable of bloodless skin ablation with improved wound healing at relatively low irradiance (2 W). Increasing the repetition rate (100 Hz) of the CO2 laser helps to achieve a better surgical outcome. This laser may be a valuable instrument for ablation of skin and skin lesions when used in a pulsed mode.32
Because the new healed interface between the soft tissues and the root was not known histologically, Yukna et al.33 conducted a study to evaluate wound healing after laser-assisted new attachment procedure in periodontal pockets. A free-running pulsed Nd:YAG laser was used to treat six single-rooted teeth with moderate to advanced chronic periodontitis associated with subgingival calculus deposits. After 3 months, all treated teeth were removed en bloc for histological processing. Laser-assisted new attachment procedure–treated teeth exhibited greater probing depth reductions and clinical gains in probing attachment level than the control teeth. All laser-assisted new attachment procedure–treated specimens showed new cementum and CTA in, and occasionally coronal to, the bur notch on the root. As for the controls, five of the six teeth had a long junctional epithelium with no evidence of new attachment or regeneration. There was no evidence of any adverse histological changes around the laser-assisted new attachment procedure specimens.
These cases support the concept that laser-assisted new attachment procedure can be associated with cementum-mediated new CTA and apparent periodontal regeneration of diseased root surfaces in humans.33 Based on current evidence, the pulsed CO2 and free-running Nd:YAG lasers are the wavelengths best supported by the literature for the laser-assisted new attachment procedure. More histological proof is needed to provide further rationale for the use of the Nd:YAG wavelength.
Biomodification of Root Surface
In 1976 Melcher14 suggested that the type of cell that repopulates the root surface after periodontal surgery determines the nature of the attachment that will form. Ideally, healing of the periodontium should occur from periodontal ligament cells that have the potential to form a new CTA, proof of which was recently provided using a novel and unique experimental model in dogs.34 The purpose of this study was to explore the formation of periodontal tissues around titanium implants. After resection of the crowns of the maxillary canine teeth in nine mongrel dogs, the roots were hollowed to a depth of 5 mm, leaving a thin dentinal wall. Slits were prepared in the cavity wall to create passages from the chamber to the periodontal ligament area. A custom-made, titanium implant was placed into the center of each chamber. Machined, titanium plasma–sprayed (TPS) and sandblasted with large grit and acid–attacked (SLA) surfaces were used. A collagen barrier was placed over the submerged chamber. After 4 months of healing, jaw sections were processed for histology. Newly formed periodontal ligament, alveolar bone, and root cementum filled the space between the implant and the wall of the chamber. Ingrown bone was neither in contact with dentin nor with the implant. Thus, an interposed soft connective tissue layer was present. Healing by fibrous encapsulation was observed around most implants. This study confirmed that strong evidence shows the progenitor cells for periodontal attachment formation reside in the periodontal ligament and not in the alveolar bone as previously assumed.
Over the years, studies have evaluated several aspects of root surface preparation, starting with simple removal of bacterial deposits, calculus, and endotoxins. It has been well established that these essential steps in the healing process are part of initial periodontal therapy. More sophisticated studies, in animal models, showed improved healing response histologically after citric acid and tetracycline root surface demineralization.35,36 Additional animal studies demonstrated adverse outcomes with the use of citric acid, such as root resorption and ankylosis.37,38 In humans, studies have demonstrated histological new CTA after citric acid demineralization of root surfaces.39 Other studies showed no statistical difference in clinical conditions after flap surgery when comparing controls to citric acid–treated roots.40,41 Citric acid and tetracycline for root preparation are rarely used at present because of the contradictory clinical outcomes.11
Biomodification of the root surface with demineralization through ethylenediaminetetraacetic acid (EDTA) followed by enamel matrix proteins (Emdogain) during surgery has been introduced to accomplish regeneration of periodontal tissues. The biological concept is that the application of enamel matrix proteins (amelogenins) may promote periodontal regeneration because it mimics events that took place during the development of the periodontal tissues.42,43 However, it is unclear how this concept stands in accordance with current knowledge about periodontal wound healing, because no evidence shows that the cells derived from the periodontal ligament are the cells encouraged to repopulate the root surface after treatment.11
Emdogain (EMD) has been extensively studied, with contradictory results. Some trials show ankylosis and root resorption.44 A recent in vitro study has failed to confirm that EMD has any significant effect on periodontal ligament cell proliferation.45 Better results with the use of EMD appear in case series showing 70% of bone fill in intrabony defects and 4 to 4.5 mm of gain in clinical attachment.46,47 Additional clinical and radiographic evidence showed larger amounts of probing attachment level (PAL) gains and statistically significantly more bone gain using the EMD versus open-flap debridement only.48,49
Compared with GTR, EMD showed similar results for clinical attachment level (CAL) gain and PAL gain.50–53 Further studies evaluated EMD in combination with some bone graft materials, achieving clinical improvements.54–56 Other investigations failed to demonstrate a beneficial effect of this combined treatment.57 Based on studies with EMD, histological evidence of new cementum formation can be observed, with collagen fibers inserting into a previously periodontitis-affected root surface and the formation of new alveolar bone in human specimens.51,58
Growth regulatory factors for periodontal regeneration may represent a potential aid in attempts to encourage regeneration of the periodontium. Such products include platelet-derived growth factor (PDGF) and insulin-like growth factor (IGF). Some studies show that control sites treated without growth factors healed with a long junctional epithelium and no new cementum or bone formation, whereas regeneration of a periodontal attachment apparatus occurred at the sites treated with growth factors.59–65
As stated earlier, surgical access should be considered only as an adjunct to cause-related therapy. A primary rationale for raising a flap is to provide access for proper instrumentation and cleaning of the root surface. If these cannot be done because of tooth anatomy, flap surgery should be considered only after as much of the etiological agents of the periodontal disease are removed by closed techniques. Case Studies 5-1 and 5-2 illustrate these points.
Case Study 5-1 Pocket Biomodification
Figure 5-1, A, shows a preoperative view of the patient on presentation. After conventional SRP, a laser was used to de-epithelialize the marginal gingiva, as shown in B. The reason for this initial step is based on sound biological principles: in order for a true fibroblast-mediated soft tissue reattachment to the root surface to occur, the epithelium must be prevented from migrating into the pocket. Removal of the marginal epithelium will prevent its migration into the pocket.
Once the marginal gingiva has been removed, the laser is then placed into the pocket parallel to the long axis of the root. The purpose of this step is the reduction/elimination of bacteria (removal of the etiologic agent) from the pocket (Figure 5-1, C). The various protocols for the depth of placement of the laser into the pocket depend on the wavelength used, manufacturer’s suggestions, and other criteria. It is important to recognize that some protocols do not address removal of the marginal gingiva before entering the pocket.

Figure 5-1, D, shows the 1-week postoperative view of laser treatment of the upper-right quadrant, as well as immediate postoperative view of laser treatment of the upper-left side. Figure 5-1, E shows the upper-left quadrant immediately after laser treatment. F shows the 1-month postoperative clinical view; G shows the preoperative charting; and H shows the 1-month postoperative charting.
Case Study 5-2 Furcation and Biomodification
Surgical access was necessary because of an anatomical problem in this 52-year-old man with no relevant medical history. Figure 5-2, A, shows an upper-left second molar with a diseased furcation. Periodontal probing of the furcation is 10 mm. Because of the fluting and curvature of the mesiobuccal root distally toward the furcation, and the distobuccal root mesially toward the furcation, it was impossible to use conventional instrumentation in this patient to remove the etiological factors.
Figure 5-2, B, shows the surgical site after elevation of a flap. Exposure of the root surfaces showed a ball of diseased tissue in the furcation, firmly adherent to the roots. After use of conventional instruments (curettes) to remove the bulk of the diseased tissue, a laser was used to remove the remnants of soft tissue from the root surface and biomodify the root surface to increase the attachment of fibroblasts to the root surface.
Figure 5-2, C, shows the root surface after laser biomodification. The inner surface of the flap was then de-epithelialized. Once again, the purpose of removing the epithelium from the flap is to allow the growth of a fibroblast-mediated soft tissue attachment to the biomodified root surface. Bone analog was placed in the furcation and the flap sutured into place. The laser was then used to de-epithelialize the gingival margin of the flap. The patient was dismissed with routine postoperative instructions. At 9 days postoperatively, the patient returned to the office. The sutures were removed, and the flap margin was again de-epithelialized. At 19 days the patient returned and had the gingival margin de-epithelialized once again.
The rationale for three sessions of de-epithelialization (day of surgery, 9 days, and 19 days postoperatively) is based on sound biological principles. The work of Rossmann, Centty, Israel, and others19–23 showed that removal of epithelium at 10-day intervals over the first 30 days enhanced a soft tissue attachment.
Figure 5-2, D, shows the surgical site with a fully healed furcation 90 days postoperatively.
Comparison of Four Lasers: Effects on Root Surfaces and Wound Healing
Lasers have mutiple uses in periodontal therapy. This section reviews four different wavelengths and their application in regenerative procedures. Most studies used the CO2 laser, Nd:YAG laser, erbium family of lasers, and to a lesser extent the diode laser.66 Lasers can be used for root surface biomodification in promoting ligament cell reinsertion or creating a proper surface for reattachment. The modification of cementum and dentin is wavelength dependent and is discussed for each laser.
The mineral component of both cementum and dentin is a carbonated hydroxyapatite that has intense absorption bands in the midinfrared region of the light spectrum. Consequently, of the laser wavelengths studied, the Er:YAG laser would appear to be the instrument of choice for effective removal of calculus, for root etching, and for creation of a biocompatible surface for cell or tissue reattachment.66,67 Research indicates that its safety and effects might be expected to be within the range reported for conventional mechanical debridement.68
A meaningful comparison between various clinical studies or between laser and conventional therapy is difficult at best and likely impossible at present. Reasons include different laser wavelengths; wide variations in laser parameters; insufficient reporting parameters, which in turn do not allow calculation of energy density; differences in experimental design; lack of proper controls; variable severity of disease and treatment protocol; and measurement of different clinical end points. Despite these problems and the lack of human clinical trials, sufficient data are available to recognize trends in the results of laser-mediated treatment of chronic periodontitis.67
Carbon Dioxide Laser
In chronological terms, the CO2 laser (wavelength of 10,600 nm) was the first of the currently available wavelengths studied for effects on root surfaces. Even at power settings as low as 4 W, the results of the earlier studies were not particularly encouraging, in that charring and melting of the root surface were common findings. In addition, Fourier-transformed infrared spectroscopy analysis of charred surfaces revealed the presence of cyanamide and cyanate, both cytotoxic chemical residues.69,70 However, the parameters used in these early studies, as well as the temporal emission mode used, were completely different from parameters used today. These early studies used a continuous-wave CO2 laser, which is known to damage hard tissues more than pulsed CO2 mode.
Another issue is water cooling. It is important to use irrigation during CO2 laser application to avoid collateral zones of heat damage and production of toxic substances.69–71 Israel et al.71 compared three laser wavelengths—CO2, Nd:YAG, and Er:YAG—for root surface changes after irradiation with and without air/water surface cooling. Those changes included cavitation defects, globules of melted and resolidified mineral, surface crazing (cracking), and production of a superficial char layer after irradiation with CO2 and Nd:YAG. In contrast, the Er:YAG laser produced root surface changes that might be expected from acid etching: removal of the smear layer and exposure of the collagen matrix. Although sharply defined microfractures of the mineralized structure were noted with the Er:YAG laser, unlike the CO2 and Nd:YAG lasers, there was no evidence of melting or surface char. Given the parameters of this study, it appears that both the CO2 laser and the Nd:YAG laser adversely alter the root surface. The Er:YAG laser, however, when used at low energy densities, shows sufficient potential for root surface modification to warrant further investigation.
Barone et al.72 showed that heat-induced cracking of the root surface occurs when the CO2 laser is used at power settings of 4 W or greater in a continuous mode. However, when used with pulsed wave in a defocused mode and at low power settings, the CO2 laser appears to cause minimal damage. Although both laser delivery modes produced changes to the treated root surface specimens, the changes from a defocused beam created a smooth surface, which may present an advantage in periodontal treatment.72 On the other hand, when used in continuous mode, the CO2 laser (0.5 W, 20 sec, continuous circular movements) creates a molten, recrystallized (nonporous, glazed) surface on root dentin, with the tubules almost completely sealed.73
After root planing, the instrumented root surface is invariably covered by a surface smear layer containing remnants of dental calculus, contaminated root cementum, bacterial endotoxins, and subgingival plaque. Studies have investigated alternatives to compensate for the limitations of mechanical root therapy. Crespi et al.74 found that the resultant root surface had areas devoid of cementum, with completely sealed dentinal tubules. The dentin surface appeared as a melted layer showing a flat, smooth surface with apparent fusion of the smear layer. The dentin layer had the appearance of a glazed surface. No residual bacteria were observed on any roots after CO2 laser irradiation. Other investigations show that the CO2 laser used in a pulsed, defocused mode and at low power settings (1.0 sec at 3 W) completely removed the smear layer, leaving partially exposed dentinal tubules with minimal change in their diameter. Root surface modifiers (e.g., EDTA, citric acid) were also effective in removing the smear layer, but the exposed dentinal tubules showed funnel-shaped widening.75
Recent studies of the biocompatibility of the CO2 laser with root surfaces show conflicting results, even at low energy densities. Crespi et al.76 and Pant et al.77 reported increased in vitro attachment of fibroblasts to laser-treated surfaces compared with controls (SRP only) or chemically treated surfaces. However, Fayad et al.78 reported a total lack of fibroblast attachment to surfaces irradiated at only 1.25 mJ/pulse.
As for the periodontal soft tissue wall, Gopin et al.79 evaluated soft tissue attachment to root surfaces after CO2 laser irradiation in an animal model. After mucoperiosteal flaps were raised, each laser-treated root surface was irradiated until a confluent char layer was visually evident. The settings and parameters were 6 W, 20 Hz, pulse length of 0.01 second, energy density approximately 240 J/cm2, and 2 mm from target surface with a focal spot diameter of 0.8 mm. This application promoted a cytotoxic char layer on the root surfaces. Where charring was observed, histological examination revealed a lack of flap reattachment to the root surface. However, all specimens treated by SRP alone or by laser irradiation followed by SRP exhibited flap reattachment to treated surfaces. These findings led to the use of the pulsed CO2 laser as an adjunctive treatment, along with SRP, in treatment of periodontal pockets in humans.
As for the use of the CO2 laser on bone, in an animal model, osteotomy defects induced by laser (vs. rotary bur) exhibited a delayed healing response, apparently related to residual char in the osseous defect.80 The healing response was severely delayed even in the presence of a surface cooling spray of air/water.81 Therefore the CO2 wavelength is not appropriate for use on bone tissue.80,81
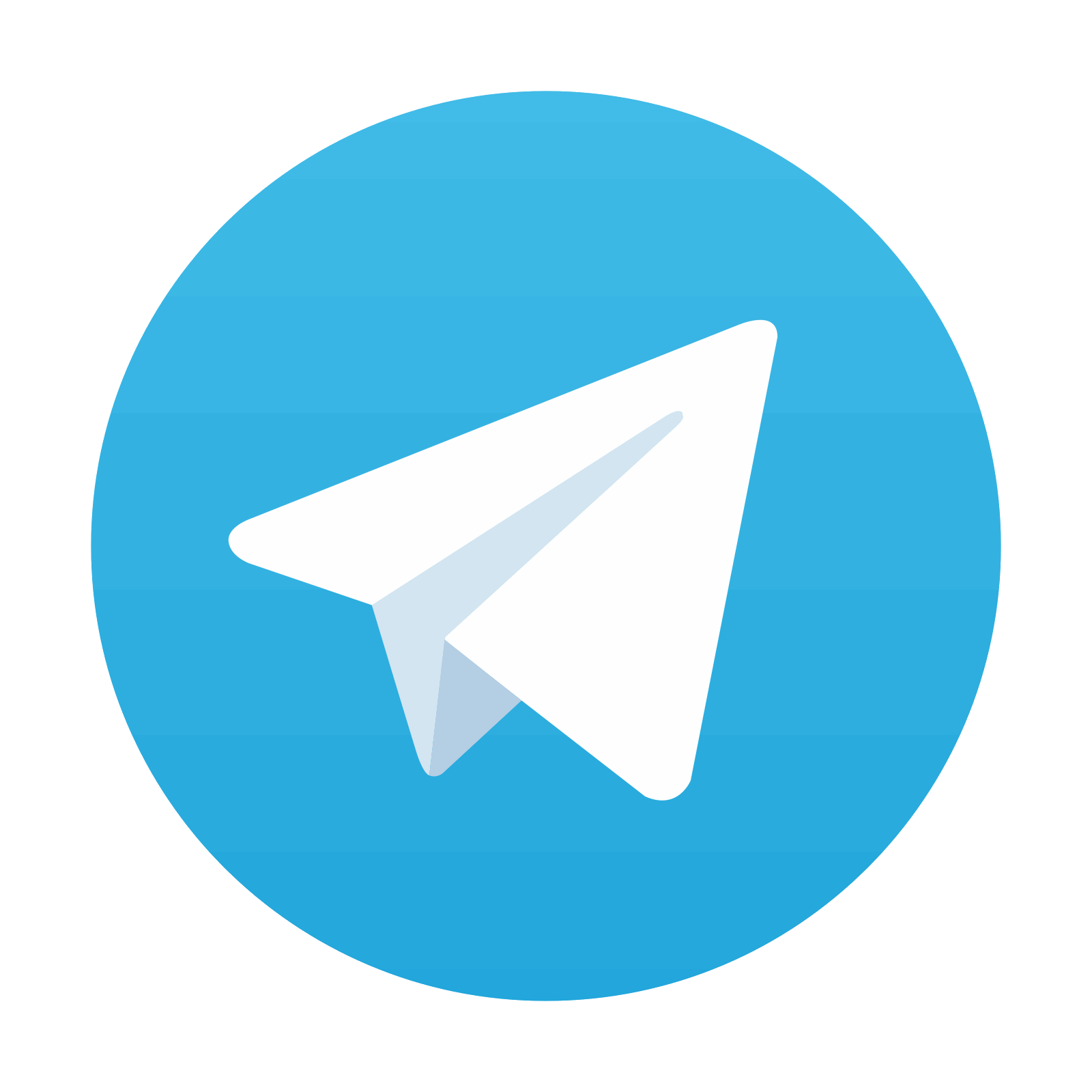
Stay updated, free dental videos. Join our Telegram channel

VIDEdental - Online dental courses
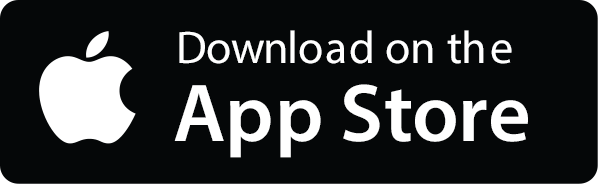
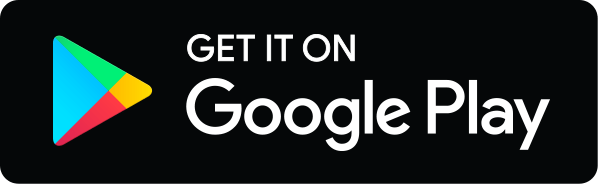