Chapter 4
Enamel
Arthur R. Hand
Department of Craniofacial Sciences and Cell Biology, School of Dental Medicine, University of Connecticut
Enamel is the hard, mineralized tissue that covers the anatomic crowns of the teeth. Although the color of enamel generally appears to be white, enamel is relatively translucent and its appearance can be affected by the color of the underlying dentin or adjacent restorations. The formation, composition, and structure of mature enamel are unique. Unlike the other mineralized tissues of the body, bone, dentin, and cementum, which are formed by cells of mesenchymal origin, enamel is formed by epithelial cells. Whereas the formation of dentin and cementum continues after tooth eruption, enamel is completely formed before eruption. Mature enamel is acellular, and its formative cells are lost upon eruption of the tooth. This dictates that enamel repair (except in the case of initial caries lesions; see “Dental Caries” later in this chapter) or replacement, at least at present, must be achieved through the use of non-biological substances.
Enamel structure, composition, and properties
Enamel is the hardest substance in the vertebrate body. Enamel contains about 96% mineral, 2% organic material, and 2% water, by weight. The mineral component, as in all other vertebrate mineralized tissues, is hydroxyapatite, Ca10(PO4)6(OH)2. Like other mineralized tissues, some of the calcium, phosphate, and hydroxyl ions of the enamel hydroxyapatite crystals may be replaced by other ions. These include strontium, magnesium, carbonate, and fluoride. Technically, the mineral of enamel is a substituted hydroxyapatite.
Hydroxyapatite forms hexagonal crystals, thus enamel crystals have six sides when seen in cross-section (Fig. 4.1). Enamel crystals are much larger than the hydroxyapatite crystals in other tissues, whose dimensions typically are 5 to 10 nm in width, 1.5 to 3.5 nm in thickness, and 40 to 50 nm in length. Enamel crystals may be up to 70 nm in width and 30 nm in thickness, and are much longer than the crystals in bone, dentin, and cementum. Although their exact length has not been determined, some investigators believe that individual enamel crystals may extend from the dentinoenamel junction to the enamel surface. The difference in size of hydroxyapatite crystals found in enamel and those in other mineralized tissues most likely can be attributed to the properties of their matrix components.
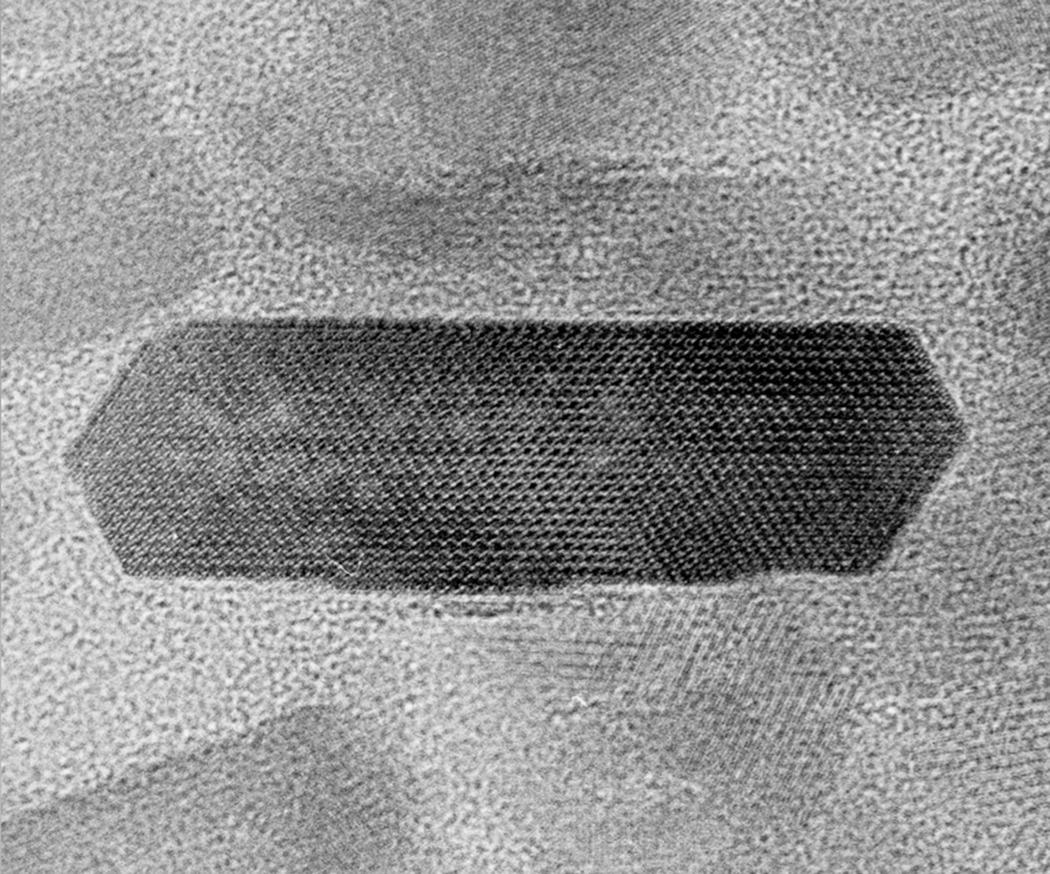
Figure 4.1 Enamel crystal. High magnification electron micrograph of a cross-sectioned hydroxyapatite enamel crystal. The separation of the lattice striations, which intersect at 60° angles, is 0.817 nanometers.
(Reproduced with permission from: Yanagisawa, T. (2009) A Color Atlas of Oral Histology and Embryology, 3rd edn. Wakaba, Tokyo.)
The organic content of mature enamel consists mainly of residual enamel matrix proteins or fragments of these proteins, mostly coating the surfaces of the enamel crystals. These proteins are produced by ameloblasts, the cells that make the enamel, and they have critical functions in the formation and mineralization of the enamel. During the maturation of the enamel, most of the matrix proteins are degraded, and along with most of the water in the matrix removed to create space for the mineral. The surface layers of enamel also may contain some salivary proteins that are adsorbed to the enamel crystals or incorporated during the repair of initial caries lesions.
The enamel crystals are organized into enamel rods (or prisms) that extend from the dentinoenamel junction to the surface of the tooth (Fig. 4.2). Within the rods the crystals are oriented predominantly parallel to the rod. Each rod is about 4 to 5 μm in diameter, with a more or less circular or slightly oval cross-section. The rods may be up to 2.5 to 3 mm in length, depending on the tooth and the location on the tooth. Additional enamel crystals, located in the interrod enamel, surround each rod. The interrod enamel crystals are oriented at an angle to those of the rod (Fig. 4.3). Around the occlusal or incisal three-quarters of each rod, the differing orientation of the rod and interrod crystals creates a slight space. This space contains an increased amount of residual matrix proteins and is called the rod sheath (Fig. 4.4). Along the cervical one-fourth of each rod, the divergence of the rod and interrod crystals is more gradual, and the rod sheath is absent. In scanning electron micrographs (Fig. 4.5), or in sections cut perpendicular to the rods, the cervical interrod enamel appears to form a “tail” attached to the “body” of the rod just occlusal to it. The observation of this pattern gave rise to the initial interpretation that the enamel rods were shaped like keyholes. It is now generally accepted that the rod-interrod organization described above reflects the true structure of enamel.
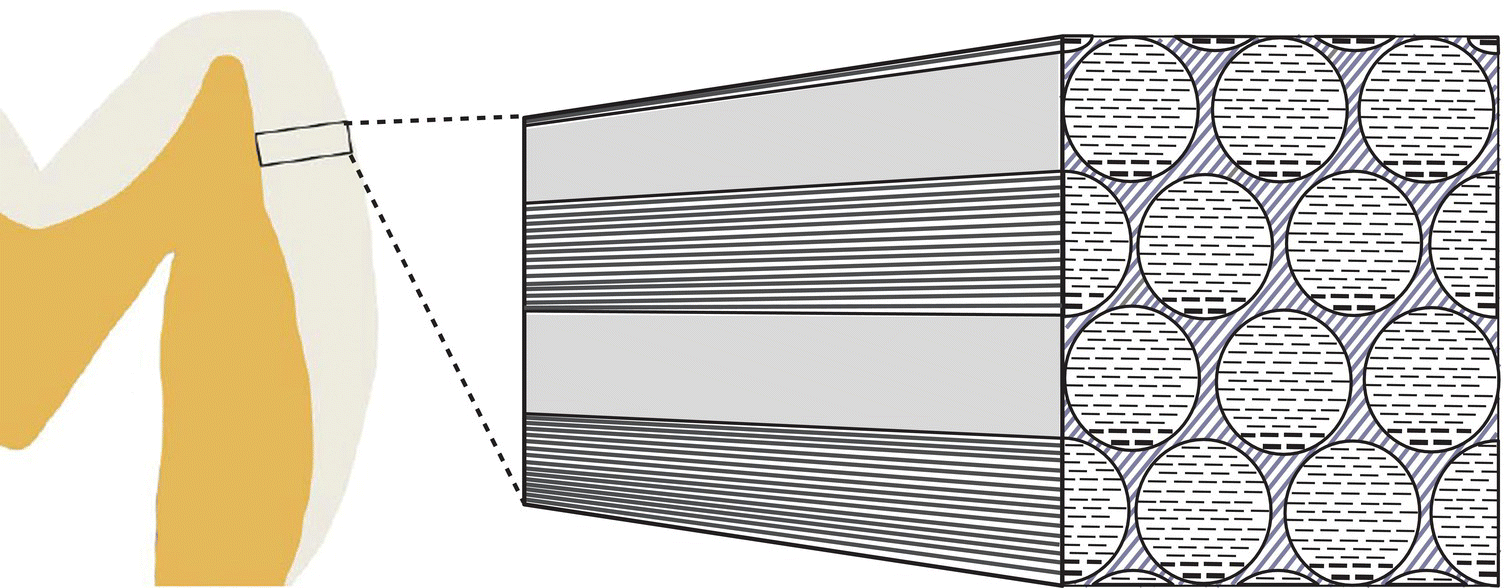
Figure 4.2 Diagram of the main structural features of enamel. Enamel consists of circular- or oval-shaped rods that extend from close to the dentinoenamel junction to the enamel surface. The rods consist of very long enamel crystals, oriented parallel to the long axes of the rods. The enamel crystals are seen in cross-section in the rod profiles in the right portion of the diagram. Interrod enamel is located between the rods. The crystals of interrod enamel, shown here as lighter diagonal lines, have a different orientation than those of the rods.
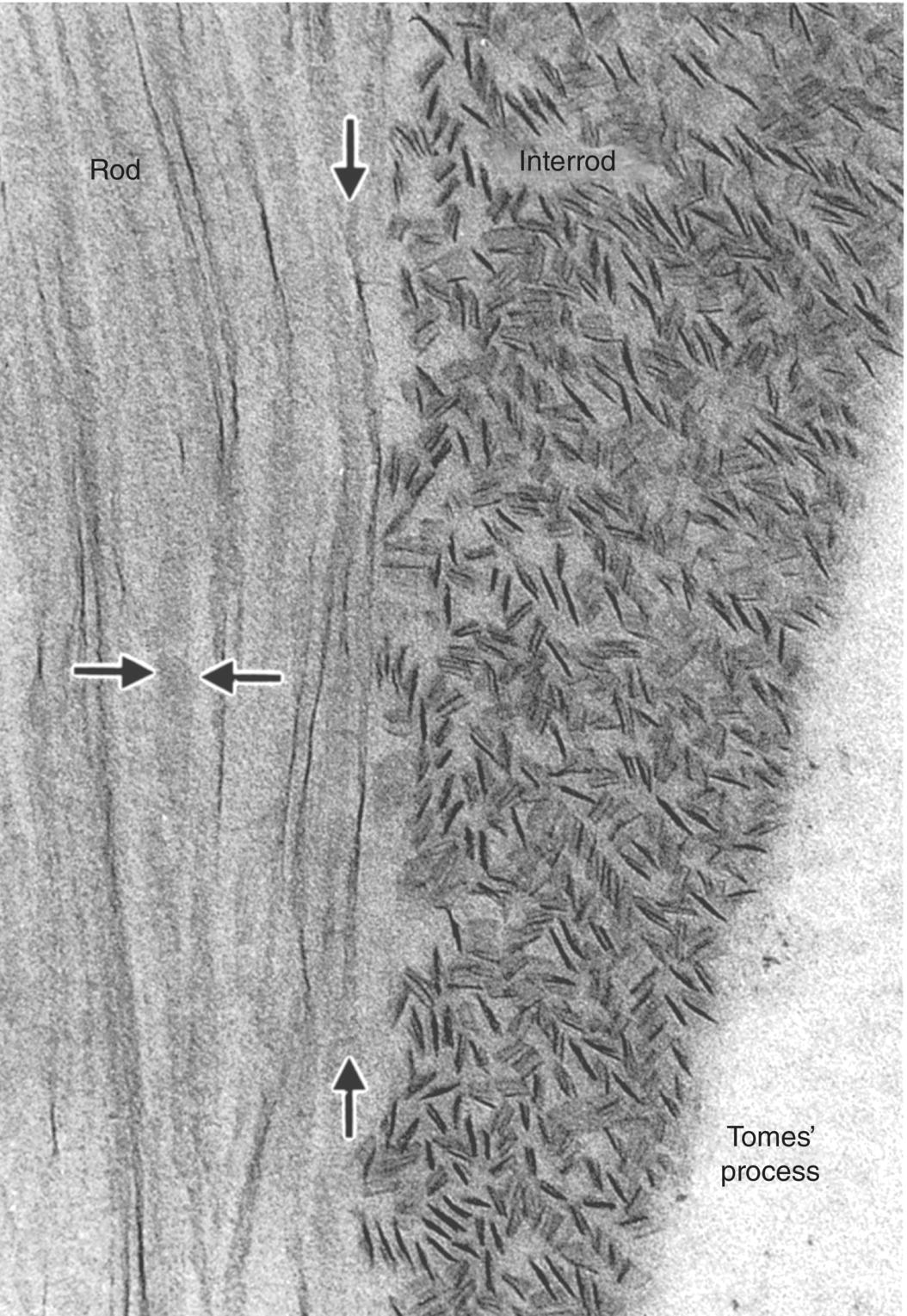
Figure 4.3 Transmission electron micrograph of newly formed enamel showing the differing orientations of crystals in rod and interrod enamel. The longitudinally oriented crystals in the rod are seen on edge or in face view (between the horizontal arrows). Twisting of an individual crystal is seen between the vertical arrows. The crystals of the interrod enamel are seen mainly in cross-section.
(From: Warshawsky, H. (1988) The teeth. In: Cell and Tissue Biology: A Textbook of Histology (L. Weiss) 6th edn. pp. 595–640. Urban and Schwarzenberg, Baltimore. Reproduced by permission of Elsevier.)
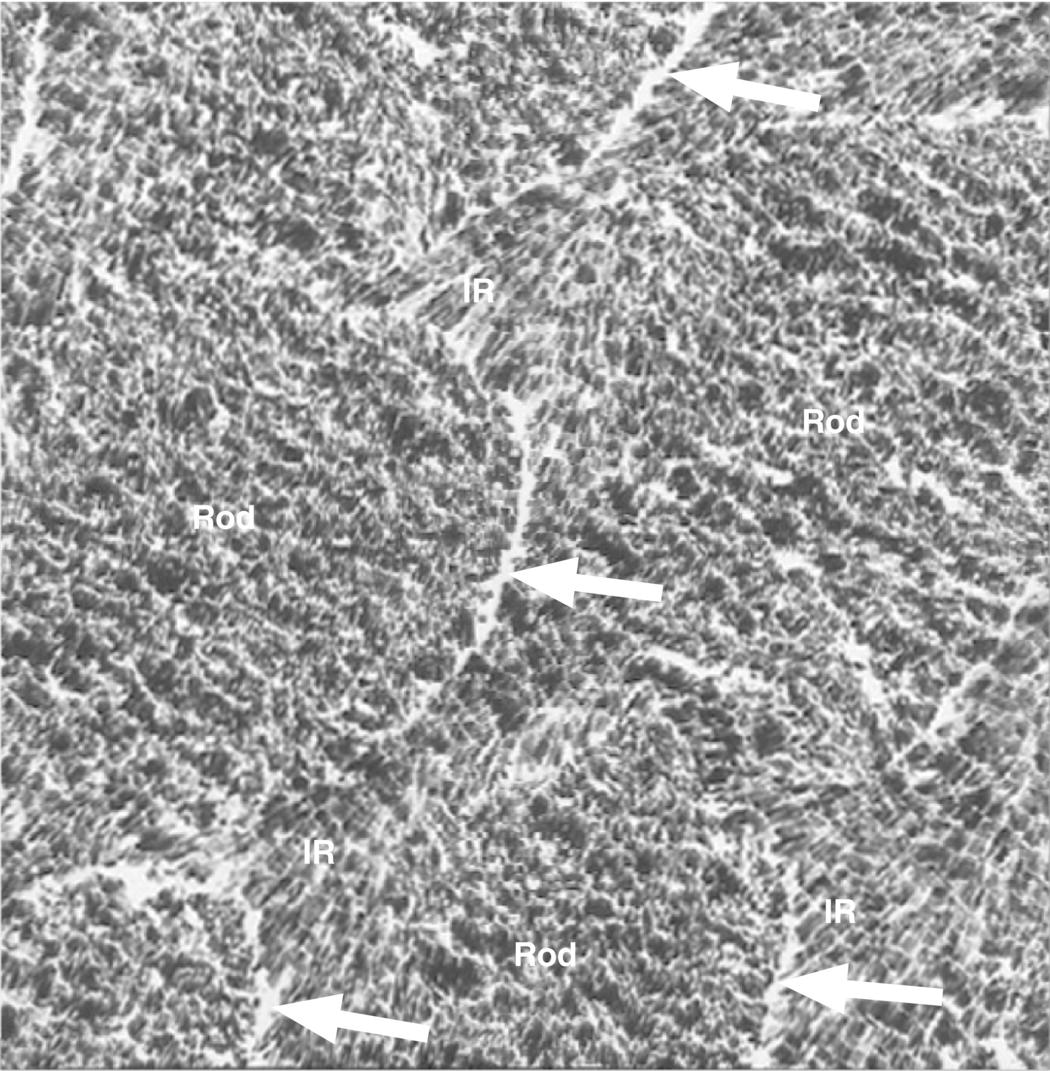
Figure 4.4 Electron micrograph of cross-sectioned enamel rods of mature enamel. Note the different orientations of the enamel crystals in the rod and interrod (IR) enamel. The rod sheathes (arrows) can be seen as increased space at the interface between the rod and interrod enamel. The residual enamel matrix proteins in between the crystals and in the rod sheathes are not visible due to the high contrast of the enamel crystals.
(Courtesy of T. Yanagisawa, Tokyo Dental College.)
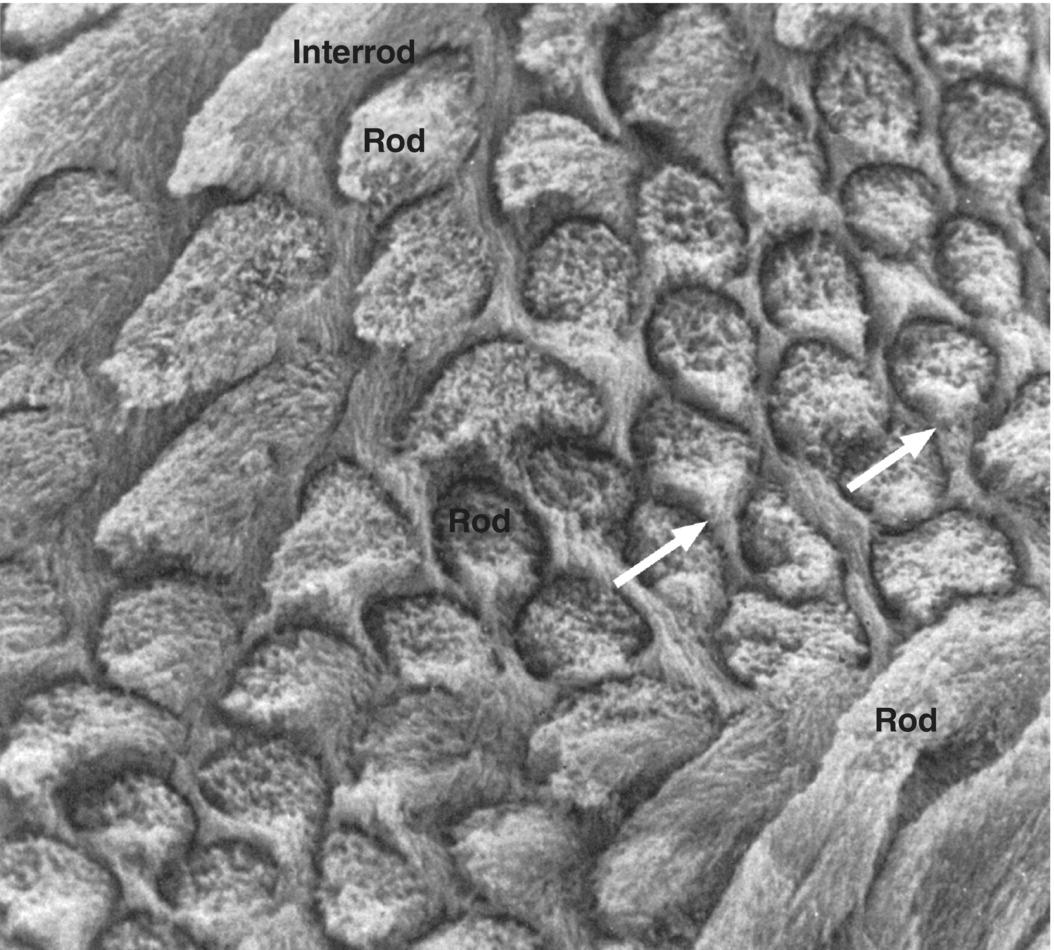
Figure 4.5 Scanning electron micrograph of enamel rods and interrod enamel of a human molar. The surface was etched with a demineralizing agent to enhance the features. The differing orientation of the rods is evident. At the top left and bottom right of the image, the rods run obliquely; in the center the rods are seen in cross-section. The interrod enamel forms a continuous phase around the rods. In the cross-sectioned rods, the cervical portion of the rod is continuous with the interrod enamel (arrows), whereas a space (rod sheath, exaggerated due to the etching) is present around the other surfaces of the rods.
(From: Warshawsky, H. (1988) The teeth. In: Cell and Tissue Biology: A Textbook of Histology (L. Weiss) 6th edn. pp. 595–640. Urban and Schwarzenberg, Baltimore. Reproduced by permission of Elsevier.)
In ground sections of enamel, individual enamel rods exhibit a pattern of cross-striations along their length, at regular intervals of about 4 μm (Fig. 4.6). These cross-striations are thought to represent the daily rhythm of enamel formation and mineralization. Because ground sections of enamel may be up to 100 μm thick, the orientation of the rods within the section is difficult to determine. If the rods are sectioned obliquely, the interrod enamel between adjacent rods may appear as cross-striations along a single rod. Also, when viewed in the scanning electron microscope, the rods show periodic variations in diameter, which may contribute to the appearance of cross-striations in ground sections.
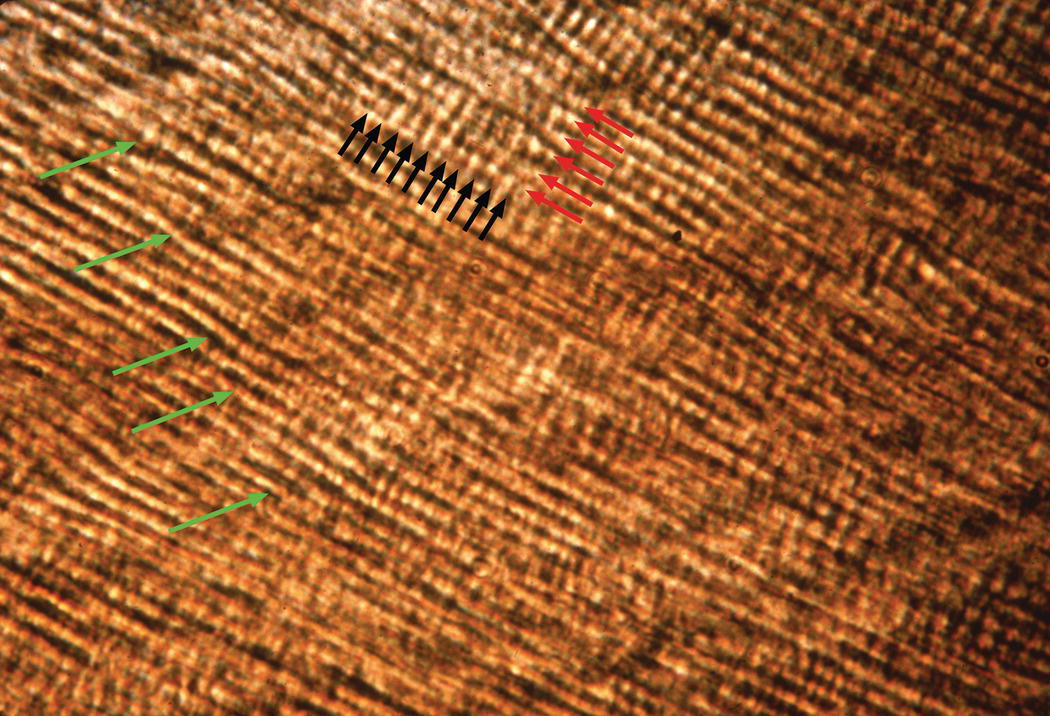
Figure 4.6 Enamel rods. High magnification light micrograph of a ground section showing enamel rods (red arrows) running from lower right to upper left. The closely spaced light and dark lines perpendicular to the rod direction are cross-striations (black arrows). Incremental lines (striae of Retzius, green arrows) run from lower left to upper right.
Additional longer-term variations in enamel formation result in incremental lines, or striae of Retzius, representing the position of the forming enamel surface at that point in time (Fig. 4.7). The striae of Retzius occur at approximately 7- to 11-day intervals during enamel formation and are thought to arise from the coincidence of two separate biological rhythms. In an erupted tooth, the intersection of the striae of Retzius with the enamel surface results in shallow horizontal grooves, perikymata, that encircle the crown of the tooth. Systemic disturbances that occur during tooth development, such as fevers, will cause the formation of additional incremental lines. The physiological changes that occur at birth also cause a prominent incremental line, the neonatal line (Fig. 4.8). Because enamel is not remodeled over its lifespan, the cross-striations and the striae of Retzius are permanent indicators of the incremental pattern of enamel growth.
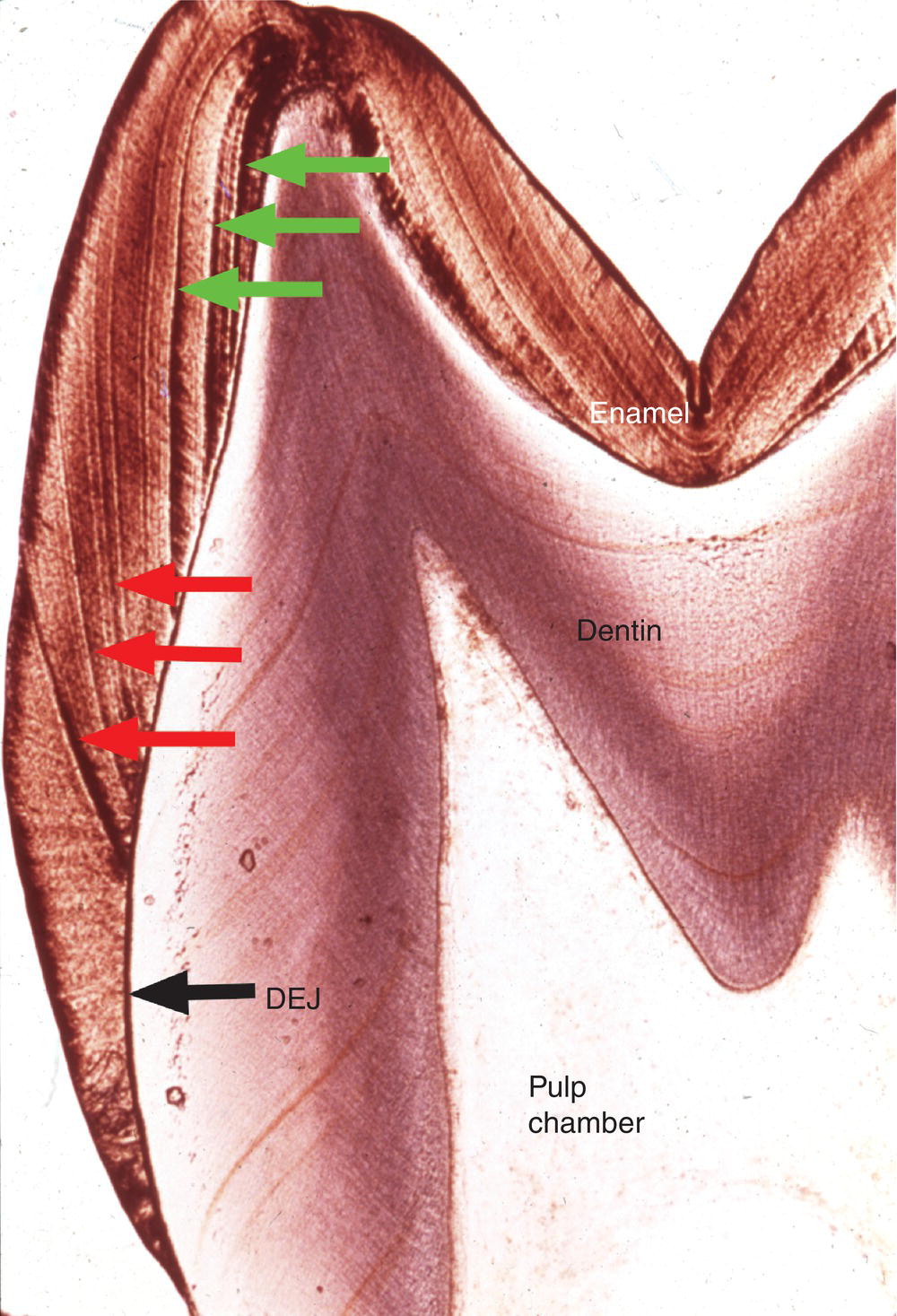
Figure 4.7 Striae of Retzius. The striae of Retzius or incremental lines (red arrows) extend from the dentinoenamel junction (DEJ) to the enamel surface. Perikymata are found where the striae of Retzius intersect the enamel surface. Because enamel formation begins at the cusp tips, striae of Rezius formed early in development (green arrows) do not intersect the tooth surface.
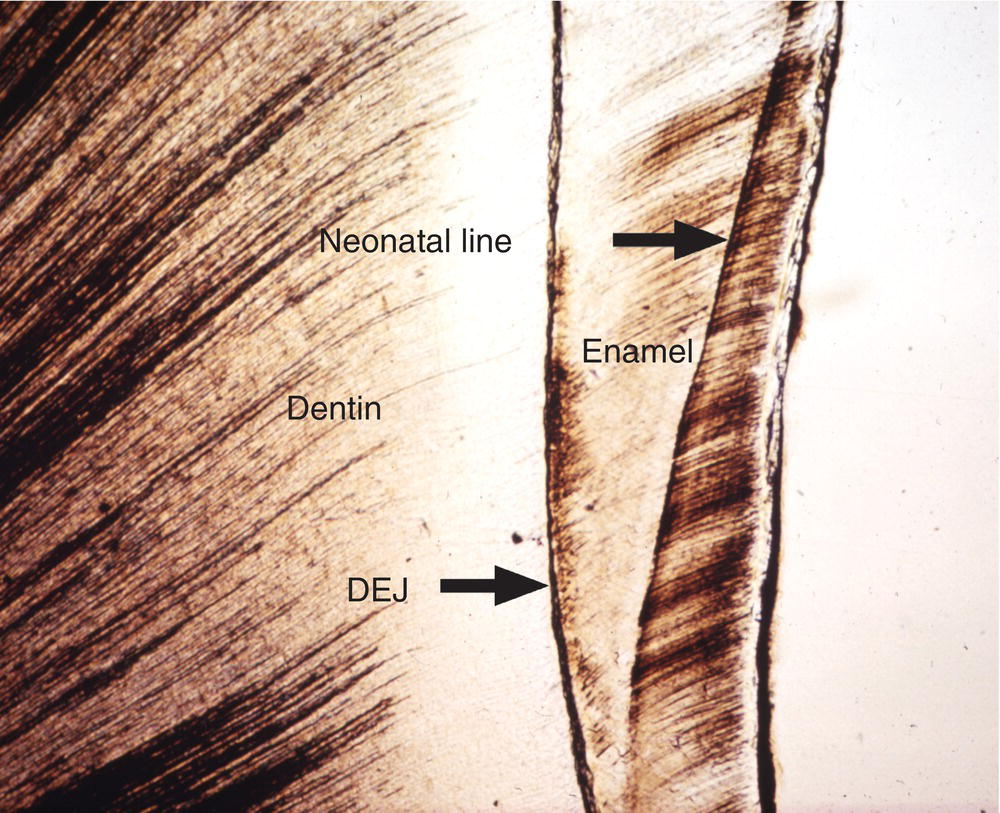
Figure 4.8 Neonatal line. The neonatal line is a prominent incremental line formed in the enamel of teeth developing at birth. Dentinoenamel junction (DEJ).
The course of each rod from the dentinoenamel junction to the surface of the tooth is not straight, but shows a slight curvature. Additionally, each horizontal row of rods is oriented at a slight angle to the adjacent occlusal and cervical rows, creating an undulating pattern of rod orientations from the occlusal/incisal edge of the tooth to the cervical margin (Fig. 4.5). This pattern is evident in ground sections of mature enamel viewed in reflected light, where adjacent bright and dark bands, the Hunter-Schreger bands, can be seen extending from the dentinoenamel junction to the enamel surface (Fig. 4.9). This varying orientation of the rods and matrix also is visible in sections of immature enamel stained with hematoxylin and eosin. In regions of sharp surface curvature, such as cusp tips and incisal edges, the curved path of the rods is accentuated; in ground sections this results in the appearance of gnarled enamel (Fig. 4.10).
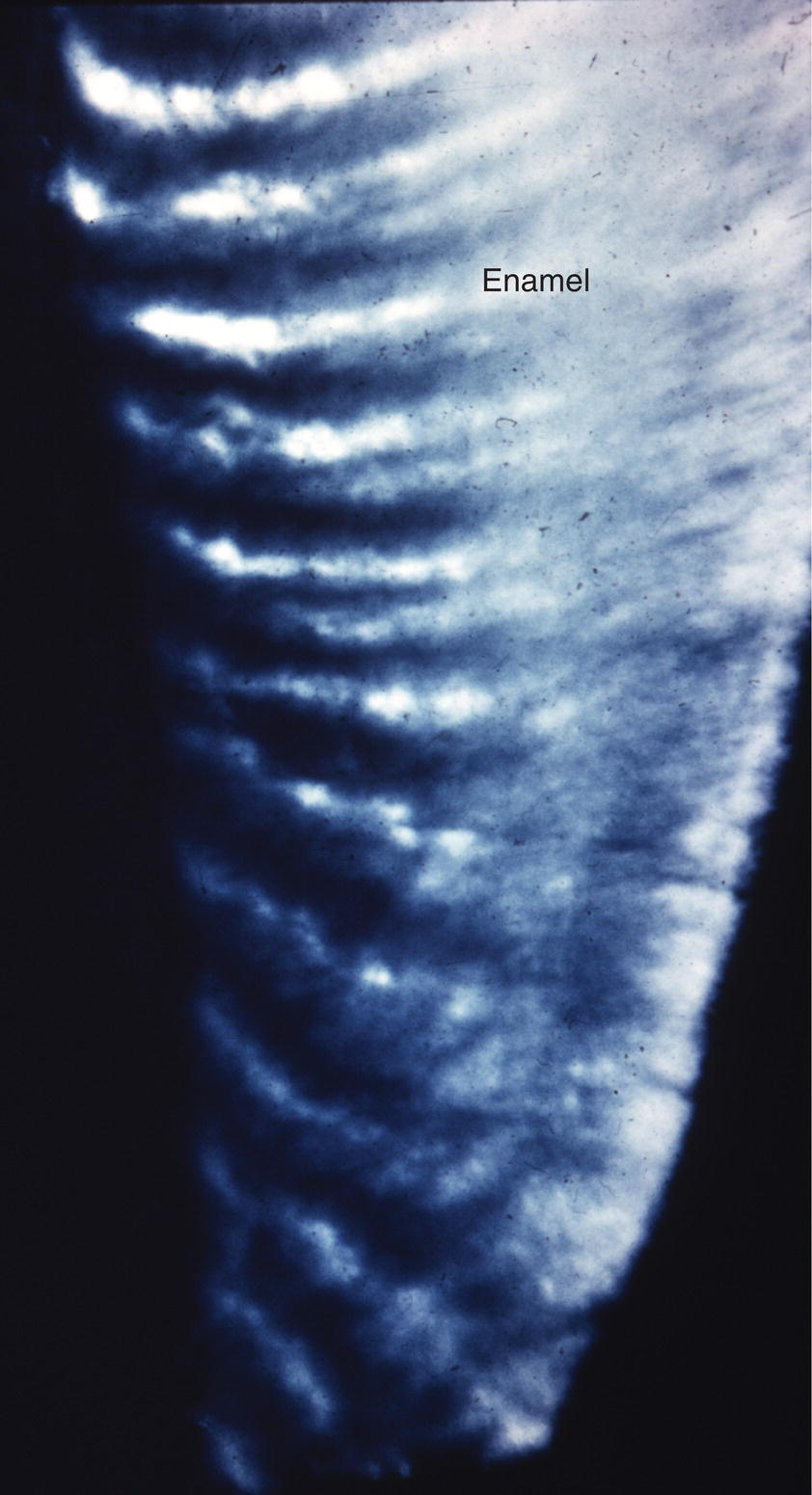
Figure 4.9 Hunter-Schreger bands. The varying direction of the enamel rods results in a group of rods with relatively similar directions adjacent to a group of rods with different directions. In a ground section examined in reflected light, the orientation of the enamel crystals in the rods in one group reflects the light back to the observer, appearing white, whereas light from the adjacent group of rods is reflected away from the observer, and that area appears dark.
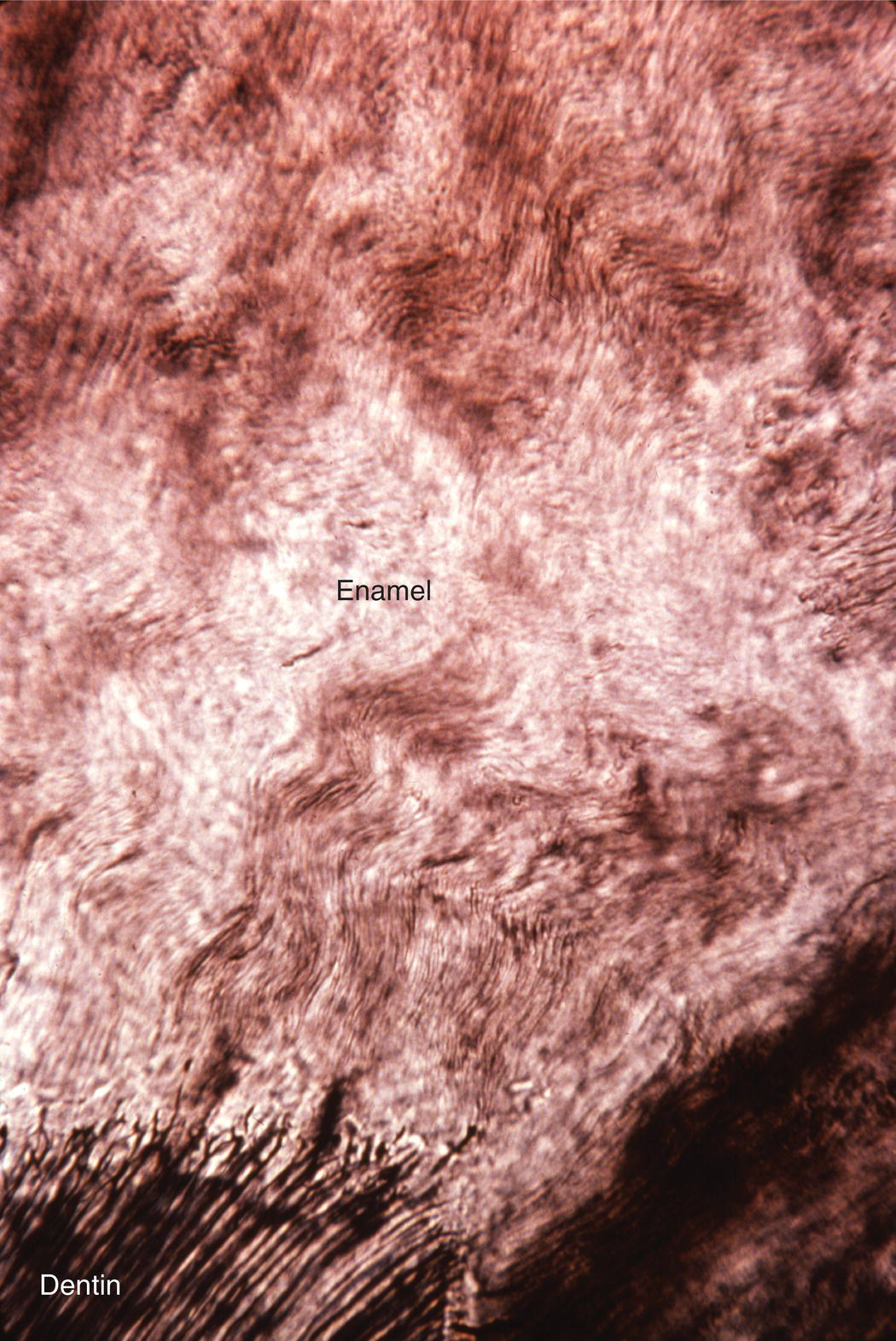
Figure 4.10 Gnarled enamel. In regions of high curvature, such as cusp tips, the varying direction of the enamel rods results in an apparent twisting of the rods around one another.
(From: Moss-Salentijn, L. Orofacial Histology and Embryology: A Visual Integration. Reproduced with permission from F.A. Davis Company, Philadelphia, PA.)
The dentinoenamel junction is the interface between dentin and enamel. It is established when the matrices of these two tissues are first deposited and mineralization begins. This is not a smooth interface; in sections it presents a scalloped profile (Fig. 4.11). The irregular profile of the dentinoenamel junction is thought to increase adherence of the enamel to the dentin, especially in areas of occlusal stress. The region of enamel within a few hundred micrometers of the dentinoenamel junction has a higher content of organic matter, mainly protein, and a lesser hardness than enamel further from the dentinoenamel junction. The different composition of this region results in slightly different physical properties, which are thought to be important in arresting the progression of cracks through enamel and into dentin.
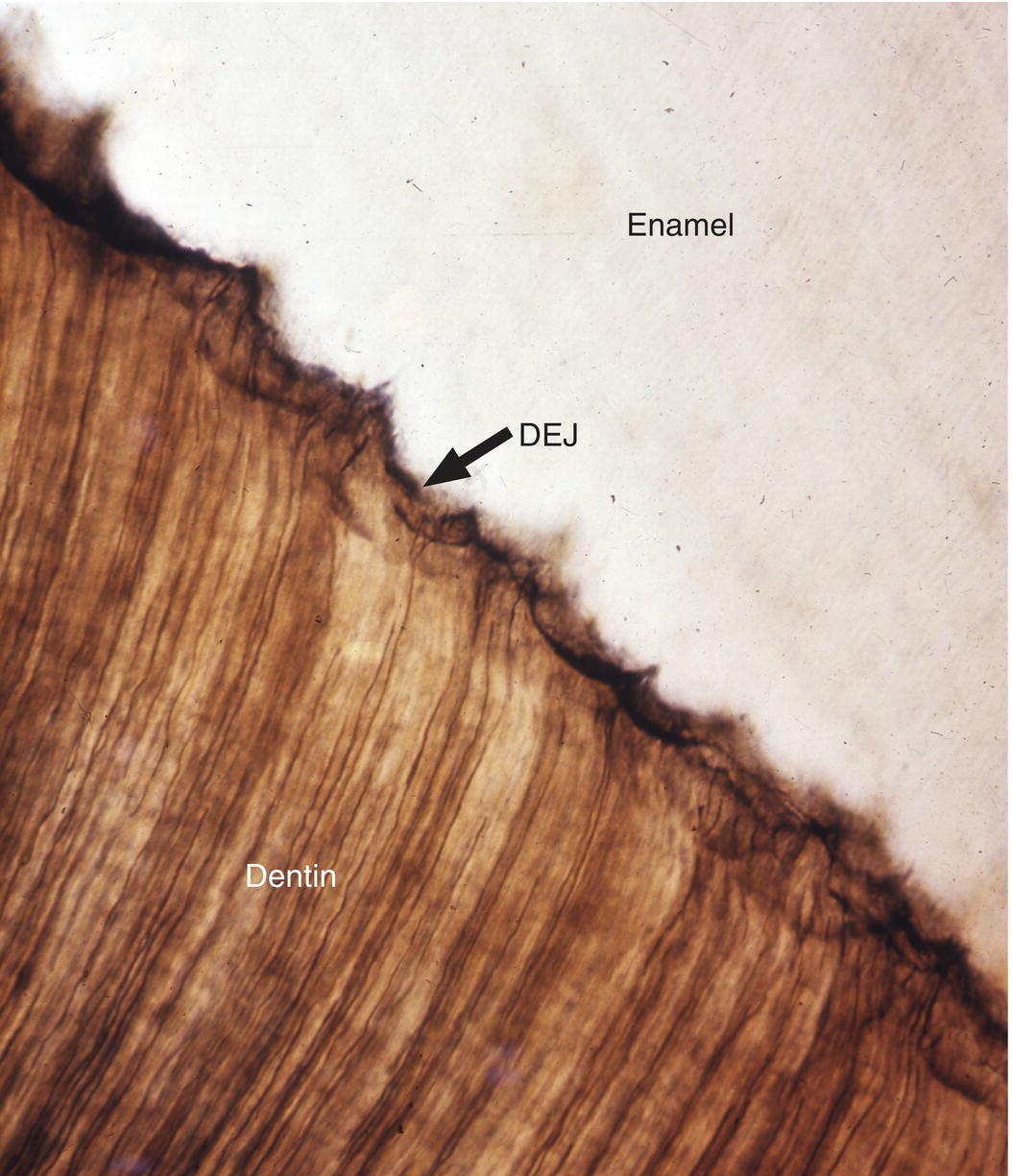
Figure 4.11 Dentinoenamel junction. The dentinoenamel junction (DEJ) exhibits a scalloped outline in sections.
Two structural features of enamel associated with the dentinoenamel junction are frequently observed in ground sections of enamel. Enamel spindles are short extensions of dentinal tubules across the dentinoenamel junction into the enamel (Fig. 4.12). During early dentin formation, prior to the deposition of enamel matrix, odontoblasts may extend their distal process across the future dentinoenamel junction, between adjacent presecretory ameloblasts. When the ameloblasts begin to deposit and partially mineralize the enamel matrix, the odontoblast process is surrounded by matrix and becomes trapped, resulting in an unmineralized space, the enamel spindle. Enamel tufts are bush-like structures that extend from the dentinoenamel junction for a short distance into the innermost enamel (Fig. 4.13). They are hypomineralized regions containing increased residual enamel matrix proteins, thought to be due to changes in direction of adjacent enamel rods originating from different areas of the scalloped dentinoenamel junction.
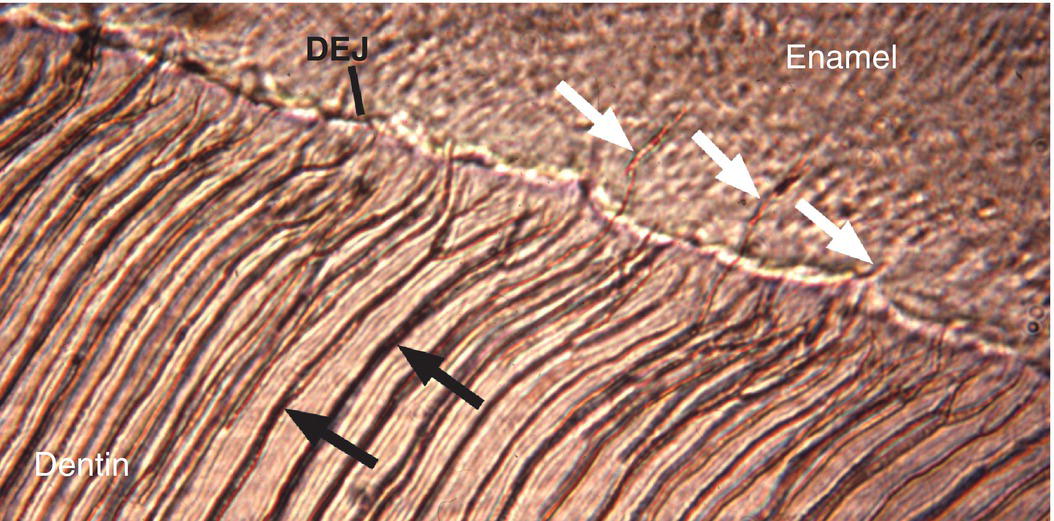
Figure 4.12 Enamel spindles. Enamel spindles (white arrows) are formed when odontoblast processes extend across the dentinoenamel junction (DEJ) and are trapped in the enamel when ameloblasts begin secreting enamel matrix. Dentinal tubules (black arrows).
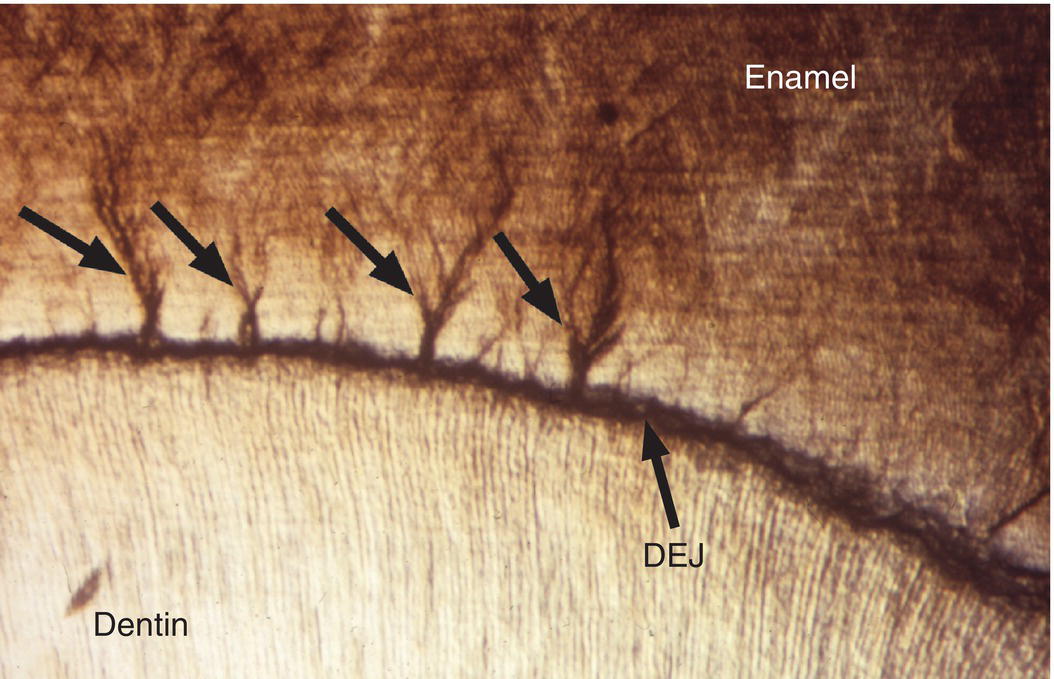
Figure 4.13 Enamel tufts. Enamel tufts are bush-like hypomineralized regions extending from the dentinoenamel junction into the innermost enamel.
Another structural feature of enamel, enamel lamellae, are longitudinal hypomineralized defects that originate at the enamel surface and extend part way or in some cases all the way to the dentinoenamel junction (Fig. 4.14). The lamellae contain an increased amount of organic material, either remnants of the enamel organ or connective tissue that has penetrated into the defect. No clinical significance is presently attributed to either tufts or lamellae.
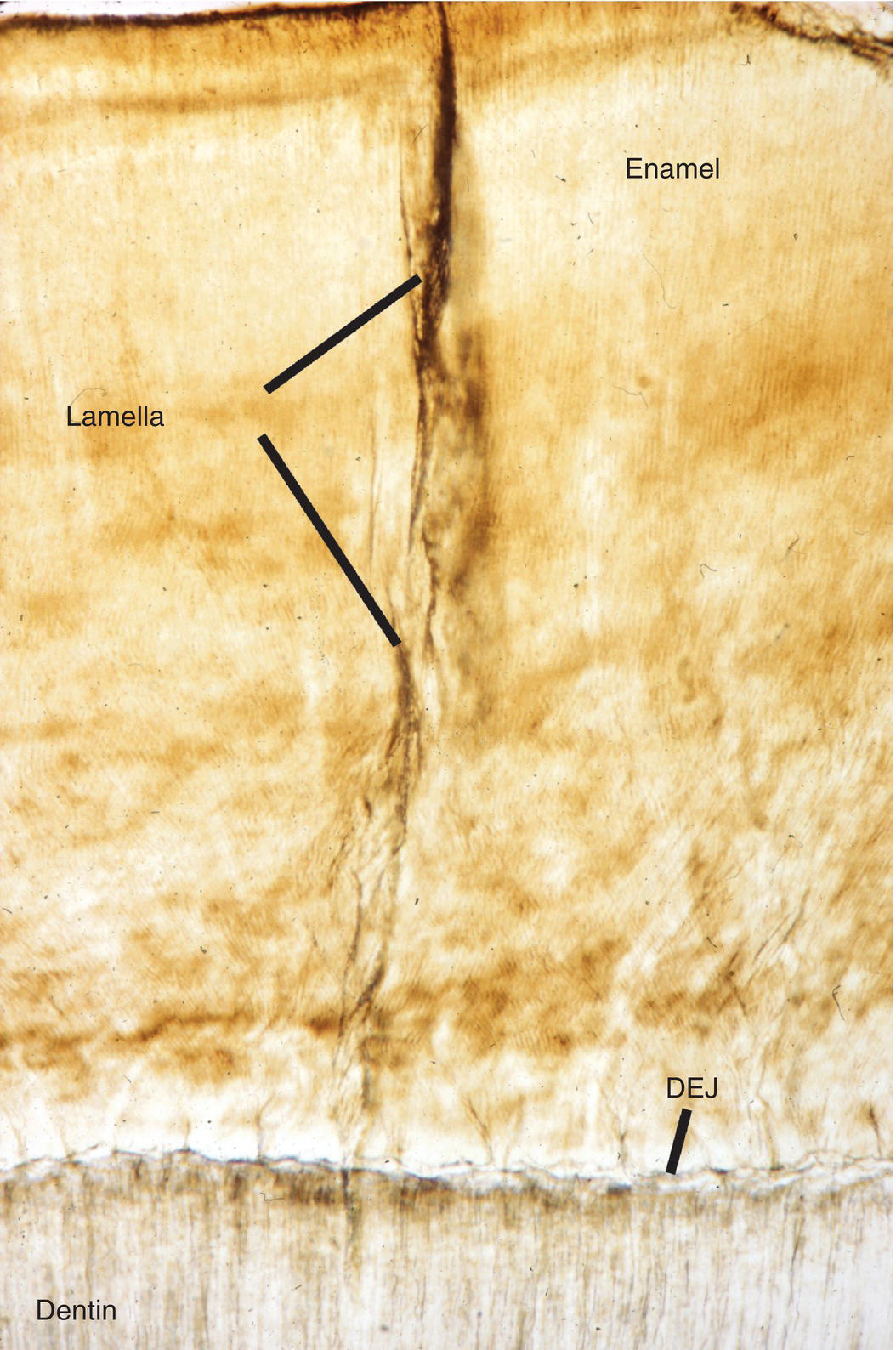
Figure 4.14 Enamel lamella. Enamel lamellae are hypomineralized defects extending from the enamel surface toward the dentinoenamel junction (DEJ).
Enamel formation
The process of enamel formation is called amelogenesis. The formation and mineralization of enamel occurs in stages, beginning at the cusp tips or incisal edges and progressing along the sides of the crown toward the cervical margin (Fig. 4.15). The cells that form the enamel are ameloblasts, which differentiate from the inner enamel epithelium of the enamel organ, as described in Chapter 3. The stages of amelogenesis (Fig. 4.16) are:
- presecretory, when the cells prepare to begin enamel matrix formation;
- secretory, during which the ameloblasts deposit and initially mineralize the full thickness of the enamel matrix;
- transition, a short stage in which the cells prepare to become maturation ameloblasts;
- maturation, during which the ameloblasts complete the removal of matrix proteins and the addition of mineral; and
- protective, during which the ameloblasts cover the enamel surface until the tooth erupts.
The function as well as the morphology of the ameloblasts changes as they progress through each stage.
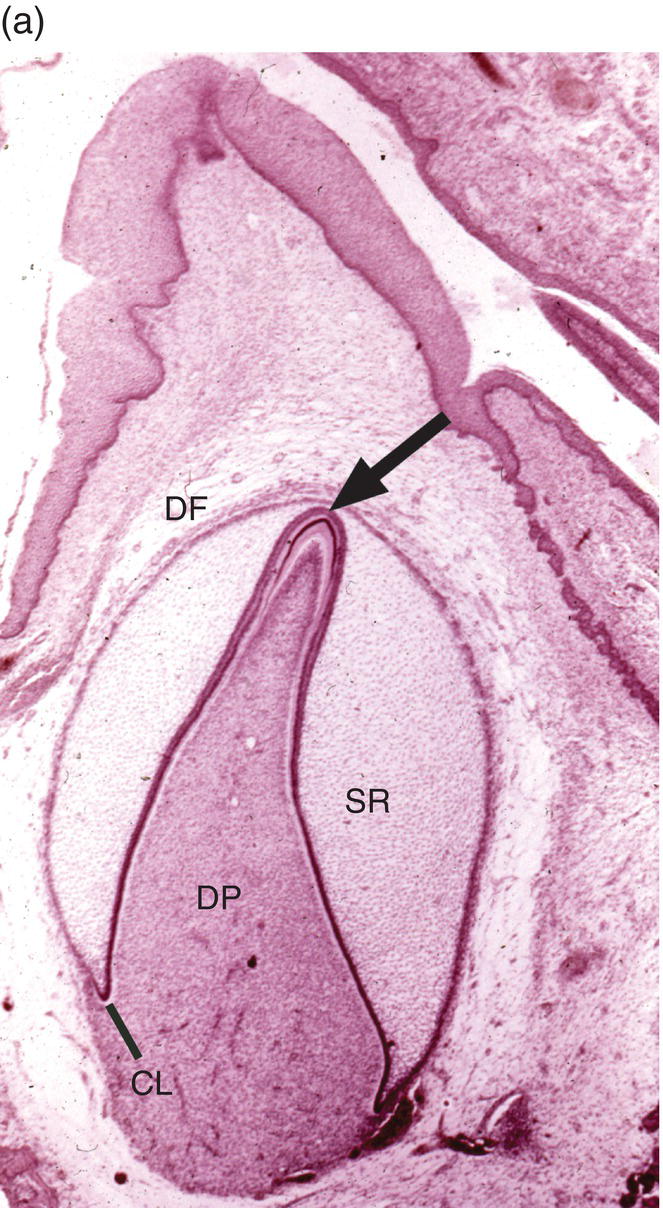
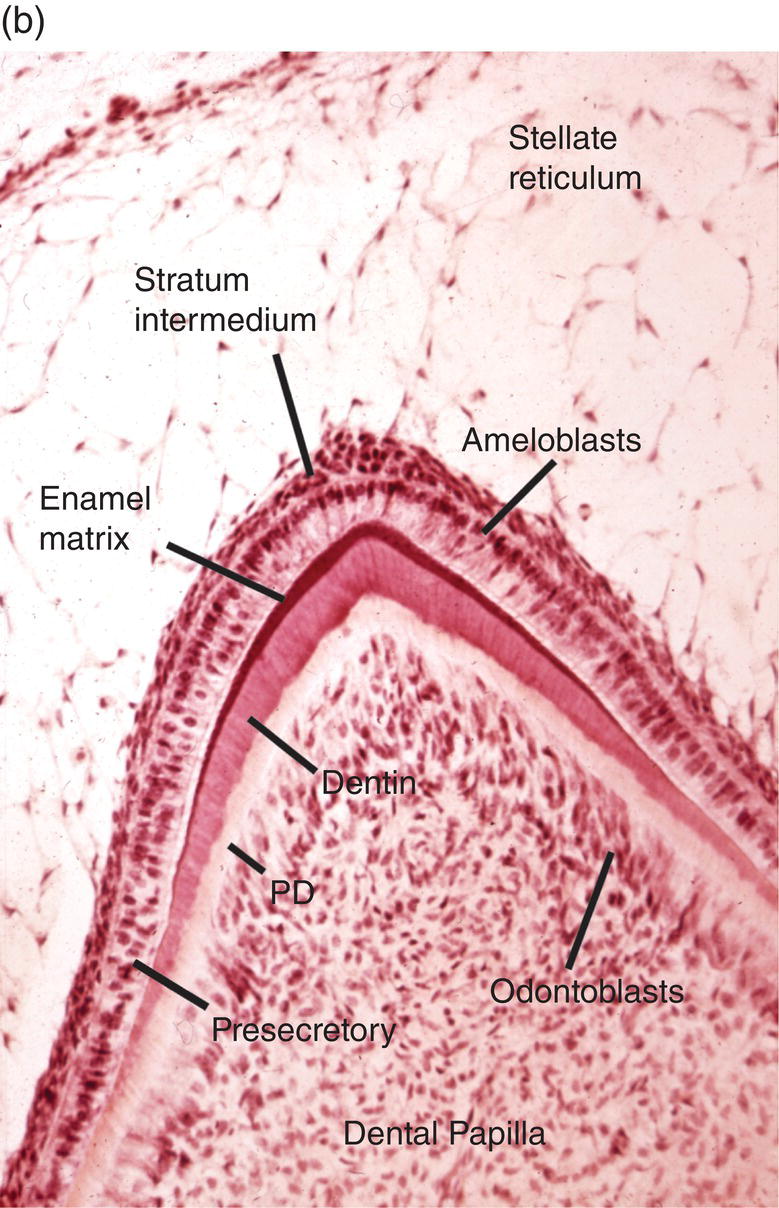
Figure 4.15 Enamel formation. (a) Low magnification micrograph of a developing incisor in the early crown stage, with deposition of enamel and dentin matrices at the cusp tip (arrow). Cervical loop (CL), dental follicle (DF), dental papilla (DP), stellate reticulum (SR). (b) Higher magnification of a similar developing cusp tip. Secretory ameloblasts have differentiated at the cusp tip and have deposited a thin layer of enamel matrix on the outer surface of the dentin. Along the sides of the cusp, closer to the cervical loop, the ameloblasts are in the presecretory stage of differentiation, and at the lower left inner enamel epithelial cells are seen. Predentin (PD).
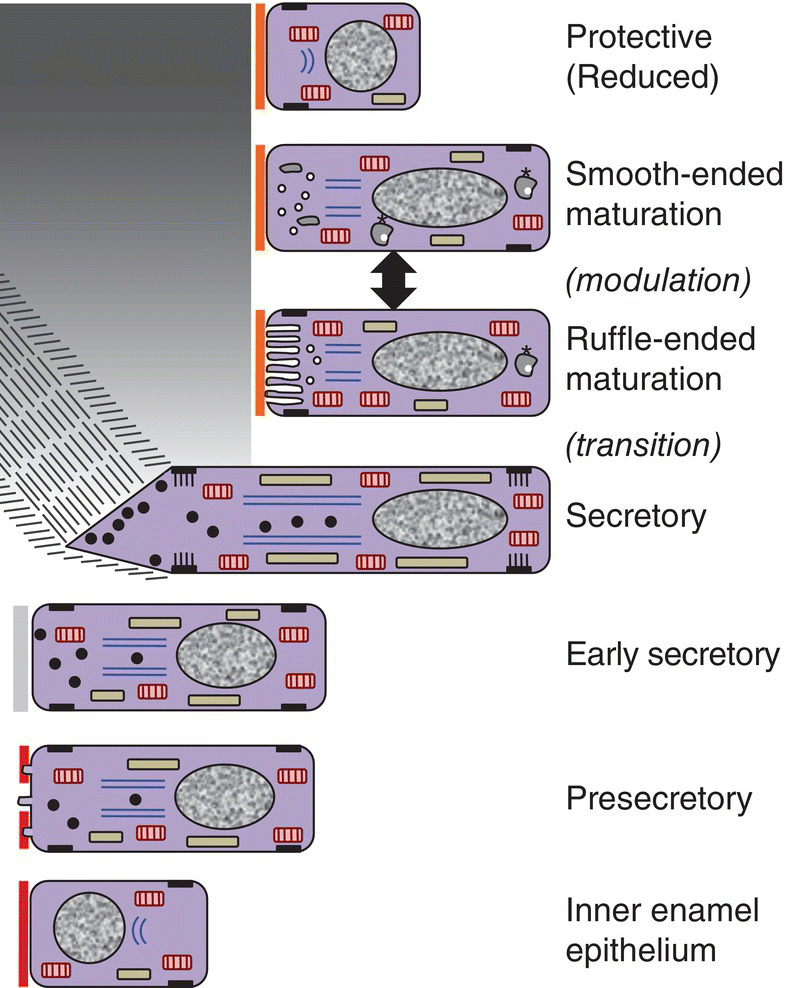
Figure 4.16 Drawing of amelogenesis stages. Inner enamel epithelial cells are separated from the dental papilla mesenchymal cells by a basal lamina (red line). Their nuclei are located basally, and apical junctional complexes hold the cells together. The inner enamel epithelial cells induce the peripheral cells of the dental papilla to differentiate into odontoblasts. Under the influence of the odontoblasts, inner enamel epithelial cells differentiate into presecretory ameloblasts. The cells elongate and the nuclei move to the former apical end of the cells, now called the proximal end. The Golgi complexes (blue lines) increase in size and relocate between the nuclei and the former basal end of the cells, now called the distal end, and the amount of rough endoplasmic reticulum (elongated beige organelles) increases. Junctional complexes develop at the new distal end of the cells, small cellular protrusions penetrate the basal lamina, and the basal lamina begins to disintegrate. As predentin begins to mineralize, the presecretory ameloblasts become early secretory ameloblasts and begin to synthesize large amounts of enamel matrix proteins and deposit them onto the newly formed mantle dentin, creating the dentino-enamel junction. Partial mineralization of the matrix occurs as it is deposited. This initial immature enamel is unstructured, lacking the typical rod-interrod structure of mature enamel. As the ameloblasts deposit and partially mineralize additional enamel matrix and retreat from the dentinoenamel junction, the cells further elongate, and Tomes’ processes form at the distal end of the cells. The cells are now fully differentiated secretory ameloblasts. Tomes’ processes create the rod-interrod stucture of enamel; rods form from enamel matrix released at the tip of Tomes’ processes, and interrod enamel forms along the sides of Tomes’ processes. After the full thickness of enamel matrix is deposited, the ameloblasts undergo a transition (not shown) to become maturation ameloblasts. The cells shorten, much of their synthetic machinery is degraded, a new basal lamina (orange line) is deposited, and about 25% of the cells die by apoptosis. Maturation ameloblasts modulate their morphology between ruffle-ended and smooth-ended forms. Ruffle-ended ameloblasts add mineral to the immature enamel, whereas smooth-ended ameloblasts allow removal of degraded matrix proteins and water. During maturation, an additional 25% of the cells die. Once mineralization is complete, the ameloblasts again shorten and become protective (reduced) ameloblasts.
Presecretory stage
During the presecretory stage, the inner enamel epithelial cells induce the peripheral cells of the dental papilla to differentiate into odontoblasts. Shortly after that, the inner enamel epithelial cells begin their differentiation to ameloblasts (Figs. 4.16, 4.17). The cells elongate, the nucleus relocates to what was the apical end of the cell (now called the proximal end), the Golgi complex migrates to the supranuclear cytoplasm, between the nucleus and the former basal end of the cell (now called the distal end), and the rough endoplasmic reticulum increases in amount and forms a cylindrical sleeve around the Golgi complex. The junctional complexes are maintained at the proximal end of the cell, and new junctional complexes are formed at the distal end. These cells are now called presecretory ameloblasts; they have not yet begun to secrete enamel matrix. The distal cell membrane of the presecretory ameloblasts has a relatively smooth outline, and the basal lamina between these cells and the early odontoblasts is still intact (Fig. 4.18a).
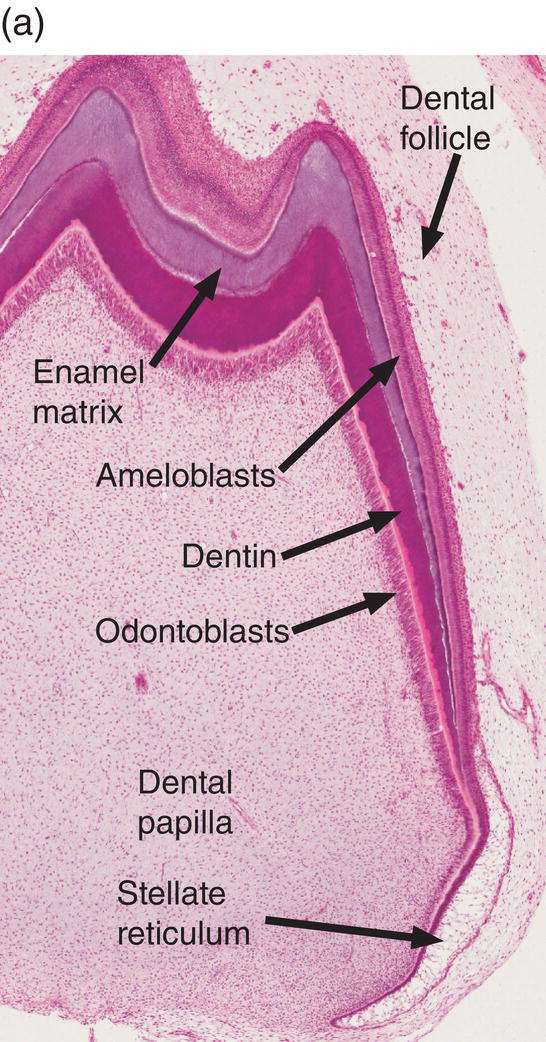
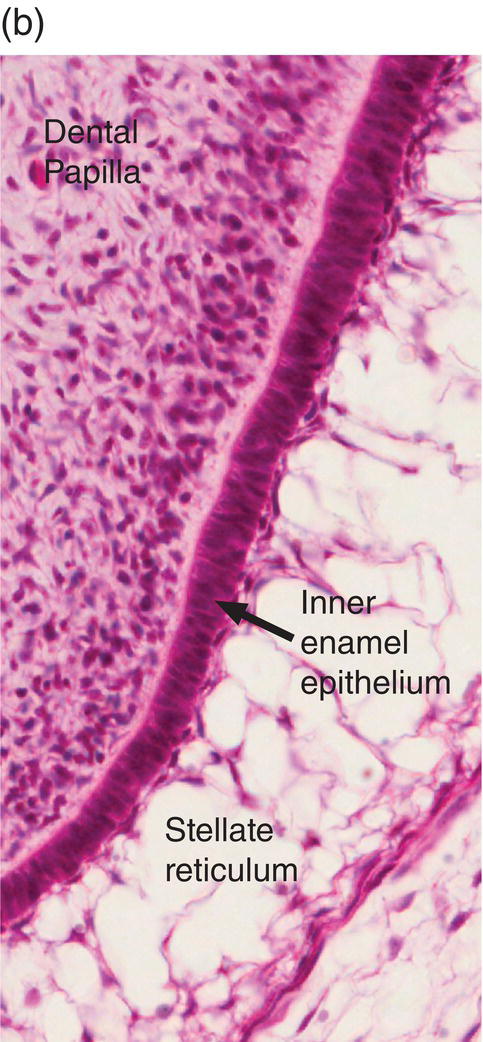
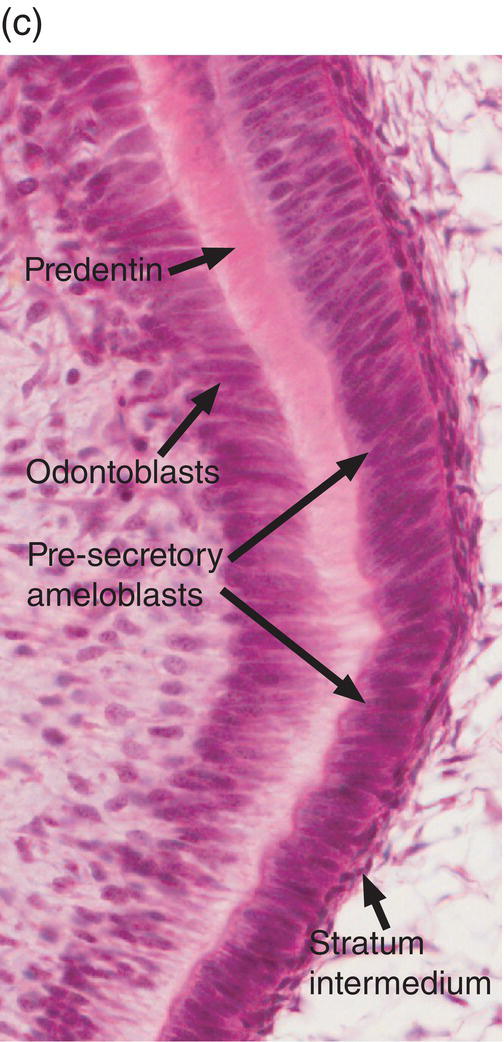
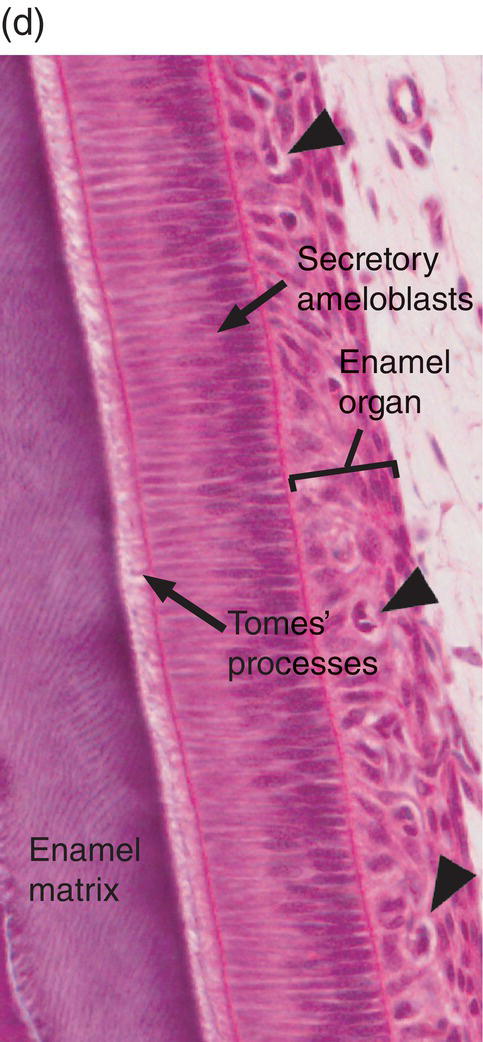
Figure 4.17 Ameloblast differentiation. (a) Low magnification micrograph showing part of the crown of a developing molar tooth. Dentin and enamel matrix formation is underway. (b) Higher magnification showing part of the dental papilla, inner enamel epithelium, and stellate reticulum. Near the top of the image, the peripheral cells of the dental papilla are just beginning to differentiate into odontoblasts, and the inner enamel epithelial cells are beginning to elongate. (c) The inner enamel epithelial cells are elongating and are now presecretory ameloblasts. Odontoblast differentiation is occurring in the lower third of the image, and just below the middle of the image predentin secretion has begun. (d) Tall secretory stage ameloblasts with Tomes’ processes and nuclei located proximally or at a mid-cytoplasmic level. Evidence of the rod pattern can be seen in the enamel matrix. The stellate reticulum has collapsed and the enamel organ forms a compact layer adjacent to the ameloblasts. Blood vessels (arrowheads) from the dental follicle have penetrated the enamel organ.
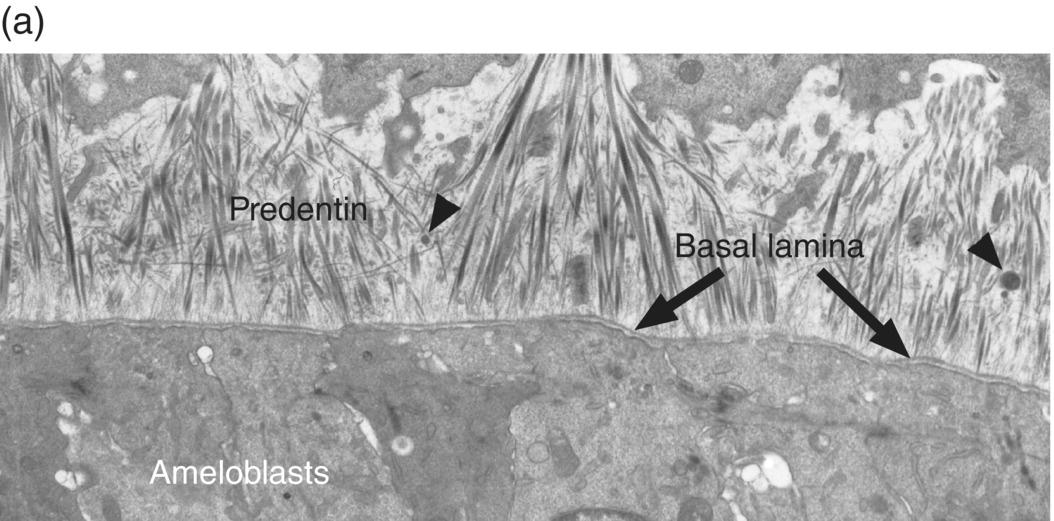
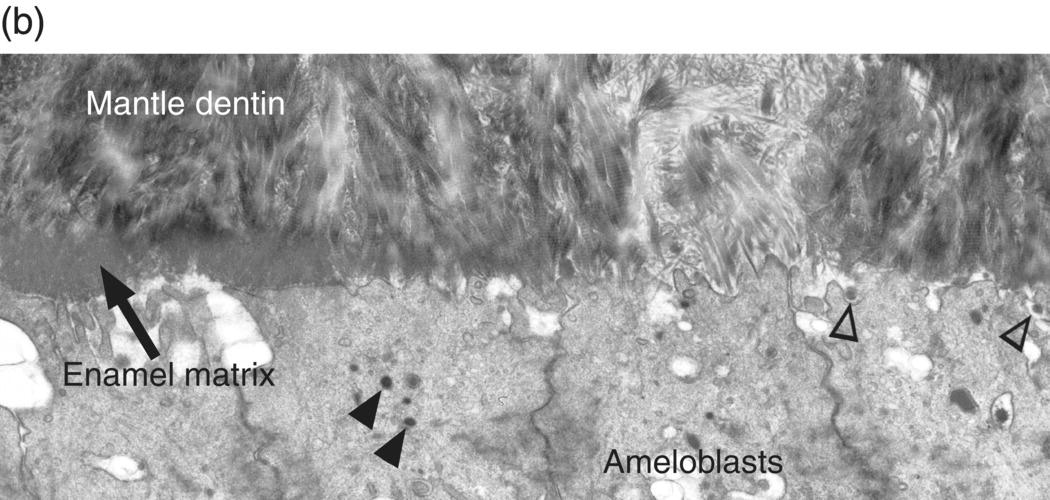
Figure 4.18 Electron micrographs of presecretory and early secretory ameloblasts. (a) Predentin of the mantle layer is deposited prior to enamel matrix secretion. The distal ends of odontoblasts are visible at the top, and matrix vesicles (arrowheads) are present in the predentin. The presecretory ameloblasts have a relatively smooth distal surface with an intact basal lamina. (b) The basal lamina has disappeared, and small projections of the distal end of the ameloblasts are seen at the right. These cells are just beginning to release enamel matrix (open arrowheads). A few secretory granules (arrowheads) containing enamel proteins are seen in the early secretory ameloblasts, and at the left a thin layer of initial enamel matrix has been deposited.
(Courtesy of V.-L. Ferrer and A. Lichter, University of Connecticut School of Dental Medicine.)
Secretory stage
Prior to the initial secretion of enamel proteins, the basal lamina disintegrates and disappears, and the distal ends of the presecretory ameloblasts develop small projections (Figs. 4.16, 4.18b). The cells increase their synthesis of enamel matrix proteins, package them in small secretory granules in the Golgi complex (Fig. 4.19), and release them by exocytosis from the distal ends of the cells, adjacent to the dentin. At this point the cells are early secretory ameloblasts (Fig. 4.16). The initial enamel matrix and forming crystals are closely integrated with the matrix and mineral of dentin. As more enamel matrix is deposited, the distal membrane of the ameloblast again becomes smooth. This early enamel adjacent to the dentin is structureless, i.e., the rod-interrod structure described earlier has not yet been established. As the ameloblasts deposit more enamel matrix and continue to move away from the dentinoenamel junction, an elongated process, Tomes’ process, is formed on the distal end of each cell. The cells are now fully differentiated secretory ameloblasts (Figs. 4.16, 4.20, 4.21). Release of matrix proteins from secretory granules at the tip of Tomes’ process creates the enamel rod, which is oriented at an approximately 45° angle to the ameloblast cell body (Fig. 4.22). The matrix proteins of the interrod enamel are released along the sides of Tomes’ processes, nearer the junctional complexes between adjacent cells. Thus, the rod is created by a single ameloblast, whereas the interrod enamel is created by that ameloblast and all of its immediate neighbors (Figs. 4.22, 4.23). As the cells retreat from the dentinoenamel junction and deposit additional enamel matrix, the interrod enamel is formed first, then the rod enamel is deposited, filling up the spaces formerly occupied by Tomes’ processes (Fig. 4.24). The secretion of the rod and interrod enamel at two different sites on the ameloblast is responsible for the differing />
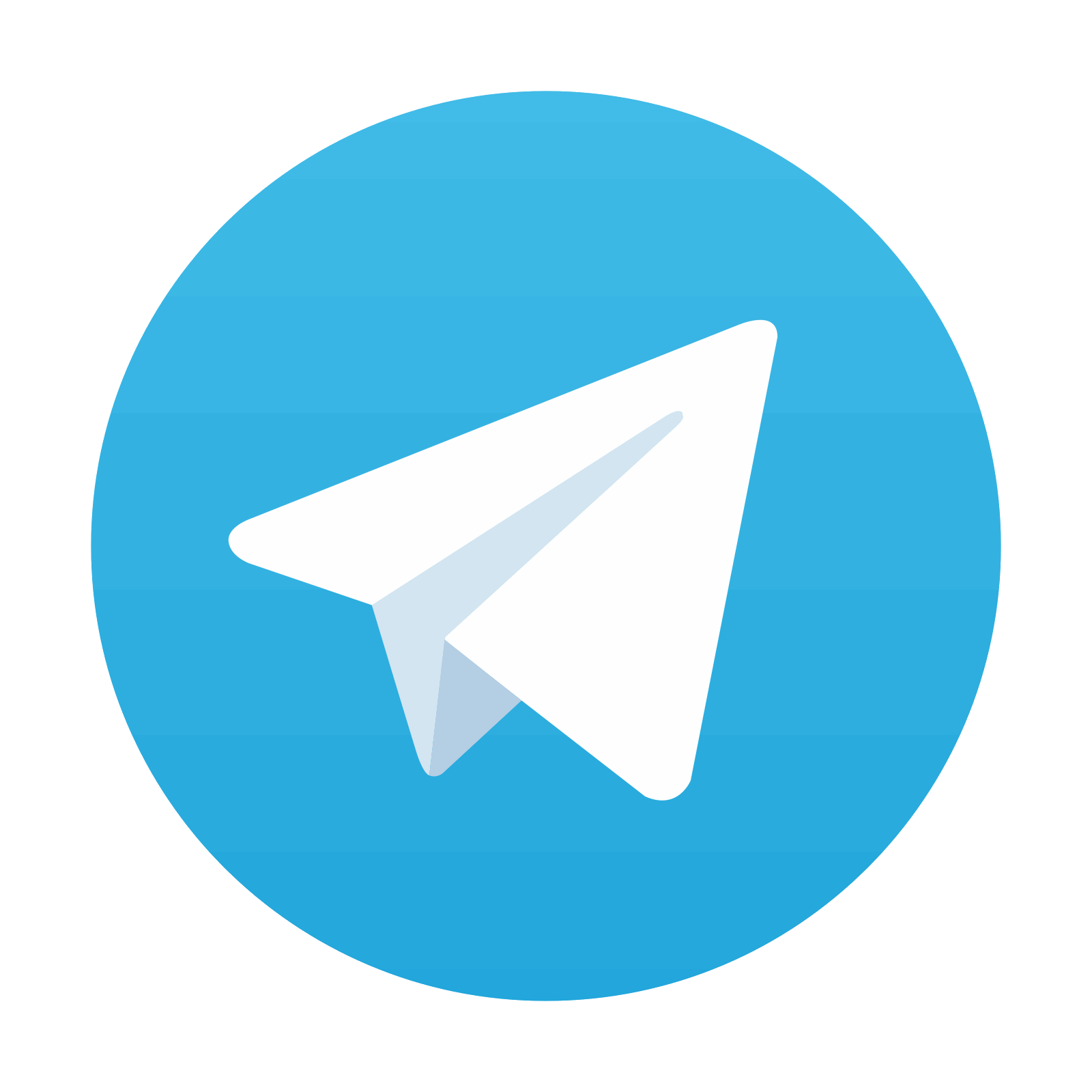
Stay updated, free dental videos. Join our Telegram channel

VIDEdental - Online dental courses
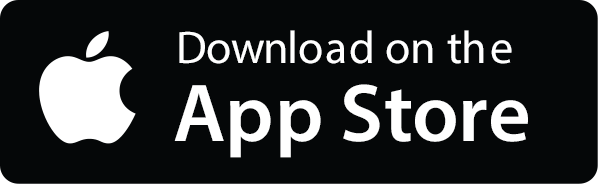
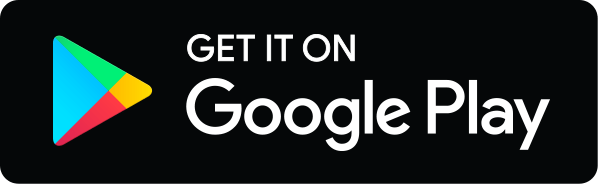