Structure of Matter and Principles of Adhesion
Around 460 B.C., the Greek philosopher Democritus proposed that all matter was composed of indivisible particles called átomos (á = “un”; temno = “to cut”; meaning “uncuttable”), which is the origin of the name atoms. We know that an atom consists of a nucleus surrounded by a cloud of negatively charged electrons, as depicted in the electron cloud model of an atom (Figure 2-1). Except for the hydrogen atom, where there are no neutrons, the nucleus contains a mix of positively charged protons and electrically neutral neutrons. The electrons of an atom exist in different clouds at the various energy levels. An atom becomes a negative ion when it gains electron(s) or a positive ion when it loses electron(s).
Interatomic Bonds
Primary Bonds
The formation of primary bonds depends on the atomic structures and their tendency to assume a stable configuration. The strength of these bonds and their ability to reform after breakage determine the physical properties of a material. Primary atomic bonds (Figure 2-2), also called chemical bonds, may be of three different types: (1) ionic, (2) covalent, and (3) metallic.
Ionic Bonds
The classic example of ionic bonding is the bond between the Na+ and Cl– of sodium chloride (Figure 2-2, A). Because the sodium atom contains one valence electron in its outer shell and the chlorine atom has seven electrons in its outer shell, the transfer of the sodium valence electron to the chlorine atom results in the stable compound Na+Cl–. In dentistry, ionic bonding exists in some dental materials, such as in gypsum structures and phosphate-based cements.
Covalent Bonds
In many chemical compounds, two valence electrons are shared by adjacent atoms (Figure 2-2, B). By virtue of sharing electrons, the two atoms are held together by covalent bonds to form a molecule that is sufficiently stable, and electrically neutral in a definite arrangement. The hydrogen molecule, H2, exemplifies covalent bonding. The single valence electron in each hydrogen atom is shared with that of the other combining atom, and the valence shells become stable. Covalent bonding occurs in many organic compounds, such as in dental resins, where they link to form the backbone structure of hydrocarbon chains (Chapter 6).
Metallic Bonds
The third type of primary atomic interaction is the metallic bond (Figure 2-2, C). The outer shell valence electrons can be removed easily from metallic atoms and form positive ions. The free valence electrons can move about in the metal space lattice (Chapter 5) to form what is sometimes described as an electron “cloud” or “gas.” The electrostatic attraction between the electron cloud and the positive ions in the lattice provides the force that bonds the metal atoms together as a solid.
Combination of Primary Bonds
Although we can describe the three primary bonds separately, it is also possible to find more than one type of primary bond existing in one material. Consider calcium sulfate (CaSO4), the main ingredient of gypsum products (Chapter 9), as an example (Figure 2-3). In the sulfate ion (SO42−) the sulfur and oxygen atoms are held together covalently but they are short of two electrons. Calcium has two electrons in the outer orbit, which are easily removed and transferred to the SO4. The result is a Ca2+ ion with attraction for an SO42− ion.
Secondary Bonds
van der Waals Forces
These van der Waals forces of attraction arise from dipole attractions (Figure 2-4). In the case of polar molecules, dipoles are induced by an unequal sharing of electrons (Figure 2-4, A). In the case of nonpolar molecules, random movement of electrons within the molecule creates fluctuating dipoles (Figure 2-4, B). Dipoles generated within these molecules will attract other similar dipoles. Such interatomic forces are quite weak compared with the primary bonds.
Hydrogen Bond
The hydrogen bond is a special case of dipole attraction of polar compounds. It can be understood by studying a water molecule (Figure 2-5). Attached to the oxygen atom are two hydrogen atoms. These bonds are covalent. As a consequence, the protons of the hydrogen atoms pointing away from the oxygen atom are not shielded efficiently by the electrons. They become positively charged. On the opposite side of the water molecule, the electrons that fill the outer shell of the oxygen provide a negative charge. The positive hydrogen nucleus is attracted to the unshared electrons of neighboring water molecules. This type of bond is called a hydrogen bridge. Polarity of this nature is important in accounting for the intermolecular reactions in many organic compounds—for example, the sorption of water by synthetic dental resins.
Atomic Arrangement
In the solid state, atoms combine in a manner that ensures minimal internal energy. For example, sodium and chlorine share one electron at the atomic scale. In the solid state, like grains of salt, they do not exist in individual pairs; in fact, each sodium ion is attracted to six chlorine ions and vice versa (Figure 2-6). They form a regularly spaced configuration (long-range repetitive space lattice) known as a crystal. A space lattice can be defined as any arrangement of atoms in space in which every atom is situated similarly to every other atom.
Crystalline Structure
There are 14 possible lattice types. The type of space lattice is defined by the length of each of three unit cell edges (called the axes) and the angles between the edges. The simplest and most regular lattice is a cubic, as shown in Figure 2-7, A; it is characterized by axes that are all of equal length and meet at 90-degree angles, representing the smallest repetitive volume of a crystal, which is called a unit cell. Each sphere represents the positions of the atoms. Their positions are located at the points of intersection of three planes, each plane (surface of the cube) being perpendicular to the other two planes. These planes are often referred to as crystal planes. However, the simple cubic arrangement shown in Figure 2-7, A, is hypothetical, as it leaves enough space to fit additional atoms per unit cell. Most crystalline lattices of atoms also contain sites of missing atoms. Each missing atom site is called a vacancy.
Most metals used in dentistry belong to the cubic system. For example, iron at room temperature has an atom at each corner of the cube and another atom at the body center of the cube (Figure 2-7, B). This crystal form is called a body-centered cubic cell. Copper, on the other hand, has additional atoms at the center of each face of the unit cell but none at the center of the cube. This form is called a face-centered cubic cell (Figure 2-7, C).
Other types of space lattices of dental interest are shown in Figure 2-8. The hexagonal close-packed arrangement (Figure 2-8, G) observed in titanium, zinc, and zirconium has become an important crystalline structure in dentistry. Note that each unit cell consists of three layers of atoms.
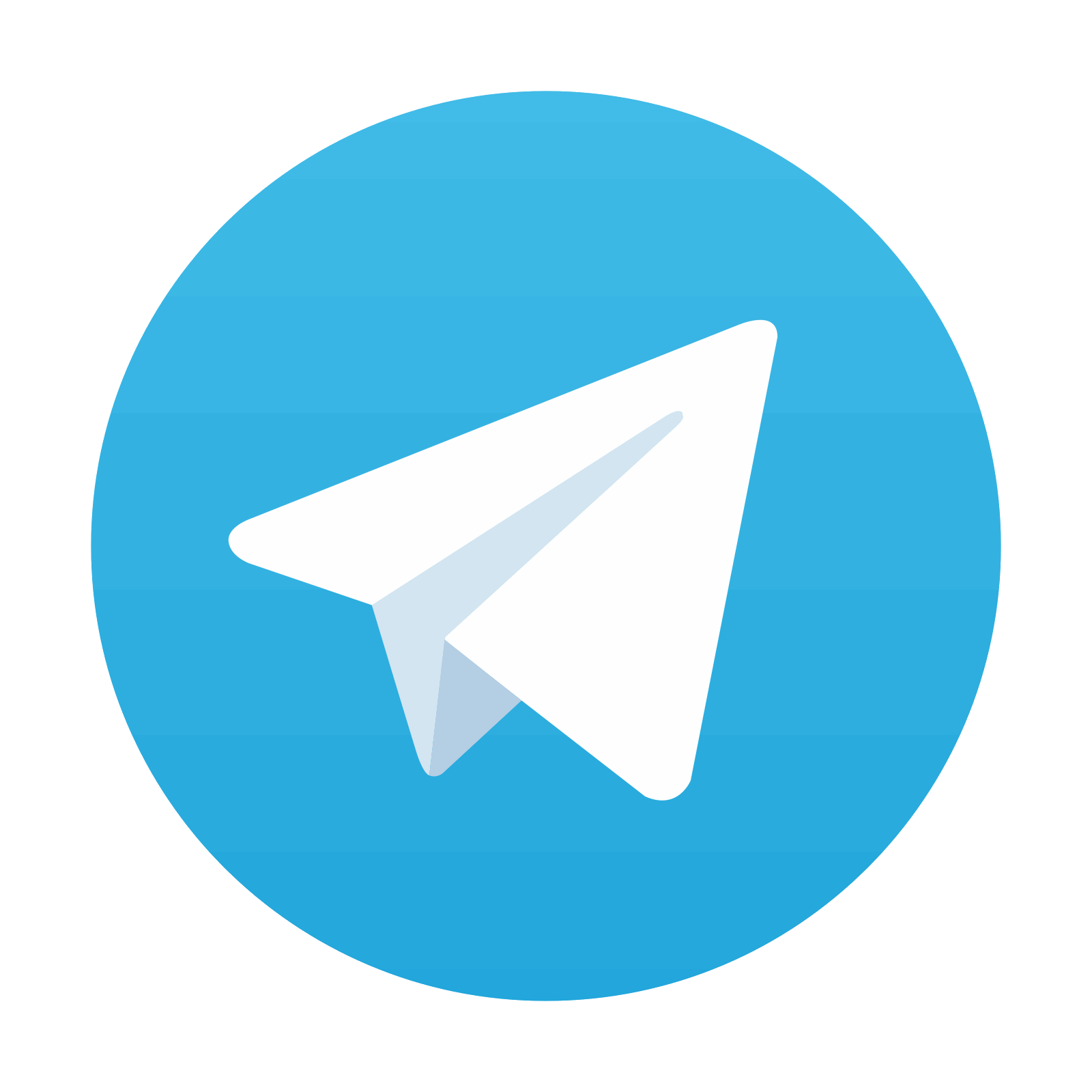
Stay updated, free dental videos. Join our Telegram channel

VIDEdental - Online dental courses
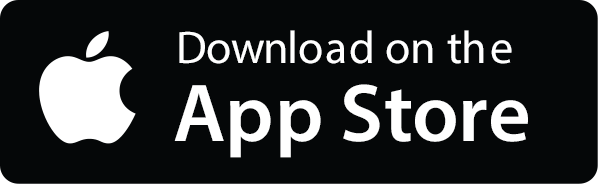
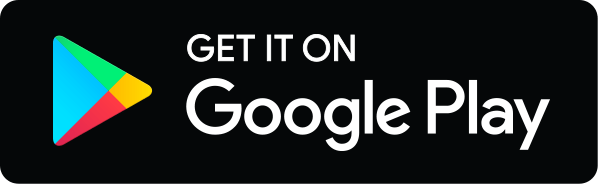