CHAPTER 2
Review of Bone-Grafting Materials
Although alveolar bone can be a contraindication for dental implants, bone grafting can provide the structural or functional support necessary in such cases. Grafts can provide scaffolding (Fig 2-1) for bone regeneration1 and augmentation for bony defects resulting from trauma, pathology, or surgery. They can also be used to restore bone loss resulting from dental disease; to fill extraction sites; and to preserve the height and width of the alveolar ridge through augmentation and reconstruction. Autogenous bone remains the best grafting material because of its osteogenic properties, which allow bone to form more rapidly in conditions that require significant bone augmentation or repair. The allografts most commonly used for restoring osseous defects are mineralized or demineralized freeze-dried bone allografts (FDBA). The primary alloplasts are hydroxyapatite, bioactive glasses, tricalcium phosphate (TCP) particulates, and synthetic polymers. The primary xenograft material is purified anorganic bone, either alone or enhanced with tissue-engineered molecules. These augmentation materials can be incorporated in the modeling, remodeling, or healing processes of bone to assist or to stimulate bone growth in areas where resorption has occurred and implants are needed.
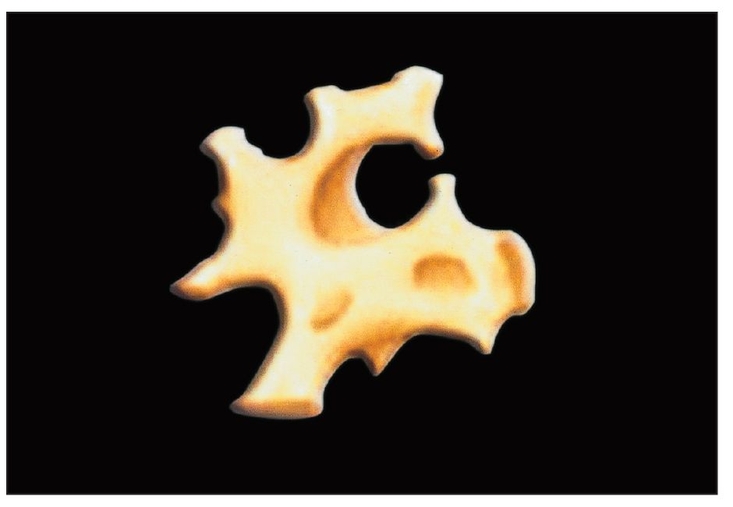
Bone-grafting materials provide a resorbing scaffold that permits osseous tissue ingrowth. Ideally, resorption is gradual enough to allow the surrounding bony tissues to occupy the recipient site completely, while still allowing dental implants to be placed in new osseous tissue as quickly as possible.
Mechanisms of Bone Regeneration and Augmentation
Three different processes are associated with successful bone grafting: osteogenesis, osteoinduction, and osteoconduction. 2, 3, 4, 5 Osteogenesis is the formation and development of bone. An osteogenic graft is derived from or composed of tissue involved in the natural growth or repair of bone. Osteogenic cells can encourage bone formation in soft tissues or activate more rapid bone growth in bone sites. Osteoinduction is the process of stimulating osteogenesis. Osteoinductive grafts can be used to enhance bone regeneration and may even cause bone to grow or extend into an area where it is not normally found. Osteoconduction provides a physical matrix or scaffolding suitable for the deposition of new bone. Osteoconductive grafts are conducive to bone growth and allow bone apposition from existing bone, but they do not produce bone formation themselves when placed within soft tissue. To encourage bone growth across its surface, an osteoconductive graft requires the presence of existing bone or differentiated mesenchymal cells. All bone-grafting materials possess at least one of these three modes of action.
Types of Graft Material
As noted above, the three primary types of bone graft material are autogenous bone; allografts; and alloplasts, of which commercially available xenografts are generally considered a subgroup. The mechanism by which these graft materials work normally depends on the origin and composition of the material.3, 6 Autogenous bone, an organic material harvested from the patient, forms new bone by osteogenesis, osteoinduction, and osteoconduction. Harvested from cadavers, allografts, which may be cortical or trabecular, have osteoconductive and possibly osteoinductive properties, but they are not osteogenic. Alloplasts, which may be composed of natural or synthetic material, are typically only osteoconductive.
In determining what type of graft material to use, the clinician must consider the characteristics of the bony defect to be restored. 3 In general, the larger the defect, the greater the amount of autogenous bone required. For small defects and for those with three to five bony walls still intact, alloplasts may be used alone or with allografts. For relatively large defects or those with only one to three bony walls intact, autogenous bone must be added to any other type of graft material being considered. Soft tissue ingrowth can be a complication during augmentation procedures with any grafting materials, so guided bone regeneration (GBR) using resorbable or nonresorbable membranes is often employed.7
Autogenous Bone
Autogenous bone, long considered the gold standard of grafting materials, is currently the only osteogenic graft material available to clinical practitioners. Grafted autogenous bone heals into growing bone through all three modes of bone formation; these stages are not separate and distinct, but rather overlap each other.3 Common areas from which autogenous bone can be harvested include extraoral sites such as the iliac crest or tibial plateau and intraoral sites such as the mandibular symphysis, maxillary tuberosity, ramus, or exostoses.3, 8, 9 Less resorption has been associated with the use of mandibular bone grafts than with iliac crest grafts.8 Resorption may be reduced during healing by the use of expanded polytetrafluoroethylene (e-PTFE) membranes or slowly resorbable collagen membranes.10 Bone grafts obtained intraorally generally result in less morbidity; however, intraoral donor sites provide a significantly smaller volume of bone than do extraoral sites such as the iliac crest or tibial plateau.
The optimal donor site depends on the volume and type of regenerated bone needed for the specific case. The posterior iliac crest provides the greatest amount of bone—up to 140 mL (Table 2-1 and Fig 2-2). This compares to up to 70 mL from the anterior iliac crest, 20 to 40 mL from the tibial plateau (Fig 2-3), 5 to 10 mL from the ascending ramus, up to 5 mL from the anterior mandible (Fig 2-4), up to 2 mL from the tuberosity, and varying amounts from bone shavings (Fig 2-5) or exostoses or through the use of suction traps (Fig 2-6). Autogenous bone is highly osteogenic and best fulfills the dental grafting requirements of providing a scaffold for bone regeneration. 11 The disadvantages associated with the use of autogenous bone are the need for a second operative site, resultant patient morbidity, and in some cases the difficulty of obtaining a sufficient amount of graft material (especially from intraoral sites). These limitations led to the development of allografts and alloplasts as alternative grafting materials.2, 11 ,12
Graft form and maximum volume available from autogenous bone donor sites
Donor site | Form available | Maximum volume (mL) |
---|---|---|
Extraoral | ||
Posterior iliac crest | Block and/or particulate | 140 |
Anterior iliac crest | Block and/or particulate | 70 |
Tibia | Particulate | 20 to 40 |
Cranium | Dense cortical block | 40 |
Intraoral | ||
Ascending ramus | Block | 5 to 10 |
Anterior mandible | Block and/or particulate | 5 |
Tuberosity | Particulate | 2 |
Miscellaneous (eg, bone shavings, suction traps) | Particulate | Varies |
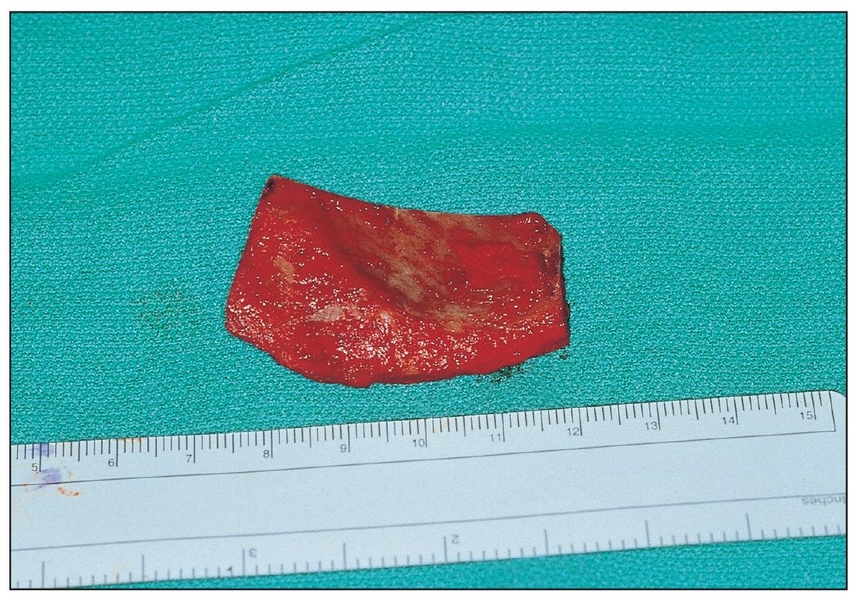
The ilium provides a greater amount of bone than do other donor sites, making it ideal when 50 mL or more is required. Harvesting is performed in a hospital operating room under general anesthesia. The harvested block is measured carefully before being divided to ensure appropriate size and dimension of the pieces.
Fig 2-3 Prominent location and low morbidity make the anterior tibial plateau (with its Gerdy tubercle) ideal for bone harvesting as an outpatient procedure under intravenous (IV) sedation in the dental surgeon’s office.
(a) The exact location of the osteotomy is marked with a red circle.
(b) Anterior tibial plateau bone harvesting requires full surgical scrub, Betadine preparation, and appropriate surgical drapes to maintain a sterile field and avoid contamination of the wound.
(c) The surrounding anatomy is outlined before surgery begins. This procedure is discussed in detail in chapter 7.
(d) A carpule of standard lidocaine with 1:100,000 epinephrine is administered before the incision is made.
(e) An initial oblique incision of approximately 1.5 cm is made through the skin above the Gerdy tubercle.
(f) Tissues are incised by layers to the bone; an appropriate bur is then used to create a small opening in the cortical bone. Bone is then harvested with a no. 4 Molt curette (G. Hartzell & Son, Concord, CA) or a straight orthopedic curette.
(g) Additional bone marrow can be scooped from the tibia with back-angle curettes to allow deeper access when needed.
(h) The cavity created within the bone. A hemostatic agent is placed in the cavity prior to suturing in layers. Within 3 to 4 months, the cancellous bone will naturally regenerate.
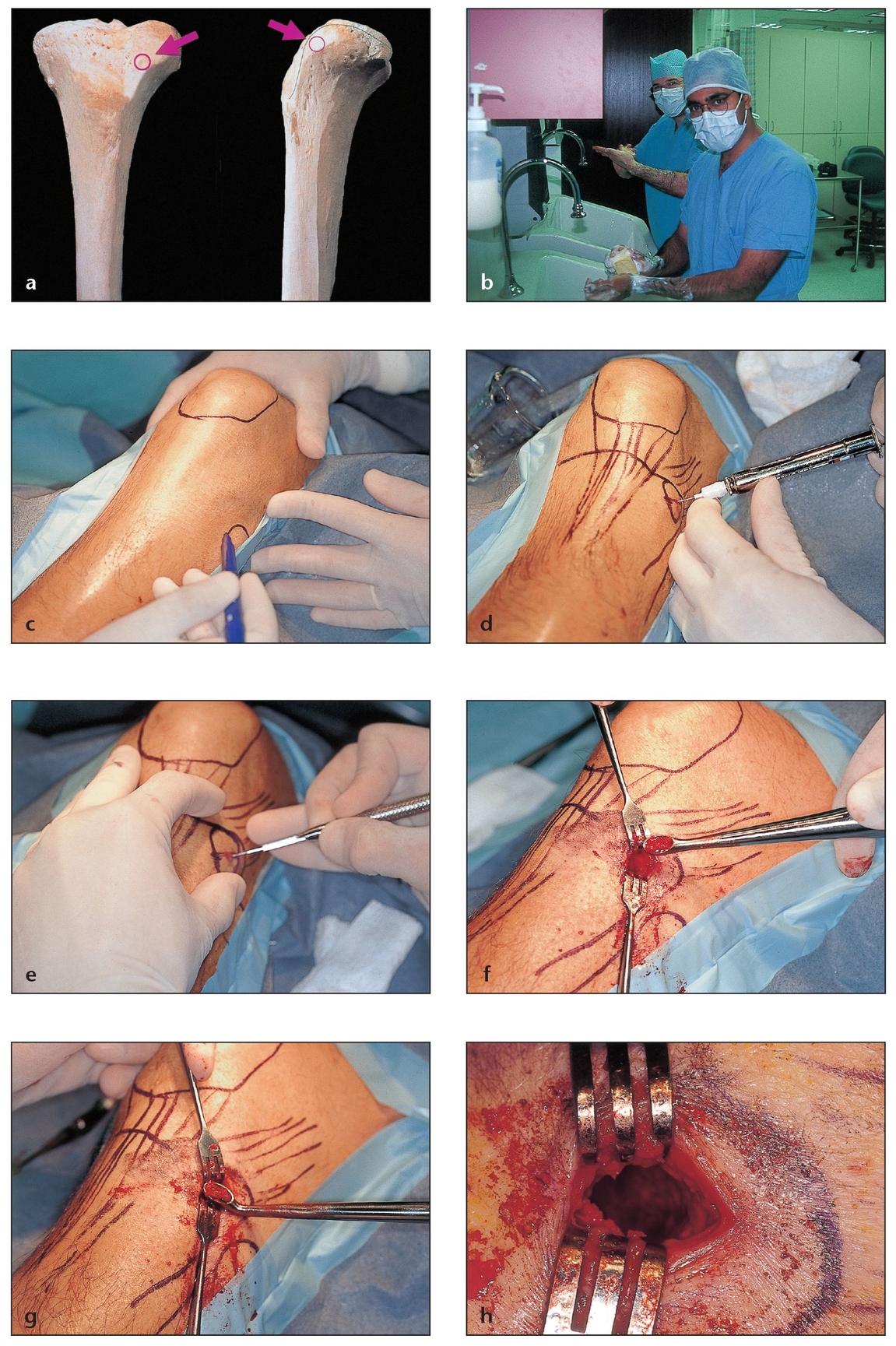
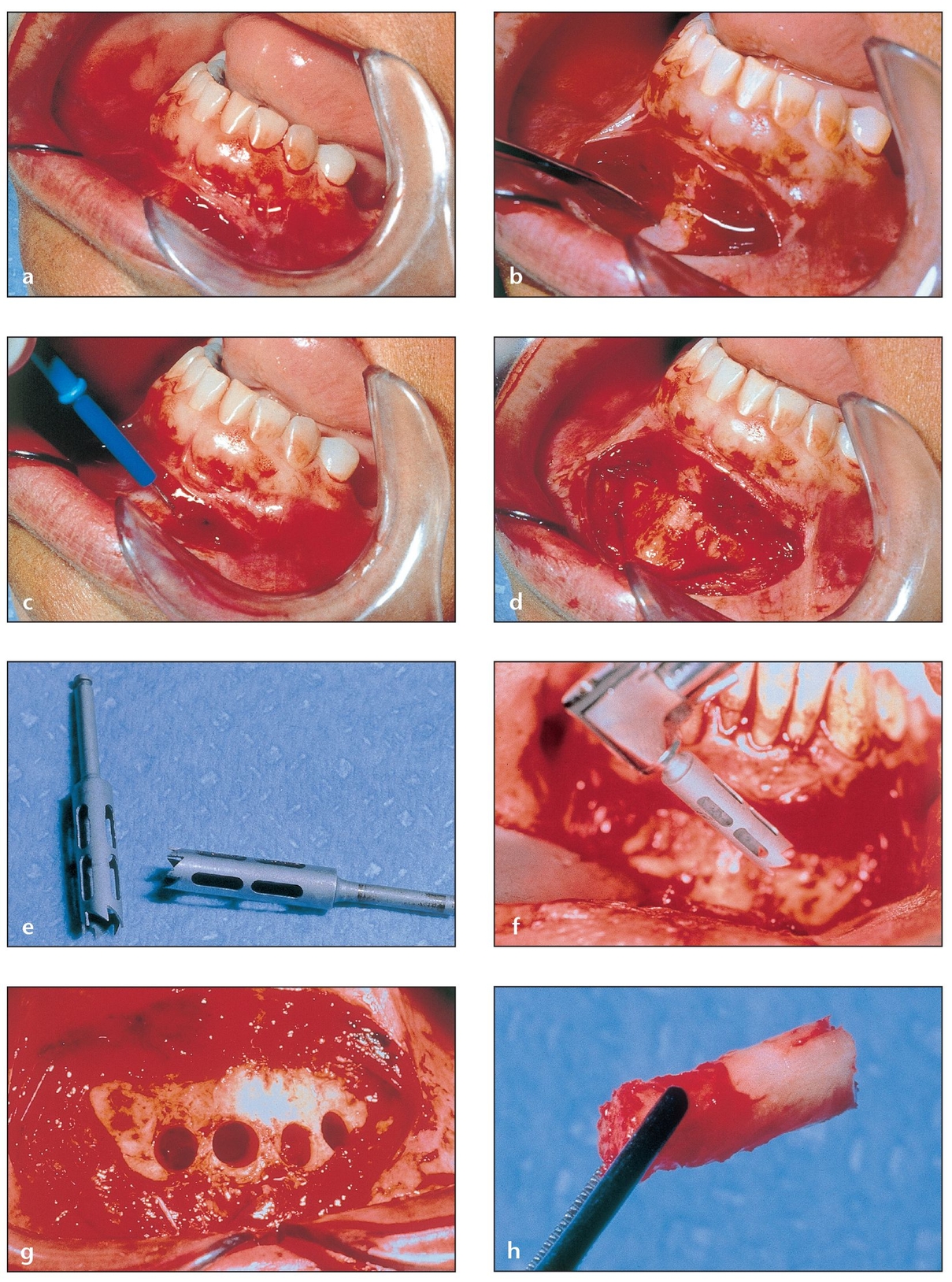
Fig 2-4 The anterior mandible may be used to harvest up to 5 mL of corticocancellous bone.
(a) A vestibular incision is made 3 to 5 mm apical to the mucogingival junction.
(b) The mentalis muscle is dissected to expose the facial aspect of the anterior mandible.
(c) The electrocautery tip is used to control bleeding, which can impair visualization of the bony wall.
(d) The mental area is exposed and ready for harvesting. This procedure is discussed in detail in chapter 6.
(e) Trephine burs of 4-mm diameter are ideal for harvesting chinbone cores that can later be crushed and used in particles. Microsaws or cylindrical burs should be used to obtain bone blocks.
(f) Trephine burs can be used effectively in either an implant drill or a straight surgical handpiece.
(g) The bone cores, generally 8 to 10 mm long, can be harvested separately with a remaining septum in between (as shown) or as a whole for larger openings.
(h) One of the harvested bone cores. The cortical portion on the right side is relatively avascular and acellular when compared to the cancellous portion on the left.
Fig 2-5 Bone shavings from adjacent areas of the surgical site or from the buccal shelf or ascending ramus can provide a more modest volume of autogenous bone.
(a) Bone-shaving devices can be used to harvest autogenous bone.
(b) The curved blade shaves bone when “raked” with appropriate pressure over the bony surface, producing very thin, curled bone strips, which are collected in a chamber that can hold up to 2 mL of bone shavings.
(c) A “pencil grip” with an angulation of 25 to 30 degrees from the bone surface is recommended during the pull stroke.
(d) The bone in the chamber is easily retrieved when the blade is retracted. The blade can then be placed back in position for additional harvesting in the same patient. The device is disposable.
(e) The osseous coagulum is placed in a receptacle in which additional allogeneic or alloplastic graft materials can be added to increase the total volume. Platelet-rich plasma (PRP) can be added to increase healing capacity.
(f) A curled strip of bone shaving seen under a scanning electron microscope reveals how multiple shavings can provide a greater exposed volume for release of cells and proteins in the recipient site than they supplied at the donor site.
(g) A nondisposable bone-shaving device can also be used with a pull stroke over the bone surface.
(h) The blade can be removed to access the bone collected in the chamber.
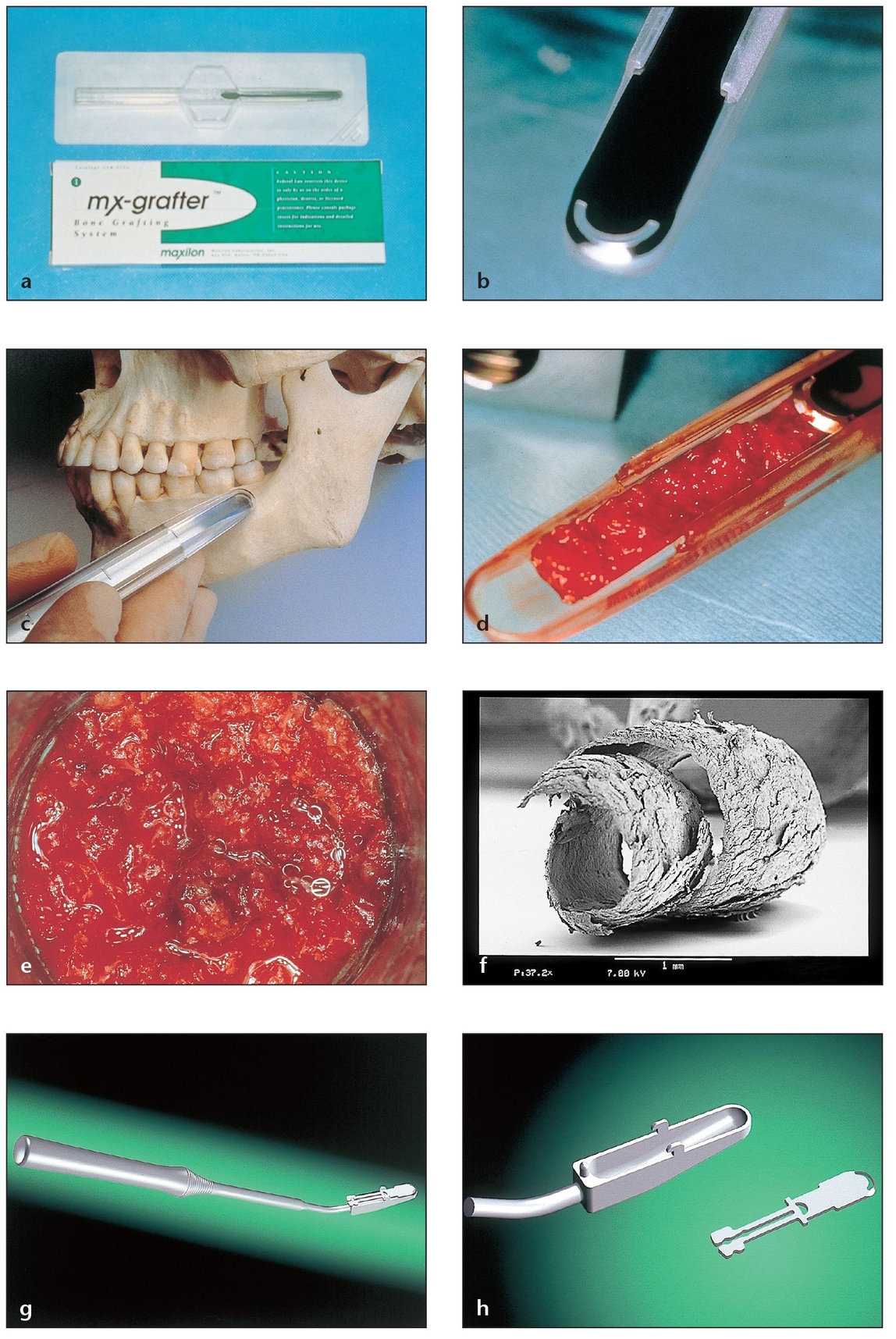
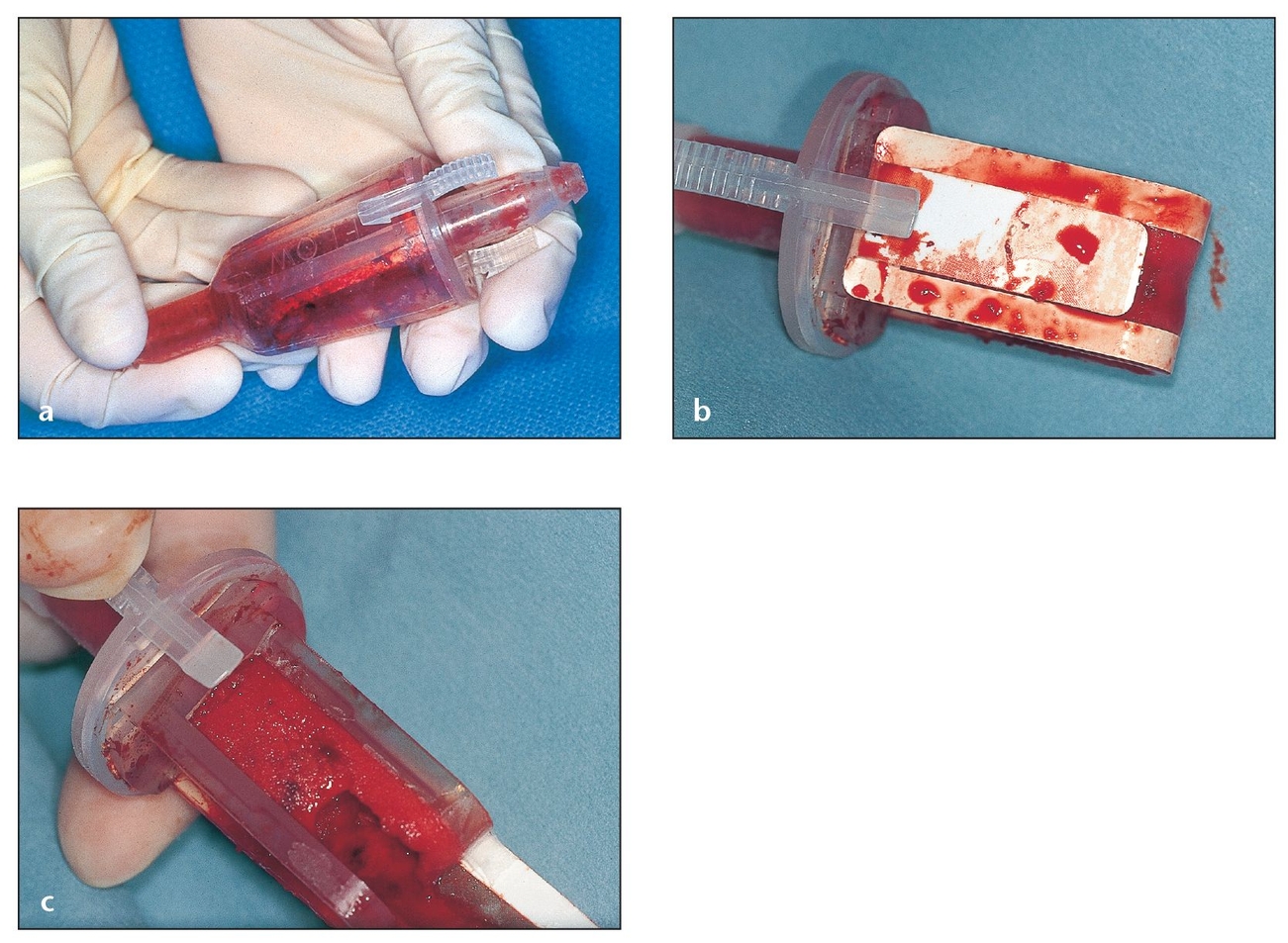
Fig 2-6 Autogenous bone collected with a suction trap while drilling osteotomies can be used to fill small defects.
(a) A commercially available, completely disposable suction trap. There are also many suction traps that can be sterilized for reuse and feature a disposable collecting screen.
(b) Bone can be harvested from a suction trap cup. The amount of bone collected in this device depends on many variables (eg, particle size obtained according to the bur used, amount of irrigation, amount of saliva, drilling speed). The viable bone cells are generally suctioned out; the autogenous bone will contain bacterial contamination.
(c) The side of this disposable trap can be opened by pulling on a tab. Inside is an osseous coagulum that can be augmented with other graft materials for small grafting procedures.
Allografts
Bone allografts are obtained from cadavers (Fig 2-7) or from patients’ living relatives or nonrelatives. Those obtained from cadavers are available through tissue banks that are accredited by the American Association of Tissue Banks, which process and store the allografts under complete sterility (Fig 2-8). The advantages of allografts include ready availability, elimination of the need for a patient donor site, reduced anesthesia and surgical time, decreased blood loss, and fewer complications.3 Disadvantages are primarily associated with the antigenicity of tissues harvested from another individual; transplanted bone may induce a host immune response. Cadaveric bone also may be rejected, as occurs with other transplanted tissues or organs.2, 3, 13
The most commonly used forms of allografts are frozen, freeze-dried (lyophilized), demineralized freeze-dried, and irradiated. Fresh allografts are the most antigenic; freezing or freeze-drying the bone significantly reduces the antigenicity.6 Because allografts are not osteogenic, bone formation takes longer and results in less volume than can be achieved with autogenous grafts.3 Concerns have been raised regarding the possible transmission of HIV through a bone allograft; however, when proper precautions and adequate laboratory studies are employed, the risk of using or receiving an allograft from an unrecognized early HIV–infected donor is approximately 1:1,600,000.14
FDBA can be used in either a mineralized or a demineralized (DFDBA) form. Demineralization removes the mineral phase of the graft material and purportedly exposes the underlying bone collagen and possibly some growth factors, particularly bone morphogenetic proteins (BMPs), which may increase its osteoinductive capabilities. 2, 15, 16 FDBA may form bone by osteoinduction and osteoconduction.3 Because it is mineralized, it hardens faster than DFDBA. Clinical experience has shown that grafting of the sinuses with DFDBA alone results in the presence of dense connective tissue after 6 months, whereas grafting with FDBA results in the presence of new bone formation.17 Bone is essential when treating defects in preparation for implant placement. The clinical and histologic findings of one study demonstrated that sites grafted with FDBA and complemented with an e-PTFE barrier can yield predictable results when augmenting alveolar ridges prior to the placement of implants.18
A memorial stone at the University of Miami honors organ and tissue donors. Harvesting, processing, and distribution of donated tissues are extensively regulated, limiting tissue availability.
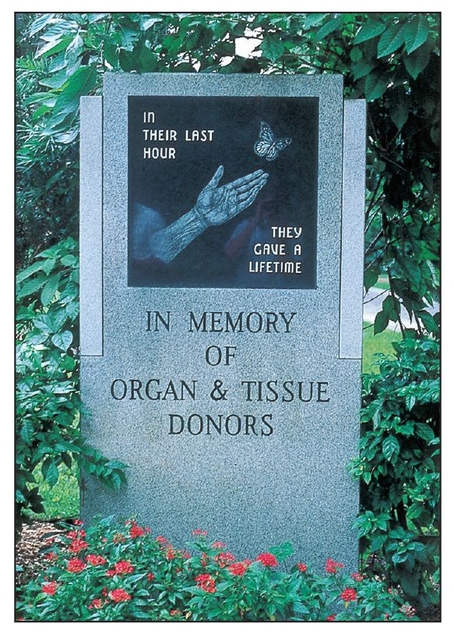
MTF (Dentsply Friadent CeraMed, Lakewood, CO) is an allogeneic freeze-dried bone that is available in both mineralized and demineralized forms. FDBA is more effective than DFDBA in the following situations:
- Repair and restoration of fenestrations
- Minor ridge augmentation
- Fresh extraction sites (used as a fill)
- Sinus lift cases (used as a graft)
- Repair of dehiscences and failing implants
This particulate material is available in a variety of sizes, which should be selected according to the intended application. Similar graft results have been shown from the use of particles ranging from 200 to 1,000 µm for various cases as needed. Indications for DFDBA are limited to periodontal defects.
Puros (Zimmer Dental, Carlsbad, CA) is an allogeneic graft material that has undergone a well-tested processing method to reduce antigenicity and to minimize any risk of viral cross-contamination from donor material.19 This type of allograft, which is solvent-preserved (as opposed to freeze-drying to extract the water component), has been shown to osseointegrate as effectively as cryopreserved material and to be equally biotolerable.20 Animal and human studies of this material have shown good bone formation and repair results.21, 22, 23, 24 Moreover, because the water component is removed by solvents rather than by freeze-drying, which can potentially alter the mineral as a result of the volume expansion that occurs during the transition from the liquid to the solid phase, the mineral matrix is purported to remain more intact.20 This material also has both the mineral and collagen phases of allogeneic tissues (Fig 2-9).
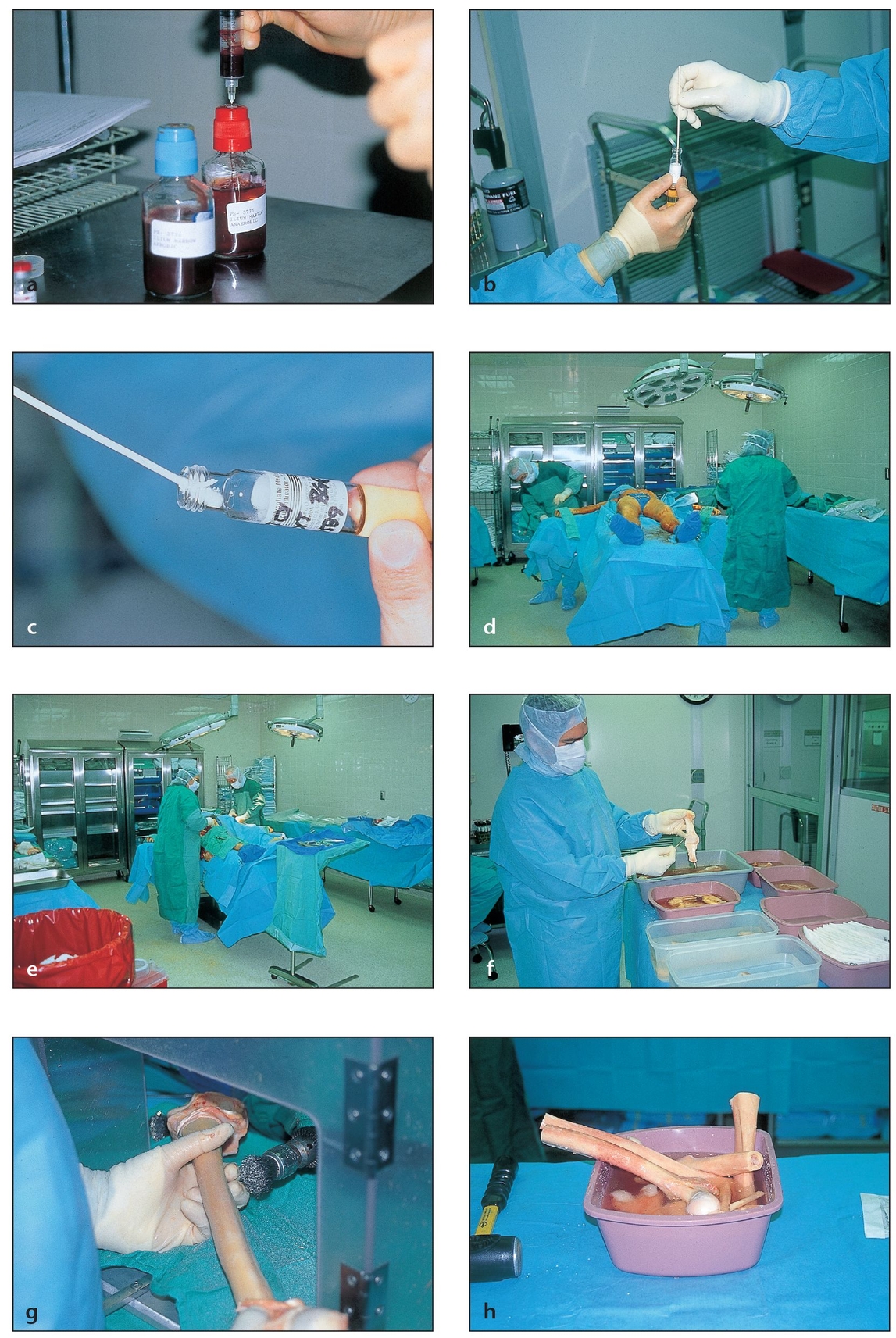
Fig 2-8 Bone allografts obtained from cadavers undergo strict screening and processing by tissue banks before they are made available to surgeons.
(a) Strict donor screening begins with blood tests.
(b) Culture studies are performed on tissue samples from the medullary canal, the specific tissues being donated, and the entire donor.
(c) Additional cultures are taken throughout the various steps of tissue processing. Material that is ready for distribution may have undergone as many as 200 cultures.
(d) High-quality tissue banks follow strict procedures for preparing and procuring specimens, beginning with a full surgical preparation within 24 hours of the donor’s death.
(e) The donor is prepared in completely sterile and aseptic conditions. A detailed autopsy is conducted to ensure that there are no underlying medical conditions that could contraindicate the use of the donor’s tissues.
(f) The different tissues are classified and placed in trays.
(g) The soft tissue is stripped from the bone surface, both by hand and mechanically.
(h) Large pieces of bone are cleaned and placed in separate containers.
(i) Osseous tissues are cut into different configurations according to the request of various surgical specialties (eg, orthopedics, oral surgery).
(j) Bones are cut into standard sizes and shapes or, when possible, based on a surgeon’s particular needs.
(k) The grafts are then ready for the removal of lipids, cells, and moisture.
(l) Tissues are soaked in sterile solutions to remove unwanted compounds.
(m) Once the lipids and cells are removed, the pieces are crushed into powders.
(n) The bone powders are passed through sifters to obtain particles of various sizes.
(o) The powders are freeze-dried in liquid nitrogen tanks or with chemical solvents to eliminate all remaining moisture.
(p) The freeze-dried bone is packaged in airtight containers and numbered for tracking before distribution. Demineralized freeze-dried bone is obtained by placing freeze-dried bone in a strong hydrochloride acid bath to remove its mineral matrix.
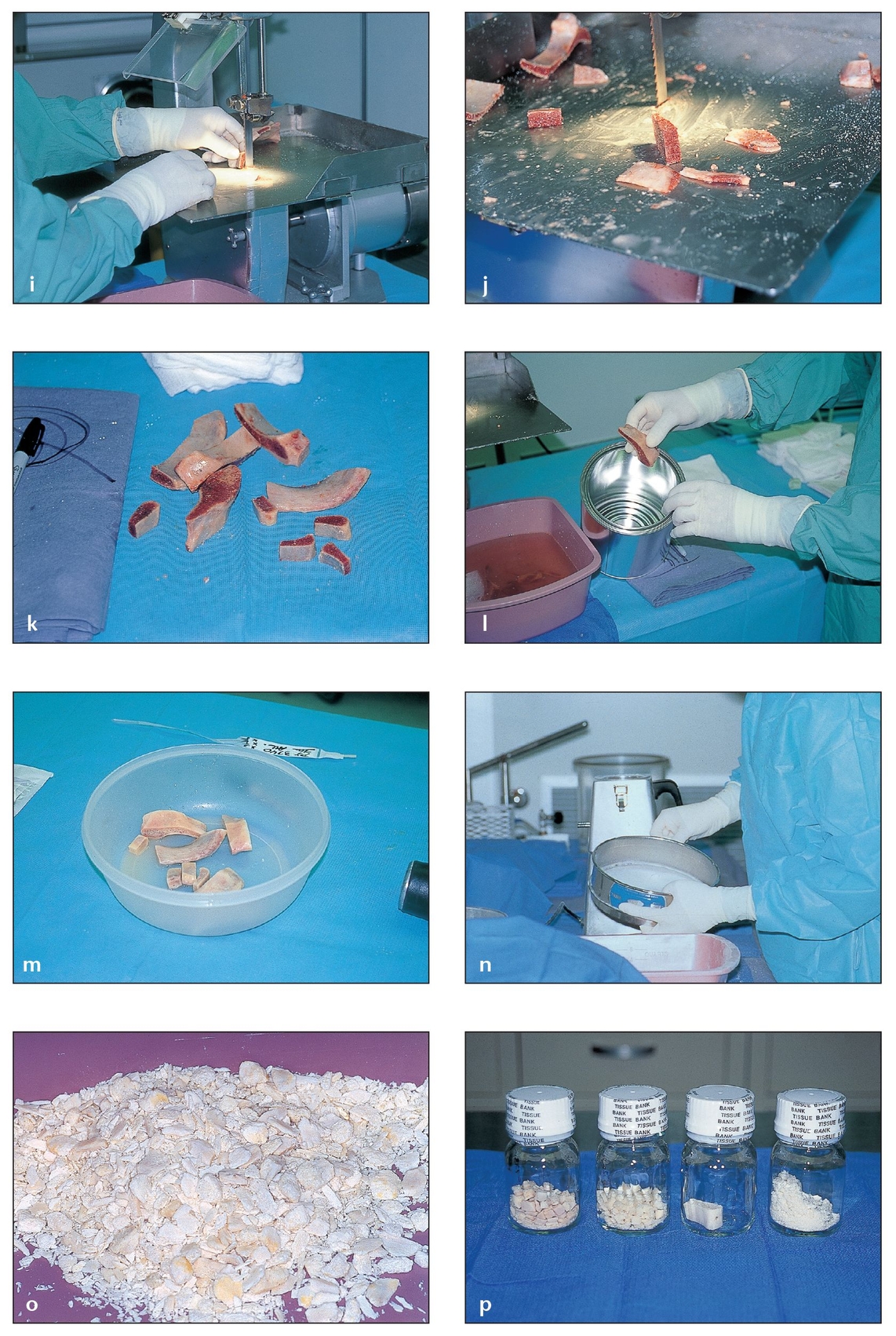
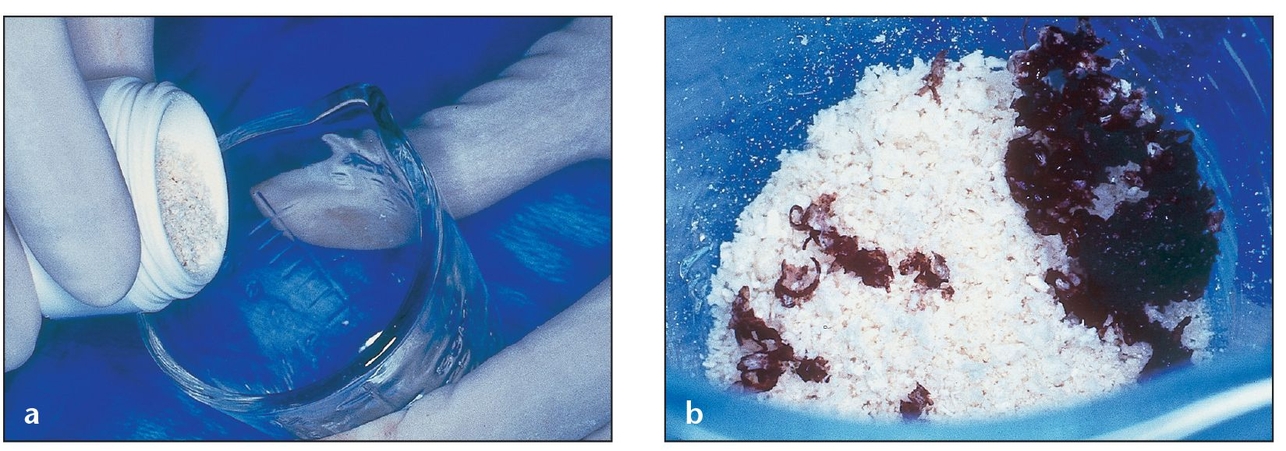
(a) A vial of Puros graft material is placed into a sterile container in a surgical field.
(b) The Puros graft material is rehydrated with nonactivated PRP to enhance healing. Blood from the patient that has been aspirated in the area of the surgical site can also be used for rehydration; alternatively, 0.9% saline solution may be used. Bone shavings from the patient have been added to the graft to provide living bone cells. Composite graft material should contain a minimum of 20% autogenous bone for best results.
The use of DFDBA as a graft material has been questioned because of some reports that it is unpredictable in regenerating new bone. In one study in humans, for instance, the DFDBA particles were found to be surrounded by uninflamed connective tissue.25 A later study showed positive results with the use of DFDBA and a cell-occlusive membrane. Incorporation of the DFDBA particles was observed in new bone that contained lacunae with osteocytes. 25 The results of this study might have been improved by the use of FDBA instead of DFDBA. The benefits seen in this study could also have been from the barrier membrane rather than the graft material, as it was DFDBA and not FDBA.
It is generally believed that BMPs and other noncollagenous proteins in the exposed matrix are responsible for the osteoinductivity of DFDBA. This osteoinductivity, however, depends on the quality and quantity of the bone matrix in the graft material.26 Studies have shown that the osteoinductive activity of DFDBA may vary considerably among bone banks as well as among different samples from the same bone bank.27 There are no widely accepted tests or guarantees to ensure that DFDBA material meets any minimum standards for osteoinductive properties. As a result, this graft material has fallen out of favor with many surgeons. In vitro and in vivo assays have been used to a limited extent to assess the osteoconductivity of DFDBA.26
DFDBA has been combined with other materials that have the potential to enhance bone growth. For example, the use of tetracycline with a DFDBA allograft has been studied. No significant benefit was shown to be derived from reconstituting the DFDBA particles in tetracycline hydrochloride during grafting of osseous defects. 28 Osteogenin, a bone-inductive protein isolated from human long bones, has been combined with DFDBA and studied in the regeneration of intrabony periodontal defects. While regeneration of new attachment apparatus and component tissues was significantly enhanced with this combination, new bone regeneration was not.29
A study using athymic rats has shown that two commercially prepared and available DFDBA preparations with gel carriers—Osteofil (Regeneration Technologies, Alachula, FL) and Grafton (Osteotech, Eatontown, NJ)—yielded similar results in bone formation via osteoconductivity at 28 days after implantation. However, significantly more bone was produced by Grafton than by Osteofil, suggesting that graft processing methods could represent a greater source of variability than do differences among donors.30
Irradiated cancellous bone (Rocky Mountain Tissue Bank, Denver, CO) has also been used as a substitute graft material for autogenous bone.31, 32 This bone allograft is trabecular bone obtained from the spinal column and treated with between 2.5 and 3.8 Megarads of radiation. Some authors have reported that among all available allografts, irradiated bone is most similar to autogenous bone in terms of demonstrating rapid replacement and consistent establishment of a reasonable ratio of new bone with less expense and morbidity than that associated with autogenous material.31, 32 However, because of a lack of published scientific documentation, use of this material is not recommended.
Alloplasts, Xenografts, and Tissue-Engineered Materials
The most commonly used bone substitutes are ceramic materials, including deorganified bovine bone, synthetic calcium phosphate ceramics (eg, hydroxyapatite, TCP), and calcium carbonate (eg, coralline). The mechanism of action in these ceramics is strictly osteoconduction,3, 33 with new bone formation taking place along their surfaces.13, 34 These materials are used to reconstruct bony defects and to augment resorbed alveolar ridges by providing a scaffold for enhanced bone tissue repair and growth. They can also improve probing de/>
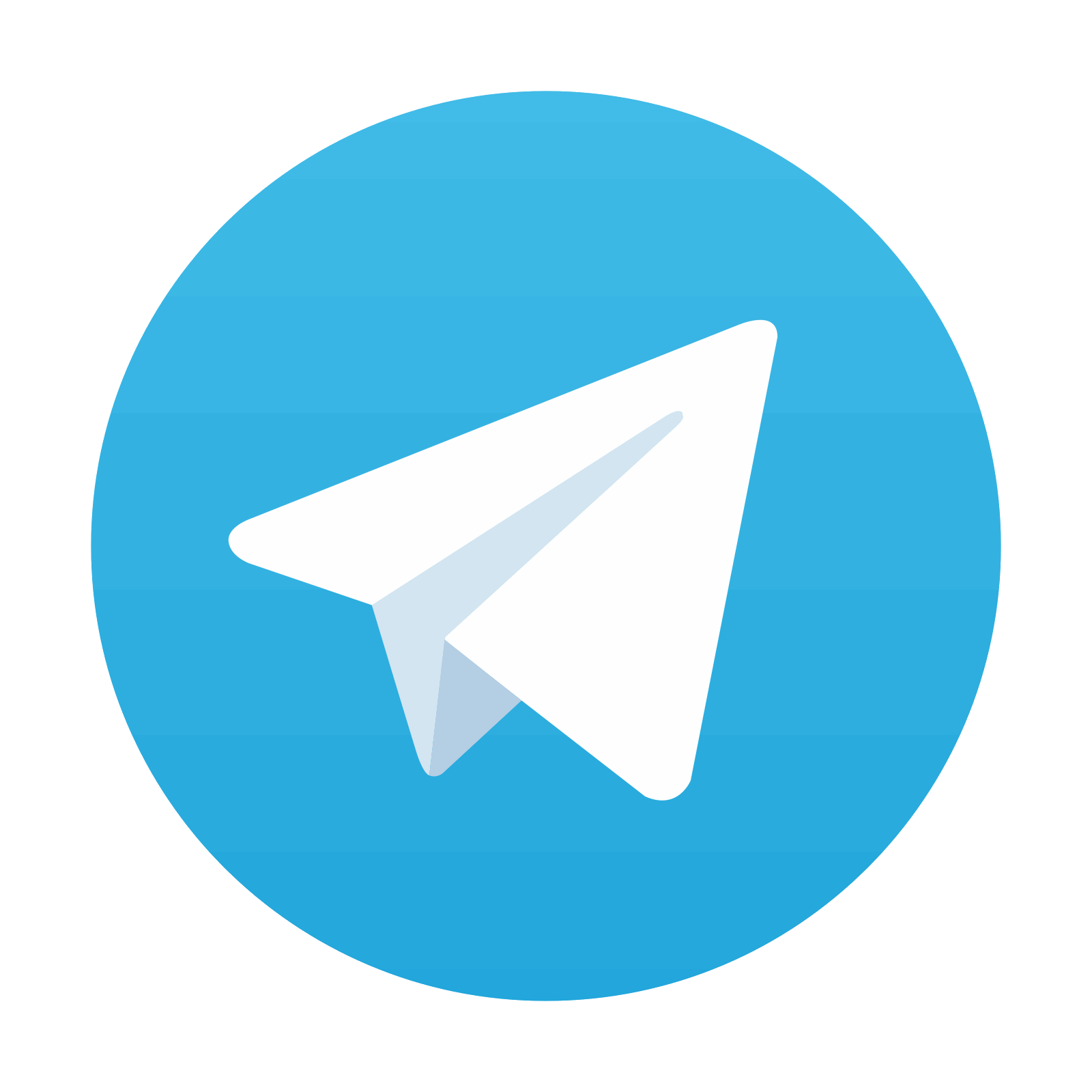
Stay updated, free dental videos. Join our Telegram channel

VIDEdental - Online dental courses
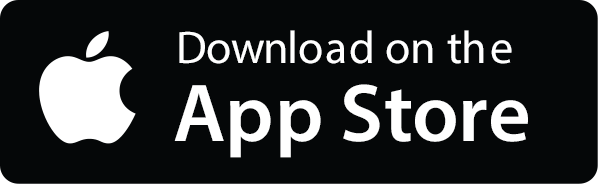
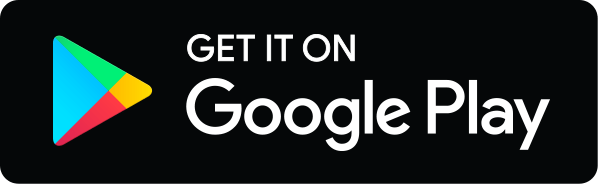