Functional Neuroanatomy and Physiology of the Masticatory System
”YOU CANNOT SUCCESSFULLY TREAT DYSFUNCTION UNLESS YOU UNDERSTAND FUNCTION.”
—JPO
THE FUNCTION OF THE MASTICATORY system is complex. Discriminatory contraction of the various head and neck muscles is necessary to move the mandible precisely and allow effective functioning. A highly refined neurologic control system regulates and coordinates the activities of the entire masticatory system. It consists primarily of nerves and muscles; hence the term neuromuscular system. A basic grasp of the anatomy and function of the neuromuscular system is essential to an understanding of the influence that tooth contacts as well as other conditions have on mandibular movement.
This chapter is divided into three sections. The first reviews in detail the basic neuroanatomy and function of the neuromuscular system. The second describes the basic physiologic activities of mastication, swallowing, and speech. The third reviews important concepts and mechanisms that are necessary to understand orofacial pain. A grasp of the concepts outlined in these three sections will greatly enhance the clinician’s ability to understand a patient’s complaint and provide effective therapy.
Anatomy and Function of the Neuromuscular System
For purposes of discussion, the neuromuscular system is divided into its two major components: the neurologic structures and the muscles. The anatomy and function of each of these components is reviewed separately, although in many instances it is difficult to distinguish them. With an understanding of these components, basic neuromuscular function can be reviewed.
Neurologic Structures
The neuron
The basic structural unit of the nervous system is the neuron. It is composed of a mass of protoplasm termed the nerve cell body and protoplasmic processes from the nerve cell body called dendrites and axons. The nerve cell bodies located in the spinal cord are found in the gray substance of the central nervous system (CNS). Cell bodies found outside the CNS are grouped together in ganglia. The axon (from the Greek word axon, meaning “axle” or “axis”) is the central core that forms the essential conducting part of a neuron and is an extension of cytoplasm from a nerve cell. Many neurons are grouped together to form a nerve fiber. These neurons are capable of transferring electrical and chemical impulses along their axes, thus enabling information to pass both in and out of the CNS. Depending on their location and function, neurons are designated by different terms. An afferent neuron conducts the nervous impulse toward the CNS, whereas an efferent neuron conducts it peripherally. Internuncial neurons, or interneurons, lie wholly within the CNS. The first sensory neuron is called the primary or first-order neuron. Second– and third-order sensory neurons are interneurons. Motor or efferent neurons convey nervous impulses to produce muscular or secretory effects.
Nerve impulses are transmitted from one neuron to another only at a synaptic junction, or synapse, where the processes of two neurons are in close proximity. All afferent synapses are located within the gray substance of the CNS; normally, therefore, there are no anatomic peripheral connections between sensory fibers. All connections are within the CNS, and the peripheral transmission of a sensory impulse from one fiber to another is abnormal.
The sensory receptors
Sensory receptors are neurologic structures or organs located in all body tissues that provide information to the CNS by way of the afferent neurons regarding the status of these tissues. As in other areas of the body, various types of sensory receptors are located throughout the tissues that make up the masticatory system. Specialized sensory receptors provide specific information to the afferent neurons and thus back to the CNS.
Sensory receptors found in the peripheral tissues such as the skin and oral mucosa are called exteroceptors. These receptors provide information from the exterior tissues of the body informing the CNS of the conditions in the environment. There are specialized exteroceptors to depict heat, cold, light touch, and pressure. There are also receptors that are specific for discomfort and pain. These are called nociceptors. Nociceptors are located not only in the peripheral tissues, but also throughout the body.
Other receptors provide information regarding the position and movement of the mandible and associated oral structures. These are called proprioceptors and are primarily found in all the musculoskeletal structures. Receptors that carry information regarding the status of the internal organs are referred to as interoceptors. Interoceptors inform the CNS of the status of internal structures and processes such as blood flow, digestion, and breathing. Constant input received from all of these receptors allows the cortex and brainstem to coordinate the action of individual muscles or muscle groups so as to create an appropriate response.
Information from the tissues outside the CNS must be transferred into the CNS and onto the higher centers in the brainstem and cortex for interpretation and evaluation. Once this information is evaluated, appropriate action must be taken. The higher centers then send impulses down the spinal cord and back out to the periphery to an efferent organ for the desired action. The primary afferent neuron (first-order neuron) receives a stimulus from the sensory receptor. This impulse is carried by the primary afferent neuron into the CNS by way of the dorsal root to synapse in the dorsal horn of the spinal cord with a secondary (second-order) neuron (Figure 2-1). The cell bodies of all the primary afferent neurons are located in the dorsal root ganglia. The impulse is then carried by the second-order neuron across the spinal cord to the anterolateral spinothalamic pathway, which ascends to the higher centers. There may be multiple interneurons (third-order, fourth-order, etc.) involved with the transfer of this impulse to the thalamus and cortex. There are also interneurons located in the dorsal horn that may become involved with the impulse as it synapses with the second-order neuron. Some of these neurons may synapse directly with an efferent neuron that is directed back out of the CNS by way of the ventral root to stimulate an efferent organ, such as a muscle.
The brainstem and brain
Once nerve impulses have been passed to the second-order neurons, these neurons carry them to the higher centers for interpretation and evaluation. There are numerous centers in the brainstem and brain that help give meaning to these impulses. It should also be remembered that numerous interneurons may be involved in transmitting the impulses on to higher centers. In fact, attempting to follow an impulse through the brainstem on to the cortex is no simple task. In order to intelligently discuss muscle function and pain in this text, certain functional regions of the brainstem and brain must be described. The following description merely overviews a few of the important functional components of the CNS; other texts may be consulted for a more complete review.1,2
Figure 2-2 is a graphic depiction of the functional areas of the brainstem and brain reviewed in this section. An understanding of these areas and their functions is most helpful in appreciating orofacial pain. The important areas reviewed below are the spinal tract nucleus, the reticular formation, the thalamus, the hypothalamus, the limbic structures and the cortex. They are discussed in the order by which neural impulses pass on to the higher centers.
The spinal tract nucleus
Throughout the body, primary afferent neurons synapse with the second-order neurons in the dorsal horn of the spinal column. Afferent input from the face and oral structures, however, does not enter the spinal cord by way of spinal nerves. Instead, sensory input from the face and mouth is carried by way of the fifth cranial nerve, the trigeminal nerve. The cell bodies of the trigeminal afferent neurons are located in the large gasserian ganglion. Impulses carried by the trigeminal nerve enter directly into the brainstem in the region of the pons to synapse in the trigeminal spinal nucleus (Figure 2-2). This region of the brainstem is structurally very similar to the dorsal horn of the spinal cord. In fact, it may be considered an extension of the dorsal horn and is sometimes referred to as the medullary dorsal horn.
The trigeminal complex of the brainstem consists of (1) the main sensory trigeminal nucleus, which is rostrally located and receives periodontal and some pulpal afferents, and (2) the spinal tract of the trigeminal nucleus, which is more caudally located. The spinal tract is divided into (1) the subnucleus oralis, (2) the subnucleus interpolaris, and (3) the subnucleus caudalis, which corresponds to the medullary dorsal horn. Tooth pulp afferents go to all three subnuclei.3 The subnucleus caudalis has especially been implicated in trigeminal nociceptive mechanisms on the basis of electrophysiologic observations of nociceptive neurons.4,5 The subnucleus oralis appears to be a significant area of this trigeminal brainstem complex for oral pain mechanisms.5–7
Another component of the trigeminal brainstem complex is the motor nucleus of the fifth cranial nerve. This area of the complex is involved with interpretation of impulses that demand motor responses. Motor reflex activities of the face are initiated from this area in a similar manner to the spinal reflex activities in the rest of the body.8
The reticular formation
After the primary afferent neurons synapse in the spinal tract nucleus, the interneurons transmit the impulses up to the higher centers. The interneurons ascend by way of several tracts passing through an area of the brainstem called the reticular formation. Within the reticular formation are concentrations of cells or nuclei that represent “centers” for various functions. The reticular formation plays an extremely important role in monitoring impulses that enter the brainstem. It controls the overall activity of the brain by either enhancing the impulses to the brain or inhibiting the impulses. This portion of the brainstem has an extremely important influence on pain and other sensory input.
The thalamus
The thalamus is located in the very center of the brain, with the cerebrum surrounding it from the top and sides and the midbrain below (Figure 2-2). It is made up of numerous nuclei that function together to interrupt impulses. Almost all impulses from the lower regions of the brain as well as from the spinal cord are relayed through synapses in the thalamus before proceeding to the cerebral cortex. The thalamus acts as a relay station for most of the communication between the brainstem, cerebellum, and cerebrum. As impulses arise to the thalamus, the thalamus makes assessments and directs the impulses to appropriate regions in the higher centers for interpretation and response.
If one were to compare the human brain to a computer, the thalamus would represent the keyboard, which controls the functions and directs the signals. The thalamus drives the cortex to activity and enables the cortex to communicate with the other regions of the CNS. Without the thalamus, the cortex is useless.
The hypothalamus
The hypothalamus is a small structure in the middle of the base of the brain. Although it is small, its function is great. The hypothalamus is the major center of the brain for controlling internal body functions, such as body temperature, hunger, and thirst. Stimulation of the hypothalamus excites the sympathetic nervous system throughout the body, increasing the overall level of activity of many internal parts of the body, especially increasing heart rate and causing blood vessel constriction. One can clearly see that this small area of the brain has some powerful effects on overall function. As discussed later, an increased level of emotional stress can stimulate the hypothalamus to upregulate the sympathetic nervous system and greatly influence nociceptive impulses entering the brain. This simple statement should have extreme meaning to the clinician managing pain.
The limbic structures
The word limbic means “border.” The limbic system comprises the border structures of the cerebrum and the diencephalon. The limbic structures function to control our emotional and behavioral activities. Within the limbic structures are nuclei or centers responsible for specific behaviors such as anger, rage, and docility. The limbic structures also control emotions such as depression, anxiety, fear, and paranoia. There also appears to be a pain/pleasure center that on an instinctive level drives the individual toward behaviors that stimulate the pleasure side of the center and away from pain. These drives are not generally perceived on a conscious level but more as a basic instinct. The instinct, however, will bring certain behaviors to consciousness. For example, when an individual experiences chronic pain, his or her behavior will be oriented toward withdrawal from any stimulus that might increase the pain. Often the sufferer will withdraw from life and mood changes such as depression will appear. It is believed that portions of the limbic structures interact and develop associations with the cortex, thereby coordinating the conscious cerebral behavioral functions with the subconscious behavioral functions of the deeper limbic system.
Impulses from the limbic system leading into the hypothalamus can modify any one or all of the many internal bodily functions controlled by the hypothalamus. Impulses from the limbic system feeding into the midbrain and medulla can control such behavior as wakefulness, sleep, excitement, and attentiveness. With this basic understanding of limbic function one can quickly understand the impact it can have on overall function. The limbic system certainly plays an essential role in pain problems, as discussed in later chapters.
The cortex
The cerebral cortex, made up predominantly of gray matter, is the outer region of the cerebrum. It is the portion of the brain most frequently associated with the thinking process—even though it cannot provide thinking without the simultaneous action of deeper structures of the brain—and it is here that essentially all of our memories are stored. This is also the area most responsible for our ability to acquire our many muscle skills. We still do not know the basic physiologic mechanisms by which the cerebral cortex stores either memories or knowledge of muscle skills.
Through most of its extent, the cerebral cortex is about 6 mm thick, and it contains an estimated 50 billion to 80 billion nerve cell bodies in all. Perhaps a billion nerve fibers lead away from the cortex and a comparable number lead into it. These nerve fibers pass to other areas of the cortex, to and from deeper structures of the brain, and in some instances all the way to the spinal cord.
Different regions of the cerebral cortex have been identified to have different functions. There is a motor area, which is primarily involved with coordinating motor function. There is a sensory area, which receives somatosensory input for evaluation. There are also areas for special senses, such as the visual and auditory areas.
If we were to again compare the human brain with a computer, the cerebral cortex would represent the hard disc drive that stores all information of memory and motor function. Once again, it is important to remember that the thalamus (the keyboard) is the necessary unit that prompts the cortex to function.
The Muscles
The motor unit
The basic component of the neuromuscular system is the motor unit, which consists of a number of muscle fibers innervated by one motor neuron. Each neuron joins with the muscle fiber at a motor endplate. When the neuron is activated, the motor endplate is stimulated to release small amounts of acetylcholine, which initiates depolarization of the muscle fibers (Figure 2-3). Depolarization causes the muscle fibers to shorten or contract.
The number of muscle fibers innervated by one motor neuron varies greatly according to the function of the motor unit. The fewer the muscle fibers per motor neuron, the more precise the movement. A single motor neuron may innervate only two or three muscle fibers, as in the ciliary muscles (which precisely control the lens of the eye). Conversely, one motor neuron may innervate hundreds of muscle fibers as in any large muscle (e.g., the rectus femoris in the leg). There is a similar variation in the number of muscle fibers per motor neuron within the muscles of mastication. The inferior lateral pterygoid muscle has a relatively low muscle fiber–motor neuron ratio and therefore is capable of the fine adjustments in length needed to adapt to horizontal changes in the mandibular position. In contrast, the masseter has a greater number of motor fibers per motor neuron, which corresponds to its more gross functions of providing the force necessary during mastication.
The muscle
Hundreds to thousands of motor units, along with blood vessels and nerves, are bundled together by connective tissue and fascia to make up a muscle. The major muscles that control movement of the masticatory system were described in Chapter 1. To understand the effect these muscles have on each other and their bony attachments, the basic skeletal relationships of the head and neck must be observed. The skull is supported in position by the cervical spine. It is not, however, centrally located or balanced over the cervical spine. In fact, if a dry skull were placed in its correct position on the cervical spine, it would be overbalanced to the anterior and quickly fall forward. Any balance becomes even more remote when the position of the mandible hanging below the anterior portion of the skull is considered. It can easily be seen that a balance of the skeletal components of the head and neck does not exist. Muscles are needed to overcome this imbalance of weight and mass. If the head is to be maintained in an upright position so it will be possible to see forward, muscles that attach the posterior aspect of the skull to the cervical spine and shoulder region must contract. Some of the muscles that serve this function are the trapezius, sternocleidomastoid, splenius capitis, and longus capitis. It is possible, however, for these muscles to overcontract and direct the line of vision too far upward. To counteract this, an antagonistic group of muscles exists in the anterior region of the head: the masseter (joining the mandible to the skull), the suprahyoids (joining the mandible to the hyoid bone), and the infrahyoids (joining the hyoid bone to the sternum and clavicle). When these muscles contract, the head is lowered. Thus there is a balance of muscular forces that maintains the head in the desired position (Figure 2-4). These muscles, plus others, also maintain proper side-to-side positioning and rotation of the head.
Muscle function
The motor unit can carry out only one action: contraction or shortening. The entire muscle, however, has three potential functions: (1) When a large number of motor units in the muscle are stimulated, contraction or an overall shortening of the muscle occurs. This type of shortening under a constant load is called isotonic contraction, which occurs in the masseter when the mandible is elevated, forcing the teeth through a bolus of food. (2) When a proper number of motor units contract in opposition to a given force, the resultant function of the muscle is to hold or stabilize the jaw. This contraction without shortening is called isometric contraction, and it occurs in the masseter when an object is held between the teeth (e.g., a pipe or pencil). (3) A muscle also can function through controlled relaxation. When stimulation of the motor unit is discontinued, the fibers of the motor unit relax and return to their normal length. By control of this decrease in motor unit stimulation, a precise muscle lengthening can occur that allows smooth and deliberate movement. This type of controlled relaxation is observed in the masseter when the mouth opens to accept a new bolus of food during mastication.
Using these three functions, the muscles of the head and neck maintain a constant desirable head position. A balance exists between the muscles that function to raise the head and those that function to depress it. During even the slightest movement of the head, each muscle functions in harmony with others to carry out the desired movement. If the head is turned to the right, certain muscles must shorten (isotonic contraction), others must relax (controlled relaxation), and still others must stabilize or hold certain relationships (isometric contraction). A highly sophisticated control system is needed to coordinate this finely tuned muscle balance.
These three types of muscle activities are present during the routine function of the head and neck. There is, however, another type of muscle activity called eccentric contraction, which can occur during certain conditions. This type of contraction is often injurious to the muscle tissue. Eccentric contraction refers to the forced lengthening of a muscle at the same time that it is contracting. An example of eccentric contraction occurs with the tissue damage associated during an extension-flexion injury (whiplash injury). At the precise moment of a motor vehicle accident, the cervical muscles contract to support the head and resist movement. If, however, the impact is great, the sudden change in the inertia of the head causes it to move while the muscles contract trying to support it. The result is a sudden lengthening of the muscles while they are contracting. This type of sudden lengthening while muscles are contracting often results in injury and is discussed in later sections devoted to muscle pain.
Muscle sensory receptors
Like other musculoskeletal units, the masticatory system utilizes four major types of sensory receptors (proprioceptors) to monitor the status of its structures: (1) the muscle spindles, which are specialized receptor organs found in the muscle tissues; (2) the Golgi tendon organs, located in the tendons; (3) the pacinian corpuscles, located in tendons, joints, periosteum, fascia, and subcutaneous tissues; and (4) the nociceptors, found generally throughout all the tissues of the masticatory system.
Muscle spindles
Skeletal muscles consist of two types of muscle fibers. The first is extrafusal fiber, which is contractile and makes up the bulk of the muscle; the other is intrafusal fiber, which is only minutely contractile. A bundle of intrafusal muscle fibers bound by a connective tissue sheath is called a muscle spindle (Figure 2-5). The muscle spindles primarily monitor tension within the skeletal muscles. They are interspersed throughout the muscles and aligned parallel with the extrafusal fibers. Within each spindle, the nuclei of the intrafusal fibers are arranged in two distinct fashions: chainlike (nuclear-chain type) and clumped (nuclear-bag type).
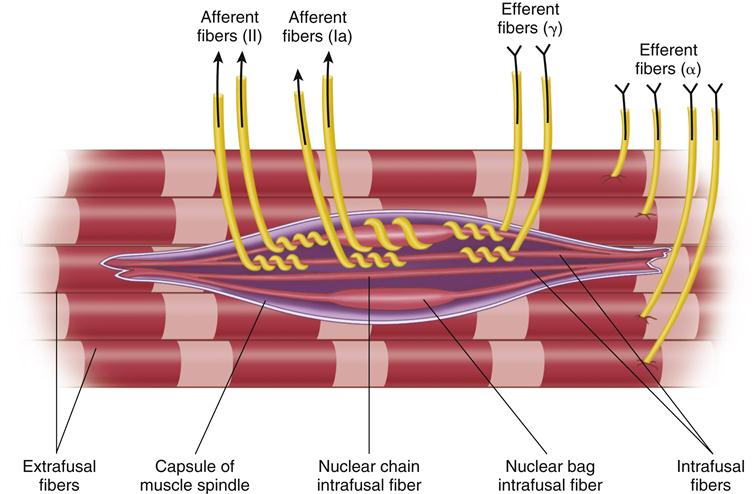
Two types of afferent nerves, classified according to their diameters, supply the intrafusal fibers. The larger fibers conduct impulses at a higher speed and have lower thresholds. Those that end in the central region of the intrafusal fibers are the larger group (Ia, A-alpha) (discussed later in this chapter) and are said to be the primary endings (so-called annulospiral endings). Those that end in the poles of the spindle (away from the central region) are the smaller group (II, A-beta) and are the secondary endings (so-called flower-spray endings).
The intrafusal fibers of the muscle spindles are aligned parallel to the extrafusal fibers of the muscles; therefore, as the muscle is stretched, so also are the intrafusal fibers. This stretch is monitored at the nuclear-chain and nuclear-bag regions. The annulospiral and flower-spray endings are activated by the stretch, and the afferent neurons carry these neural impulses to the CNS. The afferent neurons originating in the muscle spindles of the muscles of mastication have their cell bodies in the trigeminal mesencephalic nucleus.
The intrafusal fibers receive efferent innervation by way of fusimotor nerve fibers. These fibers are given the alphabetical classification of gamma fibers or gamma efferents to distinguish them from the alpha nerve fibers, which supply the extrafusal fibers. Like other efferent fibers, the gamma efferent fibers originate in the CNS; when stimulated, they cause contraction of the intrafusal fibers. When the intrafusal fibers contract, the nuclear-chain and nuclear-bag areas are stretched, which is registered as if the entire muscle were stretched, and afferent activity is initiated. Thus there are two manners in which the afferent fibers of the muscle spindles can be stimulated: generalized stretching of the entire muscle (extrafusal fibers) and contraction of the intrafusal fibers by way of the gamma efferents. The muscle spindles can register only the stretch; they cannot differentiate between these two activities. Therefore the activities are recorded as the same activity by the CNS.
The extrafusal muscle fibers receive innervation by way of the alpha efferent motor neurons. Most of these have their cell bodies in the trigeminal motor nucleus. Stimulation of these neurons therefore causes the group of extrafusal muscle fibers (the motor unit) to contract.
From a functional standpoint, the muscle spindle acts as a length-monitoring system. It constantly feeds information regarding the state of elongation or contraction of the muscle back to the CNS. When a muscle is suddenly stretched, both its extrafusal and its intrafusal fibers elongate. The stretch of the spindle causes firing of the group I and II afferent nerve endings leading back to the CNS. When the alpha efferent motor neurons are stimulated, the extrafusal fibers of the muscle contract and the spindle is shortened. This shortening brings about a decrease in the afferent output of the spindle. If there were no gamma efferent system, a total shutdown of the spindle activity would occur during muscle contraction. As stated earlier, stimulation of the gamma efferents causes the intrafusal fibers of the muscle spindle to contract. This can elicit afferent activity from the spindle even when the muscle is contracting. Gamma efferent drive can therefore assist in maintaining muscle contraction.
It is believed that the gamma efferent system acts as a mechanism to sensitize the muscle spindles. Thus this fusimotor system acts as a biasing mechanism that alters the firing of the muscle spindle. It should be noted that the gamma efferent mechanism is not as well investigated in the masticatory system as in other spinal cord systems. Although it appears to be active in most of the masticatory muscles, some apparently have no gamma efferents. The importance of the gamma efferent system is further emphasized in the discussion of muscle reflexes.
Golgi tendon organs
The Golgi tendon organs are located in the muscle tendon between the muscle fibers and their attachment to the bone. At one time they were thought to have a higher sensory threshold than the muscle spindles and therefore functioned solely to protect the muscle from excessive or damaging tension. It now appears that they are more sensitive and active in reflex regulation during normal function. They primarily monitor tension, whereas the muscle spindles primarily monitor muscle length.
The Golgi tendon organs occur in series with the extrafusal muscle fibers and not in parallel, as with the muscle spindles. Each of these sensory organs consists of tendinous fibers surrounded by lymph spaces enclosed within a fibrous capsule. Afferent fibers enter near the middle of the organ and spread out over the extent of the fibers. Tension on the tendon stimulates the receptors in the Golgi tendon organ. Therefore contraction of the muscle also stimulates the organ. Likewise, an overall stretching of the muscle creates tension in the tendon and again stimulates the organ.
Pacinian corpuscles
The pacinian corpuscles are large oval organs made up of concentric lamellae of connective tissue. These organs are widely distributed and, because of their frequent location in the joint structures, are considered to serve principally for the perception of movement and firm pressure (not light touch).
At the center of each corpuscle is a core containing the termination of a nerve fiber. These corpuscles are found in the tendons, joints, periosteum, tendinous insertions, fascia, and subcutaneous tissue. Pressure applied to such tissues deforms the organ and stimulates the nerve fiber.
Nociceptors
Generally, nociceptors are sensory receptors that are stimulated by injury and transmit injury information (nociception) to the CNS by way of the afferent nerve fibers. Nociceptors are located throughout most of the tissues in the masticatory system. Several general types exist: some respond exclusively to noxious mechanical and thermal stimuli; others respond to a wide range of stimuli, from tactile sensations to noxious injury; still others are low-threshold receptors specific for light touch, pressure, or facial hair movement. The last type is sometimes called a mechanoreceptor.
The nociceptors (along with the proprioceptors) primarily function to monitor the condition, position, and movement of the tissues in the masticatory system. When conditions exist that are either potentially harmful or actually cause injury to the tissue, the nociceptors relay this information to the CNS as sensations of discomfort or pain. The sensation of pain is discussed later in this chapter.
Neuromuscular Function
Function of the sensory receptors
The dynamic balance of the head and neck muscles previously described is possible through feedback provided by the various sensory receptors. When a muscle is passively stretched, the spindles inform the CNS of this activity. Active muscle contraction is monitored by both the Golgi tendon organs and the muscle spindles. Movement of the joints and tendons stimulates the pacinian corpuscles. All of the sensory receptors are continuously providing input to the CNS. The brainstem and thalamus are in charge of constantly monitoring and regulating body activities. Information concerning normal body homeostasis is dealt with at this level and the cortex is not even brought into the regulatory process. If, however, incoming information has significant consequence to the person, the thalamus passes the information to the cortex for conscious evaluation and decision. The thalamus and brainstem therefore have a powerful influence on the individual’s function.
Reflex action
A reflex action is the response resulting from a stimulus that passes as an impulse along an afferent neuron to a posterior nerve root or its cranial equivalent, from which it is then transmitted to an efferent neuron leading back to the skeletal muscle. Although the information is sent to the higher centers, the response is independent of will and occurs normally with no cortical or brainstem influence. A reflex action may be monosynaptic or polysynaptic. A monosynaptic reflex occurs when the afferent fiber directly stimulates the efferent fiber in the CNS. A polysynaptic reflex is present when the afferent neuron stimulates one or more interneurons in the CNS, which in turn stimulate the efferent nerve fibers.
Two general reflex actions are important in the masticatory system: (1) the myotatic reflex and (2) the nociceptive reflex. These are not unique to the masticatory muscles but are found in other skeletal muscles as well.
Myotatic (stretch) reflex
The myotatic, or stretch, reflex is the only monosynaptic jaw reflex. When a skeletal muscle is quickly stretched, this protective reflex is elicited and brings about a contraction of the stretched muscle.
A well-known example of the myotatic reflex is the patellar or knee-jerk reflex. When the patellar tendon is struck with a tendon hammer just below the patella, the quadriceps muscles in the thigh is stretched. This stimulates stretch sensory receptors (most importantly muscle spindles) that trigger an afferent impulse in a sensory nerve fiber of the femoral nerve, leading to the lumbar region (L4) of the spinal cord. There, the sensory neuron synapses directly with a motor neuron, which conducts an efferent impulse to the quadriceps femoris muscle, triggering contraction. This contraction, coordinated with the relaxation of the antagonistic flexor hamstring muscle, causes the leg to kick.
The myotatic reflex can be demonstrated in the masticatory system by observing the masseter muscle as a sudden downward force is applied to the chin. This force can be applied with a small rubber hammer (Figure 2-6). As the muscle spindles within the masseter suddenly stretch, afferent nerve activity is generated from the spindles. These afferent impulses pass into the brainstem to the trigeminal motor nucleus by way of the trigeminal mesencephalic nucleus. These same afferent fibers synapse with the alpha efferent motor neurons, leading directly back to the extrafusal fibers of the masseter. Stimulation of the alpha efferent by the Ia afferent fibers causes the muscle to contract. Clinically, this reflex can be demonstrated by relaxing the jaw muscles, allowing the teeth to separate slightly. A sudden downward tap on the chin will cause the jaw to be reflexively elevated. The masseter then contracts, resulting in tooth contact.
The myotatic reflex occurs without a specific response from the cortex and is very important in determining the resting position of the jaw. If there were complete relaxation of all the muscles that support the jaw, the forces of gravity would act to lower the jaw and separate the articular surfaces of the TMJ. To prevent this dislocation, the elevator muscles (and other muscles) are maintained in a mild state of contraction called muscle tonus. This property of the elevator muscles counteracts the effect of gravity on the mandible and maintains the articular surfaces of the joint in constant contact. The myotatic reflex is a principal determinant of muscle tonus in the elevator muscles. As gravity pulls down on the mandible, the elevator muscles are passively stretched, which also creates stretching of the muscle spindles. This information is reflexively passed from the afferent neurons originating in the spindles to the alpha motor neurons that lead back to the extrafusal fibers of the elevator muscles. Thus passive stretching causes a reactive contraction that relieves the stretch on the muscle spindle. Muscle tonus can also be influenced by afferent input from other sensory receptors, such as those from the skin or the oral mucosa.
The myotatic reflex and resulting muscle tonus can also be influenced by the higher centers via the fusimotor system. The cortex and brainstem can bring increased gamma efferent activity to the intrafusal fibers of the spindle. As this activity increases, the intrafusal fibers contract, causing a partial stretching of the nuclear-bag and nuclear-chain areas of the spindles. This lessens the amount of stretch needed in the overall muscle before the spindle afferent activity is elicited. Therefore the higher centers can use the fusimotor system to alter the muscle spindles’ sensitivity to stretch. Increased gamma efferent activity increases the sensitivity of the myotatic (stretch) reflex, whereas decreased gamma efferent activity decreases the sensitivity of this reflex. The specific manner by which the higher centers influence gamma efferent activity is summarized later in this chapter.
When a muscle contracts, the muscle spindles are shortened, which shuts down the afferent activity of these spindles. If the electrical potential of the afferent nerve activity is monitored, a silent period (no electrical activity) will be noted during this contraction stage. Gamma efferent activity can influence the length of the silent period. High gamma efferent activity makes the intrafusal fibers contract, which reduces the time the spindle is shut down during a muscle contraction. Decreased gamma efferent activity lengthens this silent period.
Nociceptive (flexor) reflex
The nociceptive, or flexor, reflex is a polysynaptic reflex to noxious stimuli and is therefore considered to be protective. Examples in the large limbs include the withdrawal of a hand as it touches a hot object. In the masticatory system, this reflex becomes active when a hard object is suddenly encountered during mastication—for example when, in eating a cherry pie, you unexpectedly encounter a cherry pit (Figure 2-7). The suddenly increased chewing force placed on the tooth instantly overloads the periodontal structures, producing a noxious stimulus. The primary afferent nerve fibers carry this information to the trigeminal spinal tract nucleus, where they synapse with interneurons. These interneurons travel to the trigeminal motor nucleus. The motor response that occurs during this reflex is more complicated than the myotatic reflex in that the activity of several muscle groups must be coordinated to carry out the desired motor response.9,10 Not only must the elevator muscles be inhibited to prevent further jaw closure on the hard object but the jaw opening muscles must be activated to bring the teeth away from potential damage.11,12 As the afferent information from the sensory receptors reaches the interneurons, two distinct actions occur. Excitatory interneurons leading to the efferent neurons in the trigeminal motor nucleus of the jaw-opening muscles are stimulated. This action causes these muscles to contract. At the same time, the afferent fibers stimulate inhibitory interneurons, which causes the jaw-elevating muscles to relax. The overall result is that the jaw quickly drops and the teeth are pulled away from the object causing the noxious stimulus. This process, called antagonistic inhibition, occurs in many nociceptive reflex actions throughout the body.
The myotatic reflex protects the masticatory system from sudden stretching of a muscle and maintains the stability of the musculoskeletal system with muscle tonicity. The nociceptive reflex protects the teeth and supportive structures from potential damage due to sudden and unusually heavy functional forces. The Golgi tendon organs protect the muscle from overcontraction by sending inhibiting stimuli directly to the muscle they monitor. Numerous other types of reflex actions are found in the muscles of mastication. Many are very complex and controlled by higher centers of the CNS. Reflex actions play a major role in functioning3 (e.g., mastication, swallowing, gagging, coughing, speaking).
Reciprocal innervation
The control of antagonistic muscles is of vital importance in reflex activity. It is of equal importance to the everyday function of the body. As in other muscle systems, each muscle that supports the head and in part controls function has an antagonist that counteracts its activity. This is the basis of the muscle balance previously described. Certain groups of muscles primarily elevate the mandible; other groups primarily depress it. For the mandible to be elevated by the temporal, medial pterygoid, or masseter muscles, the suprahyoid muscles must relax and lengthen. Likewise for the mandible to be depressed, the suprahyoids must contract while the elevators relax and lengthen.
The neurologic controlling mechanism for these antagonistic groups is known as reciprocal innervation. This phenomenon enables smooth and exact control of mandibular movement to be achieved. For the skeletal relationship of the skull, mandible, and neck to be maintained, each of the antagonistic muscle groups must remain in a constant state of mild tonus. This overcomes the skeletal imbalances of gravity />
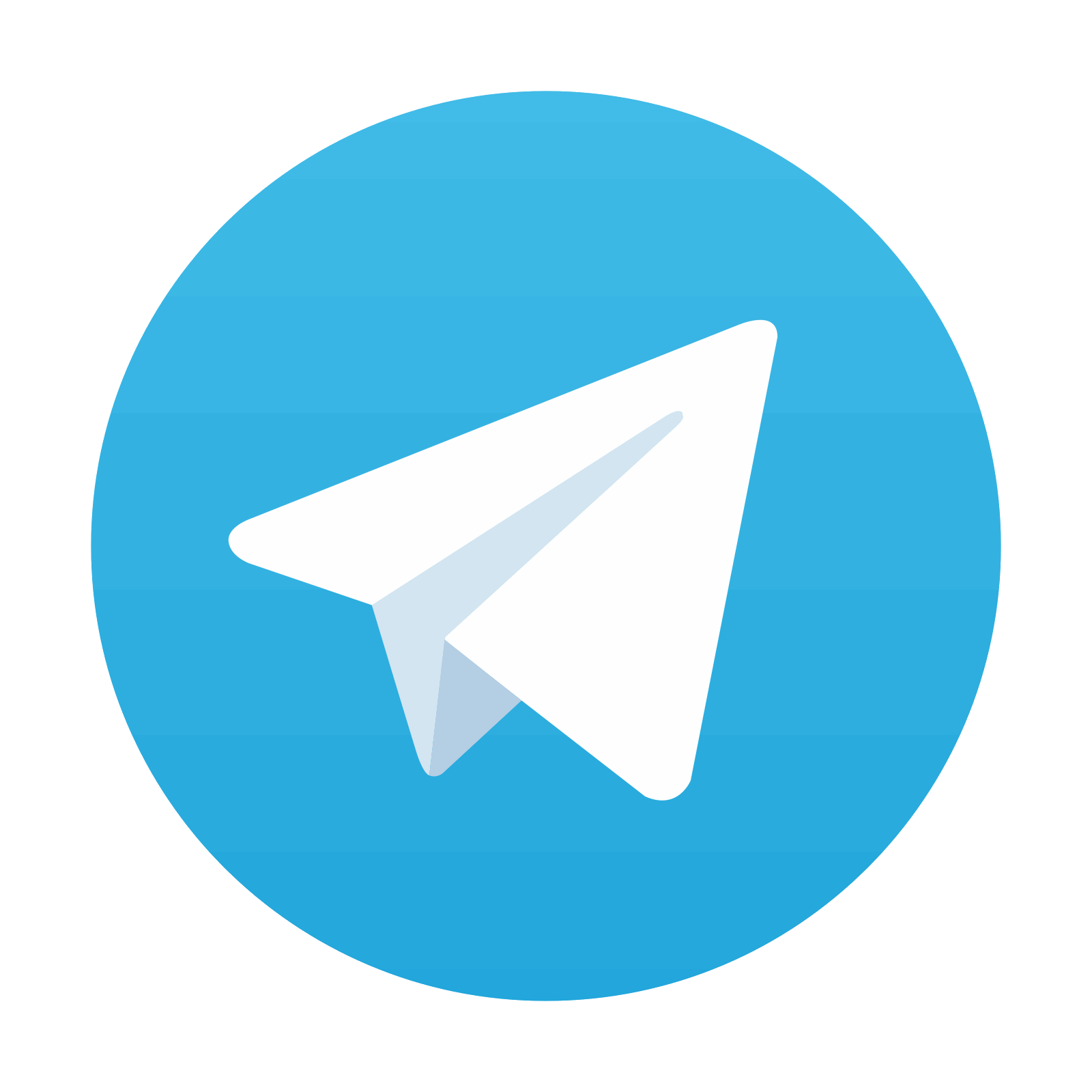
Stay updated, free dental videos. Join our Telegram channel

VIDEdental - Online dental courses
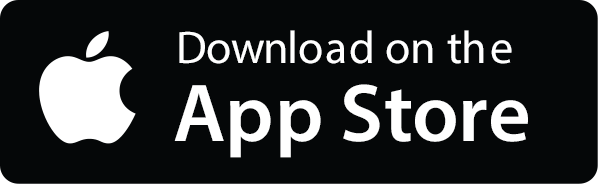
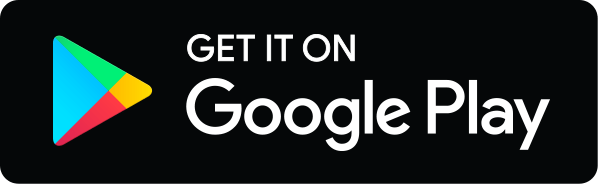