CHAPTER 1
Bone Physiology for Dental Implantology
The entire adult skeleton exists in a dynamic state, constantly broken down and re-formed by the coordinated actions of osteoclasts and osteoblasts (Fig 1-1). Bone is a living tissue that serves two primary functions: structural support and calcium metabolism.1 The bone matrix is composed of an extremely complex network of collagen protein fibers impregnated with mineral salts that include calcium phosphate (85%), calcium carbonate (10%), and small amounts of calcium fluoride and magnesium fluoride (5%).2 The minerals in bone are present primarily in the form of hydroxyapatites. Bone also contains small quantities of noncollagen proteins embedded in its mineral matrix, including the all-important family of bone morphogenetic proteins (BMPs). Coursing through the bone is a rich vascular network that provides perfusion to the viable cells, as well as the network of nerves (Fig 1-2). To maintain normal bone structure, sufficient amounts of proteins and minerals must be present.
Because of its unique architecture, bone is a mass-efficient structure in which maximal strength is achieved with absolutely minimal mass (Fig 1-3). In humans, bone mass reaches its maximum level approximately 10 years after the end of linear growth. This level normally remains fairly constant as bone is continually deposited and absorbed throughout the skeleton until sometime in the fourth decade of life, when bone mass begins to gradually decrease. Although the reasons are not clearly understood, this decline is a result of an ongoing net loss effect that begins to occur in the bone remodeling process. By age 80, both men and women typically have lost about half of their maximum bone mass value. Humans reach peak bone mineral density in their 30s, although it is lower in women than in men and in whites than in blacks. Women lose an estimated 35% of their cortical bone and 50% of cancellous bone as they age, while men lose only two-thirds of these amounts.3 Bone deemed unnecessary by the body (eg, atrophy and bone loss in paraplegic patients) is also lost during a shift in the absorption-deposition balance in bone remodeling; in addition, turnover may be a response to metabolic reactions.
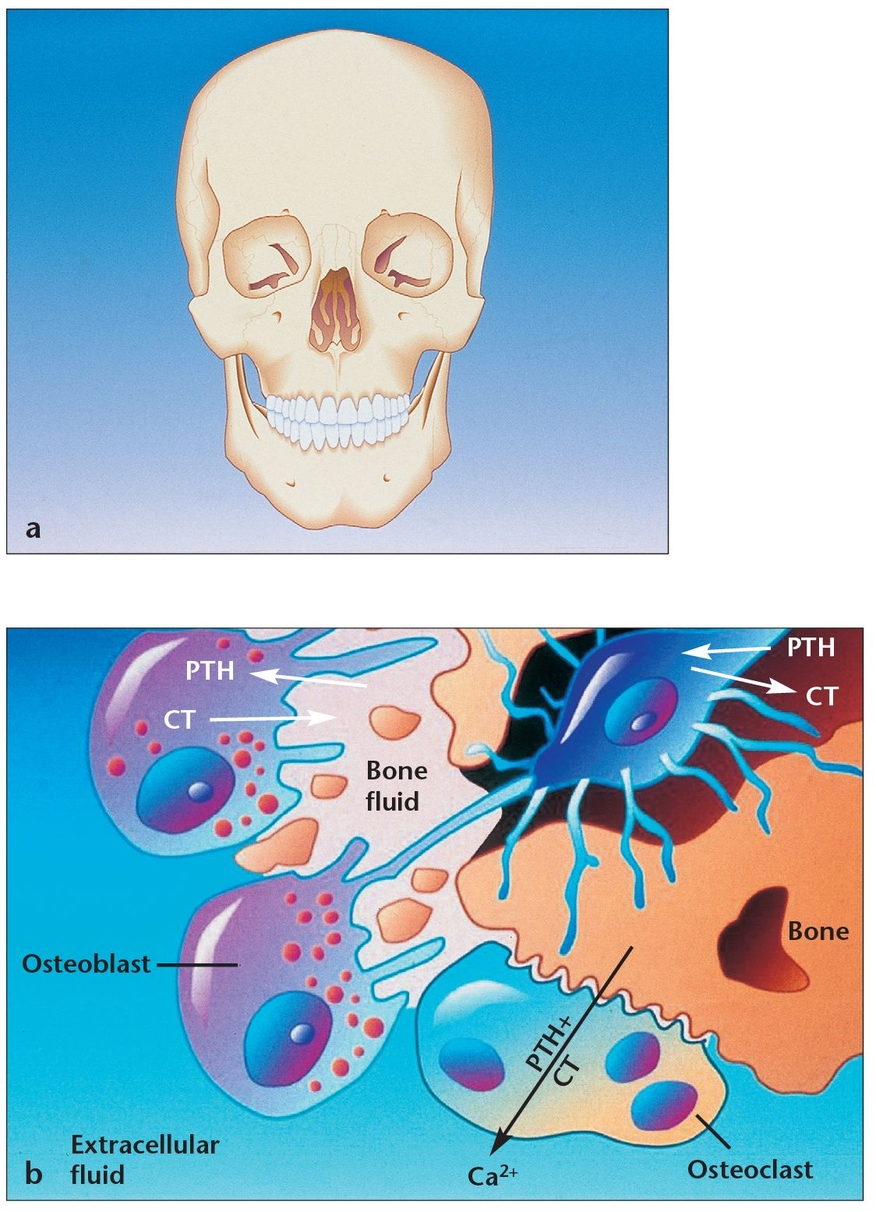
(a) A thorough knowledge of bone physiology is essential for understanding bone behavior during oral bone grafting, implant placement, osseointegration, and long-term bone maintenance.
(b) Osteoblasts and osteoclasts maintain metabolic equilibrium in all human bones, including the mandible and maxilla. When osteoblasts have successfully formed bone matrix and then become embedded in it, they transform into osteocytes. Osteocytes communicate with each other and with cells on the bone surface via dendritic processes encased in canaliculi. (PTH = parathyroid hormone; CT = calcitonin.)
The skull and jaws are unquestionably affected in all of these scenarios; therefore, it is important for the clinician working with dental implants to have a good understanding of bone structure and metabolism as well as knowledge of the process of osseointegration when bone grafts and implants are placed.
(a) Bone cells maintain viability as a result of the rich arterial supply, with smaller vessels reaching the cells embedded within the bone matrix.
(b) The vascular system within the lamellar bone emerges from the medullary spaces; the interconnected design irrigates the entire structure.
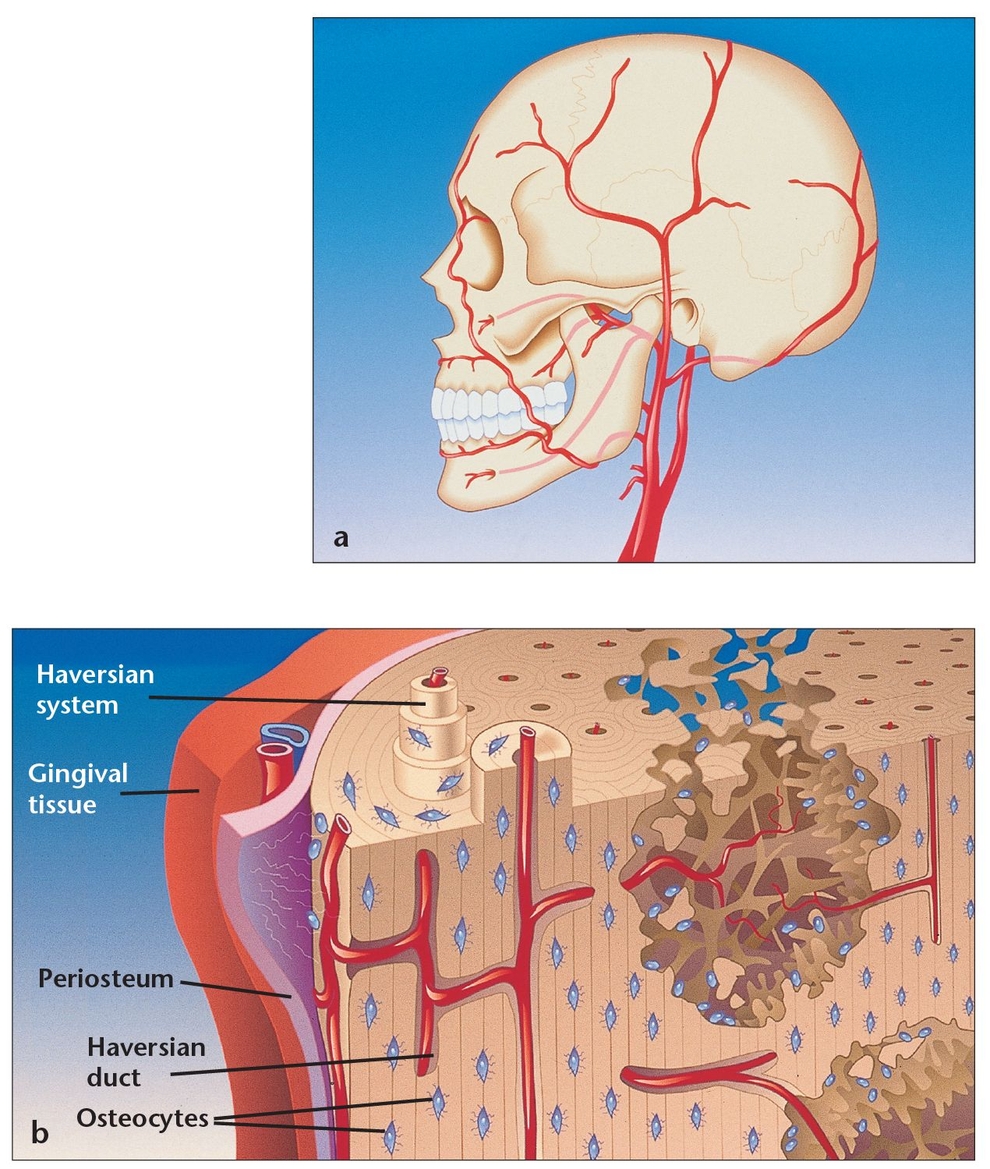
Bone, a mass-efficient tissue, has a trabecular configuration that provides resilience under functional load.
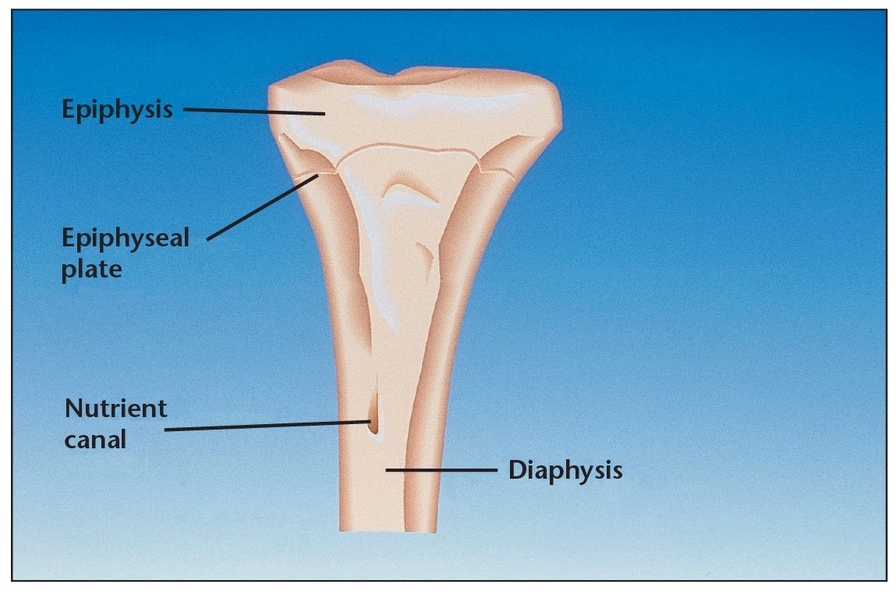
Bone Cells
Three main types of cells are involved in bone metabolism and physiology: osteoblasts, osteocytes, and osteoclasts.
Osteoblasts, which are involved in building bone, are located in two general areas. These cells deposit bone matrix (Fig 1-4) and are frequently referred to as either endosteal osteoblasts or periosteal osteoblasts . Periosteal osteoblasts are present on the outer surfaces of the bones beneath the periosteum, while endosteal osteoblasts line the vascular canals within bone. Mature osteoblasts are responsible for producing the proteins of bone matrix. Indeed, the cytoplasm of osteoblasts is intensely basophilic, suggesting the presence of ribonucleoproteins that are related to the synthesis of these protein components. Bone deposition continues in an active growth area for several months, with osteoblasts laying down new bone in successive layers of concentric circles on the inner surfaces of the cavity in which they are working. This activity continues until the tunnel is filled with new bone to the point that the new growth begins to encroach on the blood vessels running through it. In addition to mineralizing newly formed bone matrix, osteoblasts also produce other matrix constituents, such as phospholipids and proteoglycans, that may also be important in the mineralization process. During osteogenesis, the osteoblasts secrete growth factors, including transforming growth factor-beta (TGF-β), BMPs, platelet-derived growth factor (PDGF), and insulin-like growth factors (IGFs), which are stored in bone matrix.4 Recent research suggests that osteoblasts may even act as helper cells for osteoclasts during normal bone resorption, possibly by preparing the bone surface for their attack. 4 However, further study is needed to clarify this possible role.
When osteoblasts have successfully formed bone matrix and then become embedded in it, they transform into osteocytes (see Fig 1-1b). Osteocytes are the most abundant bone cells, and they communicate with each other and with cells on the bone surface via dendritic processes encased in canaliculi. Osteocytes have a slightly basophilic cytoplasm, the prolongations of which extend from the osteocyte through a network of fine canaliculi that emerge from the lacunae. During bone formation, these prolongations extend beyond their normal limit, creating direct continuity with adjacent osteocyte lacunae and with the tissue spaces. Fluid in these spaces mixes with fluid from the canaliculi; this appears to allow an exchange of metabolic and biochemical messages between the bloodstream and osteocytes. In mature bone, there is almost no extension of these prolongations, but the canaliculi continue to function as a means of messenger exchange. This mechanism allows the osteocytes to remain alive, regardless of the calcified intercellular substance surrounding them. This duct system does not function, however, if it is located more than 0.5 mm from a capillary, which may explain the abundant blood supply in bone through capillaries that run through the Haversian systems and Volkmann canals (see below). Osteocytes have also been shown to express TGF-β and possibly other growth factors. Some researchers have also suggested that weight-bearing loads may influence the behavior of bone remodeling cells located on bone surfaces by their effects on the osteocytes buried within the bone, which subsequently release TGF-β into the canalicular system.4 Other research suggests that osteocytes may play a role in transporting calcium through the bones.5
Osteoclasts are the cells responsible for bone resorption, and their activity is controlled by parathyroid hormone.4 Osteo-clastsare fused monocytes that histologically appear as large, multinucleated giant cells (containing as many as 50 nuclei). They are located in shallow excavations (Howship lacunae) along the mineralized bone surfaces.6 A specific area of their cell membrane forms adjacent to the bone surface to be resorbed. This area, known as the ruffled border, is formed by villus-like projections that the osteoclasts send out toward the bone. It consists of folds and invaginations that allow intimate contact between the cell membrane and the bone surface (see Fig 1-1b). Bone resorption occurs in the ruffled border as the villi secrete proteolytic enzymes that digest or dissolve the organic bone matrix and acids that cause dissolution of the bone cells. Via phagocytosis, osteoclasts also absorb minute particles of bone matrix and crystals, eventually dissolving them and releasing the products into the bloodstream. In adults, osteoclasts are usually active on fewer than 1% of bone surfaces at any one time.7 They typically exist in small but concentrated masses. Once a mass has developed, it usually eats away at the bone for about 3 weeks, creating a tunnel that ranges from 0.2 to 1.0 mm in diameter and is several millimeters long. After local bone resorption is complete, the osteoclasts disappear, probably by degeneration. Subsequently, the tunnel is invaded by osteoblasts, and the bone formation segment of the continuous remodeling cycle begins again.
When influenced by the transforming growth factors secreted by platelets and osteoblasts, undifferentiated stem cells can transform into pre-osteoblasts, then osteoblasts, and eventually osteocytes, thus maturing into the tissues essential to the body.
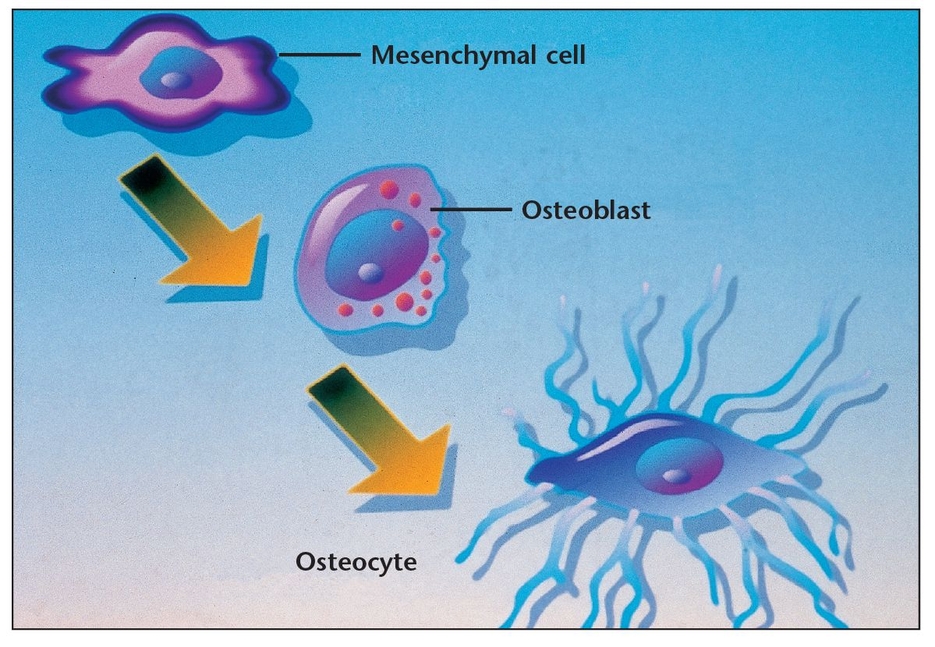
In addition to the three main types of bone cells, there is a fourth type, the bone-lining cell. These cells are similar to osteocytes in that they are “retired” osteoblasts—in other words, osteoblasts that do not become embedded in newly formed bone, but instead adhere to the outer bone surfaces when formation halts. Bone-lining cells become quiescent and flattened against the bone surface, but they do not form a contiguous gap-free barrier. They maintain communication with osteocytes and with each other via gap-junctioned processes, and they also appear to maintain their receptors for hormones such as parathyroid hormone and estrogens. As with osteocytes, bone-lining cells are thought to play a role in transferring mineral into and out of bone and in sensing mechanical strain.8 They may also initiate bone remodeling in response to various chemicals or mechanical stimuli.9
Bone Metabolism
Bone is the body’s primary reservoir of calcium. Its tremendous turnover capability allows it to respond to the body’s metabolic needs and to maintain a stable serum calcium level.1,2 Calcium has an essential life-support function. It works in conjunction with the lungs and kidneys to help maintain the body’s pH balance by producing additional phosphates and carbonates. It also assists in the conduction of nerve and muscle electrical charges, including those involving the heart (see Fig 1-1b).
Bone structure and mass—including that of the skull and jaw—are directly affected by the body’s metabolic state. Faced with unmet calcium requirements or certain diseases, the structural integrity of bone may be altered and even compromised. Consider the bone structure of postmenopausal women. In response to decreased estrogen hormone in the system, bone mass begins to dwindle, and the interconnections between bone trabeculae are lost. Because normal interconnections are crucial for making bone biomechanically rigid, the decrease in bone leads to an increase in fragility. This is an important phenomenon in dental implantology and related bone grafting because declining estrogen levels appear to significantly increase the risk of implant failure.10 The effects of a disrupted balance in bone remodeling are also illustrated by Albers-Schoenberg, or “marble bone,” disease, which involves defective osteoclasts. Because these osteoclasts do not resorb the existing bone matrix and liberate BMP, new bone is not formed, resulting in avascular and acellular bone (essentially, old bone) that is brittle and thus fractures easily and frequently becomes infected. Other diseases associated with bone remodeling abnormalities include cancer, primary hyperparathyroidism, and Paget disease. Although these disorders are common, in most cases little is known about what mechanisms are responsible for controlling normal bone remodeling or how it is coordinated and balanced.
Metabolic-hormonal interactions play a crucial role in maintaining bone structure. Most importantly, they help to maintain the coupled cycle of bone resorption and bone apposition through BMP. As previously mentioned, when osteoblasts form bone, they also secrete BMP into the mineral matrix. This acid-insoluble protein resides in the matrix until it is released during osteoclastic resorption. The acid insolubility is an evolutionary mechanism by which the pH of 1 created by osteoclasts is able to dissolve bone mineral without affecting BMP.11 Once released, BMP binds to the cell surface of undifferentiated mesenchymal stem cells, where it causes a membrane signal protein to become activated with high-energy phosphate bonds. This, in turn, affects the gene sequence in the nucleus, causing expression of osteoblast differentiation and stimulation of new bone production. A disturbance in this process maybe at the root of osteoporosis. Of current research interest is the therapeutic potential of applying BMPs directly to a healing site to induce bone formation. Some researchers suggest that in the future, this biologic material may replace or assist bone grafts in restorative therapy,12 an issue discussed in more detail in chapter 11.
Normally, about 0.7% of the human skeleton is resorbed and replaced by new healthy bone each day (see Figs 1-1b and 1-2b). Therefore, normal turnover of the entire skeleton occurs approximately every 142 days. With aging and metabolic disease states, there may be a reduction in the normal turnover process and thus an increase in the average age of functional bone. This raises the risk for fatigue damage of old bone, compromised bone healing, failed implant integration, and loss of implant osseointegration.13 Thus, it is important for dental clinicians to recognize that a compromised status must be considered before treatment planning because its effects may not be revealed until the clinician attempts to place implants or until the implants have been in place for some time.
Macroscopic Structure of Bone
The human skeleton is composed of two distinct kinds of bone based on porosity: dense cortical tissue and spongy cancellous tissue (Fig 1-5). In principle, the porosity of bone could vary continuously from 0% to 100%; however, most sites are either of very low or very high porosity. In most cases, both cortical and cancellous tissue is found at every bone site, but their quantity and distribution vary. The non-mineralized spaces within bone contain marrow, a tissue consisting of blood vessels, nerves, and various cell types. Marrow’s chief function is to generate the principal cells present in blood; it is also a highly osteogenic material that can stimulate bone formation if placed in an extracellular skeletal location, as with bone grafting in the dental area.
Cortical or compact bone, which comprises about 85% of total bone in the body,4 is found in the shafts of long bones and forms a shell around vertebral bodies and other spongy bones (Fig 1-6). This tissue is organized in bony cylinders consolidated around a central blood vessel, called a Haversian system. Haversian canals, which contain capillaries and nerves, are connected to each other and to the outside surfaces of the bone by short, transverse Volkmann canals.
Trabecular, or cancellous, bone, which comprises about 15% of the body’s total bone, is found in cuboidal and flat bones and in the ends of long bones. Its pores are interconnected and filled with marrow. The bone matrix is in the form of plates (called trabeculae) arranged in a varied fashion; sometimes, they appear to be organized into orthogonal arrays, but often they are randomly arranged.14 The medullary cavities are filled with marrow, which is red when there is active production of blood cells or a reserve population of mesenchymal stem cells and yellow when aging causes the cavity to be converted into a site for fat storage.
Exce/>
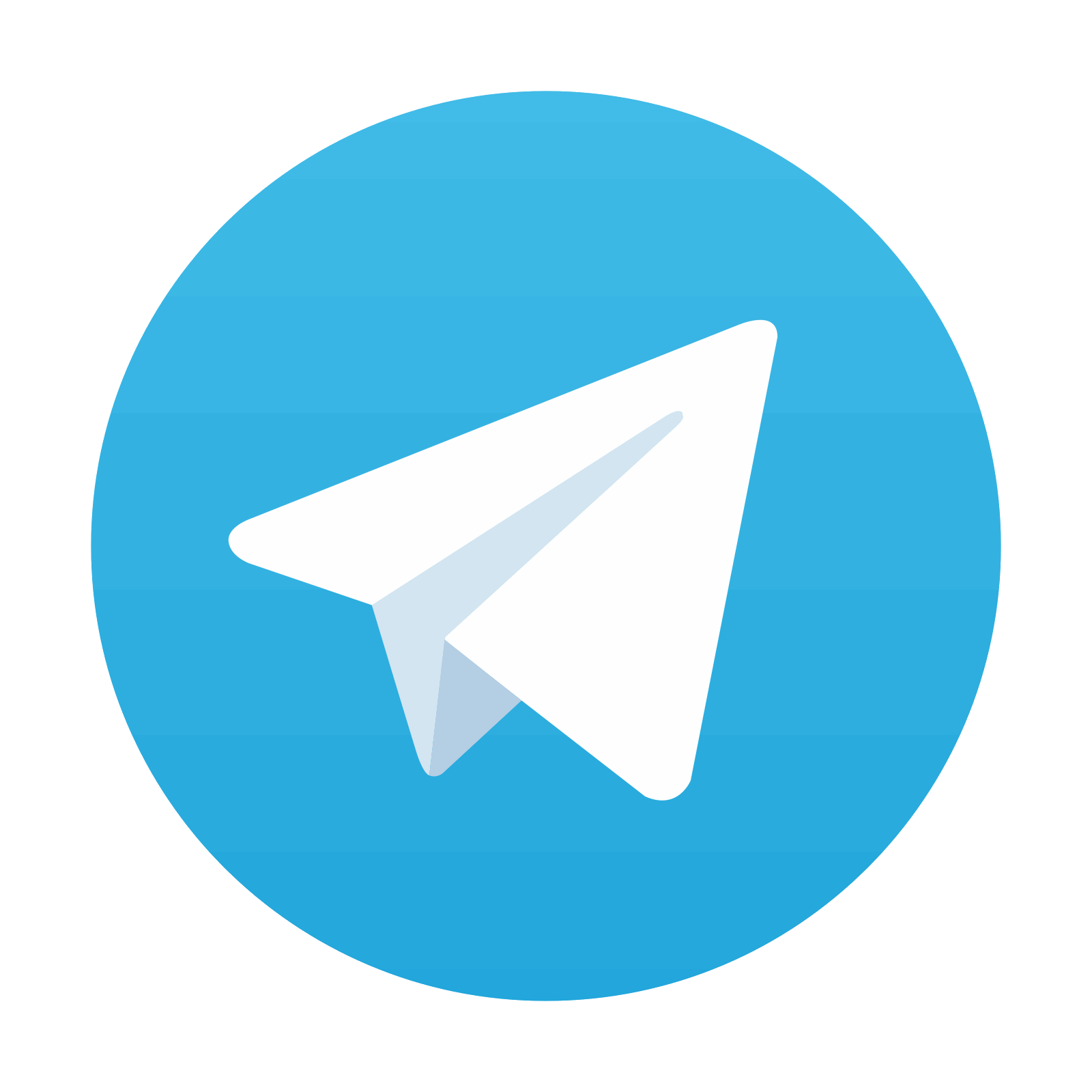
Stay updated, free dental videos. Join our Telegram channel

VIDEdental - Online dental courses
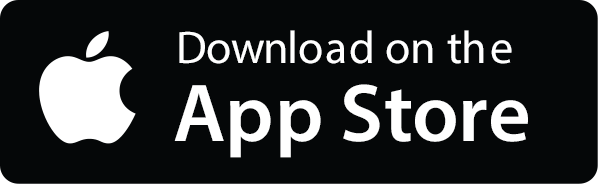
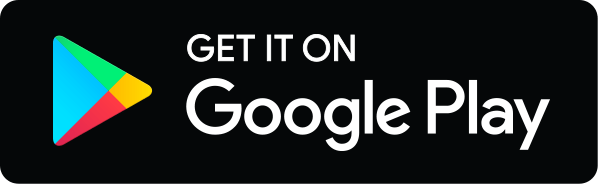