Learning Objectives
After reading this chapter, the student should be able to:
- 1.
Describe the development of pulp.
- 2.
Describe the process of root development.
- 3.
Recognize the anatomic regions of pulp.
- 4.
List all cell types in the pulp and describe their function.
- 5.
Describe both fibrous and non-fibrous components of the extracellular matrix of pulp.
- 6.
Describe the blood vessels and lymphatics of pulp.
- 7.
List the neural components of pulp and describe their distribution and function.
- 8.
Discuss theories of dentin sensitivity.
- 9.
Describe the pathway of efferent nerves from pulp to the central nervous system.
- 10.
Describe the changes in pulp morphology that occur with age.
- 11.
Describe the structure and function of the periradicular tissues.
The dental pulp is the loose connective tissue in the center of the tooth. The primary function of the pulp is to form and support the dentin that surrounds it and forms the bulk of the tooth. The pulp contains odontoblasts that not only form dentin, but also interact with dental epithelium early in tooth development to initiate the formation of enamel. The pulp remains vital throughout life and is able to respond to external stimuli. Both dentin and pulp contain nociceptive nerve fibers. Autonomic nerve fibers occur only in the pulp. When needed for repair, more dentin can be laid down and new odontoblasts differentiated.
The pulp is equipped with all the necessary peripheral components of the immune system and will react to foreign antigens, such as those presented by dental caries. Injury and foreign antigens lead to inflammation and pain. The good health of the pulp is important to the successful completion of restorative and prosthetic dental procedures. In restorative dentistry, for example, the size and shape of the pulp must be considered in determining cavity depth. The size and shape of the pulp depend on the tooth type (e.g., incisor, molar), the degree of tooth development related to the age of the patient, and any restorative procedures that may have been carried out on the tooth. When a tooth is injured, the stage of development the pulp influences the type of treatment rendered. Procedures routinely undertaken on a fully developed tooth are not always practicable for a tooth that is only partially developed, and special procedures are applied.
Because endodontics involves the diagnosis and treatment of diseases of the pulp and their sequelae, a knowledge of the biology of the pulp is essential for the development of an evidence-based treatment plan. This chapter presents an overview of the biology of the pulp and the periodontium as a fundamental component of the evidential base.
Learning Objectives
After reading this chapter, the student should be able to:
- 1.
Describe the development of pulp.
- 2.
Describe the process of root development.
- 3.
Recognize the anatomic regions of pulp.
- 4.
List all cell types in the pulp and describe their function.
- 5.
Describe both fibrous and non-fibrous components of the extracellular matrix of pulp.
- 6.
Describe the blood vessels and lymphatics of pulp.
- 7.
List the neural components of pulp and describe their distribution and function.
- 8.
Discuss theories of dentin sensitivity.
- 9.
Describe the pathway of efferent nerves from pulp to the central nervous system.
- 10.
Describe the changes in pulp morphology that occur with age.
- 11.
Describe the structure and function of the periradicular tissues.
The dental pulp is the loose connective tissue in the center of the tooth. The primary function of the pulp is to form and support the dentin that surrounds it and forms the bulk of the tooth. The pulp contains odontoblasts that not only form dentin, but also interact with dental epithelium early in tooth development to initiate the formation of enamel. The pulp remains vital throughout life and is able to respond to external stimuli. Both dentin and pulp contain nociceptive nerve fibers. Autonomic nerve fibers occur only in the pulp. When needed for repair, more dentin can be laid down and new odontoblasts differentiated.
The pulp is equipped with all the necessary peripheral components of the immune system and will react to foreign antigens, such as those presented by dental caries. Injury and foreign antigens lead to inflammation and pain. The good health of the pulp is important to the successful completion of restorative and prosthetic dental procedures. In restorative dentistry, for example, the size and shape of the pulp must be considered in determining cavity depth. The size and shape of the pulp depend on the tooth type (e.g., incisor, molar), the degree of tooth development related to the age of the patient, and any restorative procedures that may have been carried out on the tooth. When a tooth is injured, the stage of development the pulp influences the type of treatment rendered. Procedures routinely undertaken on a fully developed tooth are not always practicable for a tooth that is only partially developed, and special procedures are applied.
Because endodontics involves the diagnosis and treatment of diseases of the pulp and their sequelae, a knowledge of the biology of the pulp is essential for the development of an evidence-based treatment plan. This chapter presents an overview of the biology of the pulp and the periodontium as a fundamental component of the evidential base.
Development of the Dental Pulp
Early Development of Pulp
The tooth originates as a band of epithelial cells, the dental lamina ( Fig. 1.1, A ), on the surface of the embryonic jaws. Downgrowths from this band ultimately form the teeth. The stages of tooth formation are described by the shapes of these downgrowths. Initially, they look like the bud of a forming flower (bud stage, Fig. 1.1, B ). The bud becomes invaginated at the cap stage ( Fig. 1.1, C ). The invagination deepens, and the bell stage is reached ( Fig. 1.1, D ). The bell-shaped downgrowth is the enamel organ. It is ectodermal in origin and will be responsible for amelogenesis. The tissue within the invagination ultimately becomes the dental pulp, known as the dental papilla, during the early stages of development. Before that, the cells it contains differentiate. The papilla (and thus the pulp) is derived from cells that have migrated from the neural crest (ectomesenchymal cells) and mingled with cells of local mesenchymal origin.
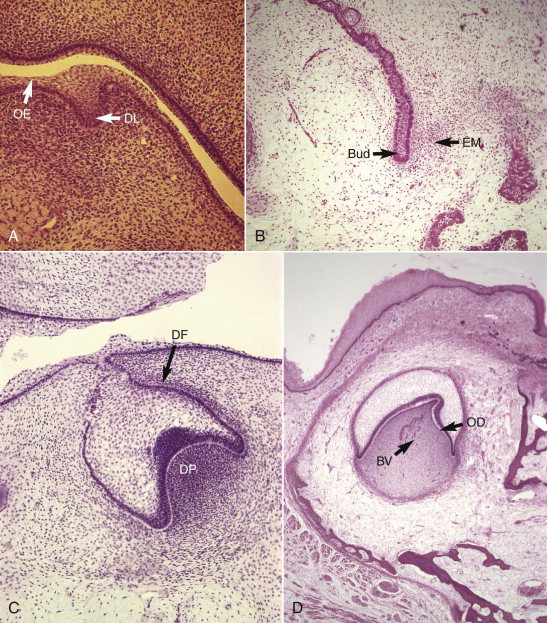
During the bell stage, the inner layer of cells of the enamel organ differentiate into ameloblasts ( Fig. 1.2, A ). This is followed by the outer layer of cells of the dental papilla differentiating into odontoblasts ( Fig. 1.2, B ), which begin to lay down dentin in the late bell (or crown) stage ( Fig. 1.2, C ). From this point on, the tissue within the invagination is known as the dental pulp. A layer of tissue begins to differentiate around the enamel organ and dental papilla and forms the dental follicle, which later becomes the periodontal attachment. The combination of the enamel organ dental papilla/pulp and dental follicle is the tooth germ.
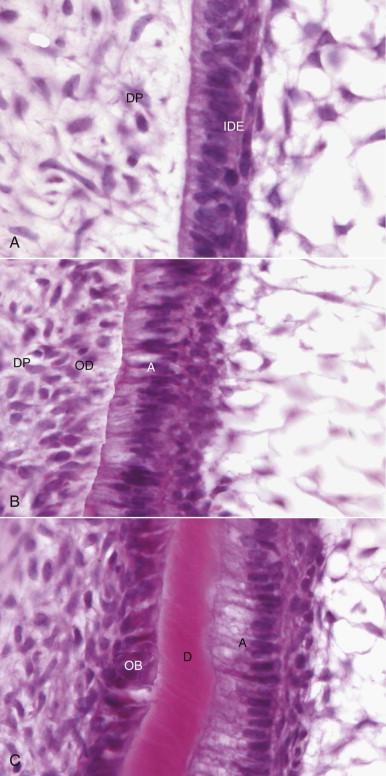
The histodifferentiation and morphodifferentiation of the tooth germ are genetically determined and executed by a group of growth factors, transcription factors, and other signaling molecules. Several of the genes controlling this process have been identified. Disorder at this stage can lead to anodontia, amelogenesis imperfecta, odontogenesis imperfecta, and related defects. A substantial research effort has been underway for some years, with a long-term goal of using these molecules therapeutically in procedures such as apexogenesis and pulpal regeneration.
The differentiation of odontoblasts from undifferentiated ectomesenchymal cells is initiated and controlled by the ectodermal cells of the inner dental epithelium of the enamel organ. The ameloblasts synthesize growth factors and signaling molecules that pass into the basal lamina of the epithelium and from there to the preodontoblast. The cells beneath the forming odontoblasts remain as undifferentiated stem cells and retain the potential to differentiate.
Once the odontoblast layer has differentiated, the basal lamina of the inner dental epithelium that contained the signaling molecules disappears, and the odontoblasts, now linked to each other by tight junctions, desmosomal junctions, and gap junctions, begin to lay down dentin (see Fig. 1.2, C ). Once dentin formation has begun, the cells of the inner dental epithelium begin responding to a signal from the odontoblasts and start to deposit enamel. This back-and-forth signal control is an example of epithelial-mesenchymal interaction, a key developmental process that has been heavily studied in the tooth germ model. Deposition of unmineralized dentin matrix begins at the cusp tip and progresses in a cervical (apical) direction in a regular rhythm at an average of 4.5 µm/day. Crown shape is genetically predetermined by the proliferative pattern of the cells of the inner dental epithelium. The first thin layer of dentin formed is called mantle dentin. The direction and size of the collagen fibers in mantle dentin, together with the mineralization pattern, differ from those in the subsequently formed circumpulpal dentin. Processes from the odontoblasts remain at least in the inner part of the dentinal tubules. A pattern of matrix formation followed by mineralization continues throughout dentin deposition. Between 10 and 50 µm of the dentin matrix immediately adjacent to the odontoblast layer remains unmineralized at all times and is known as predentin.
As crown formation occurs, nerves and blood vessels begin migrating into the pulp from the future root apex in a coronal direction. Both undergo branching and narrowing toward the odontoblast layer, and at a late stage, each forms plexuses beneath the layer with the nerves extending branches into the odontoblast layer and some of the dentinal tubules. Dentin formation continues throughout life in an incremental pattern marked by lines in the matrix and changes in direction of the tubules. The rate of deposition slows in adulthood but never completely stops. The rate can increase if the odontoblasts are stimulated by toxin molecules penetrating the dentin.
Root Formation
In the developing tooth, cells of the inner and outer dental epithelia meet at a point known as the cervical loop. This delineates the end of the anatomic crown and the site where root formation begins. Root formation is initiated by the apical proliferation of the two fused epithelia, now known as Hertwig’s epithelial root sheath. The function of the sheath is similar to that of the inner enamel epithelium during crown formation. It provides signals for the differentiation of odontoblasts and thus acts as a template for the root ( Fig. 1.3, A ). Cell proliferation in the root sheath is genetically determined; its pattern regulates whether the root will be wide or narrow, straight or curved, long or short, or single or multiple. Multiple roots result when opposing parts of the root sheath proliferate both horizontally and vertically. As horizontal segments of Hertwig’s epithelial root sheath join to form the “epithelial diaphragm,” the pattern for multiple root formation is laid down. This pattern is readily discernible when the developing root end is viewed microscopically ( Fig. 1.3, B ).
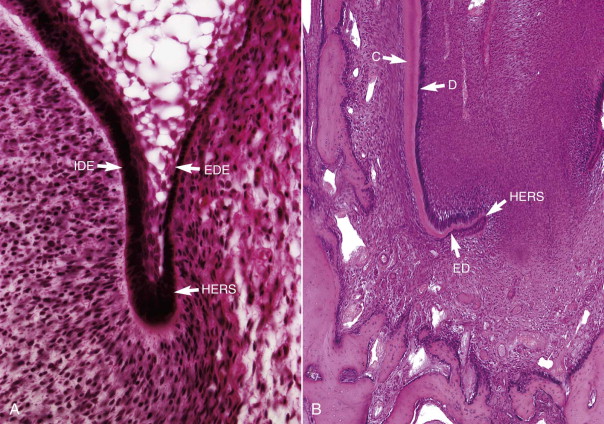
After the first dentin in the root has formed, the basement membrane beneath Hertwig’s sheath breaks up and the innermost root sheath cells secrete a hyaline material over the newly formed dentin. After mineralization has occurred, this becomes the hyaline layer of Hopewell-Smith, which helps bind the soon-to-be-formed cementum to dentin. Fragmentation of Hertwig’s epithelial root sheath occurs shortly afterward. This fragmentation allows cells of the surrounding dental follicle (the future periodontium) to migrate and contact the newly formed dentin surface, where they differentiate into cementoblasts and initiate acellular cementum formation ( Fig. 1.4 ). This cementum ultimately serves as an anchor for the developing principal fibers of the periodontal ligament (PDL). In many teeth, cell remnants of the root sheath persist in the periodontium in close proximity to the root after root development has been completed. These are the epithelial cell rests of Malassez. Normally functionless, in the presence of inflammation they can proliferate and may under certain conditions give rise to a radicular cyst.
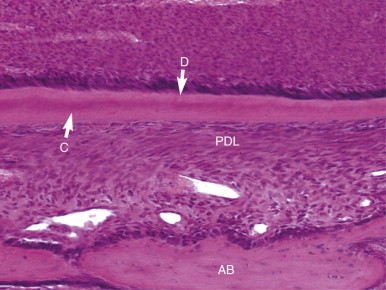
Formation of Lateral Canals and Apical Foramen
Lateral Canals
Lateral canals (or, synonymously, accessory canals) are channels of communication between pulp and PDL ( Fig. 1.5 ). They form when a localized area of root sheath is fragmented before dentin formation. The result is direct communication between pulp and the PDL via a channel through the dentin and cementum that carries small blood vessels and, perhaps, nerves. Lateral canals may be single or multiple, large or small. They may occur anywhere along the root but are most common in the apical third. In molars, they may join the pulp chamber PDL in the root furcation. Lateral canals are clinically significant; like the apical foramen, they represent pathways along which disease may extend from the pulp to periradicular tissues and from the periodontium to the pulp.
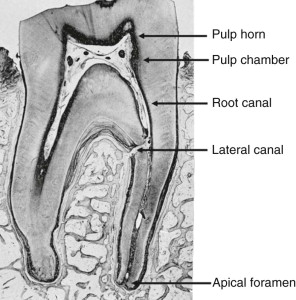
Apical Foramen
The epithelial root sheath continues to extend until the full, predetermined length of the root is reached. As the epithelial root sheath extends, it encloses more of the dental pulp until only an apical foramen remains, through which pulpal vessels and nerves pass. During root formation, the apical foramen is usually located at the end of the anatomic root. When tooth development has been completed, the apical foramen is smaller and can be found a short distance coronal to the anatomic end of the root. This distance increases later as more apical cementum is formed.
There may be one foramen or multiple foramina at the apex. Multiple foramina occur more often in multirooted teeth. When more than one foramen is present, the largest one is referred to as the apical foramen and the smaller ones as accessory canals. (Together they constitute the apical delta .) The diameter of the apical foramen in a mature tooth usually ranges between 0.3 and 0.6 mm. The largest diameters are found on the distal canal of mandibular molars and the palatal root of maxillary molars. Foramen size is unpredictable, however, and cannot be accurately determined clinically.
Formation of the Periodontium
Tissues of the periodontium develop from ectomesenchyme-derived tissue (dental follicle) that surrounds the developing tooth. After the mantle dentin has formed, enamel-like proteins are secreted into the space between the basement membrane and the newly formed collagen by the root sheath cells. This area is not mineralized with the mantle dentin but does mineralize later from the hyaline layer of Hopewell-Smith. After mineralization has occurred, the root sheath breaks down. This fragmentation allows cells from the follicle to proliferate and differentiate into cementoblasts, which lay down cementum over the hyaline layer. Bundles of collagen, produced by fibroblasts in the central region of the follicle (Sharpey’s fibers), are embedded in the forming cementum and will become the principal fibers of the PDL.
At the same time, cells in the outermost area of the follicle differentiate into osteoblasts to form the bundle bone that will also anchor the periodontal fibers. Later, periodontal fibroblasts produce more collagen that binds the anchored fragments together to form the principal periodontal fibers that suspend the tooth in its socket. Loose, fibrous connective tissue carrying nerves and blood vessels remains between the principal fibers. Undifferentiated mesenchymal cells (tissue-specific stem cells) are plentiful in the periodontium and possess the ability to form new cementoblasts, osteoblasts, or fibroblasts in response to specific stimuli. Cementum formed after the formation of the principal periodontal fibers is cellular and plays a lesser role in tooth support. As with the development of the dental pulp, these processes are genetically predetermined and executed via signaling molecules. There is intense research in this area, because it promises truly biologic approaches to periodontal disease.
The blood supply to the periodontium is derived from the surrounding bone, gingiva, and branches of the pulpal vessels. It is extensive and supports the high level of cellular activity in the area. The pattern of innervation is similar to that of the vasculature. The neural supply consists of small, unmyelinated sensory and autonomic nerves and larger myelinated sensory nerves. Some of the latter terminate as unmyelinated neural structures thought to be nociceptors and mechanoreceptors.
Anatomic Regions and Their Clinical Importance
The tooth has two principal anatomic divisions, root and crown, that join at the cervix (cervical region). The pulp space is similarly divided into coronal and radicular regions. In general, the shape and the size of the tooth surface reflect the shape and size of the pulp space. The coronal pulp is subdivided into the pulp horn(s) and pulp chamber (see Fig. 1.5 ). Pulp horns extend from the chamber into the cuspal region. In young teeth, they are extensive and may be inadvertently exposed during routine cavity preparation.
The pulp space becomes asymmetrically smaller after root growth is complete because of the slower production of dentin. There is a pronounced decrease in the height of the pulp horn and a reduction in the overall size of the pulp chamber. In molars, the apical-occlusal dimension is reduced more than the mesial-distal dimension. Excessive reduction of the size of the pulp space is clinically significant and can lead to difficulties in locating, cleaning, and shaping the root canal system ( Fig. 1.6 ).
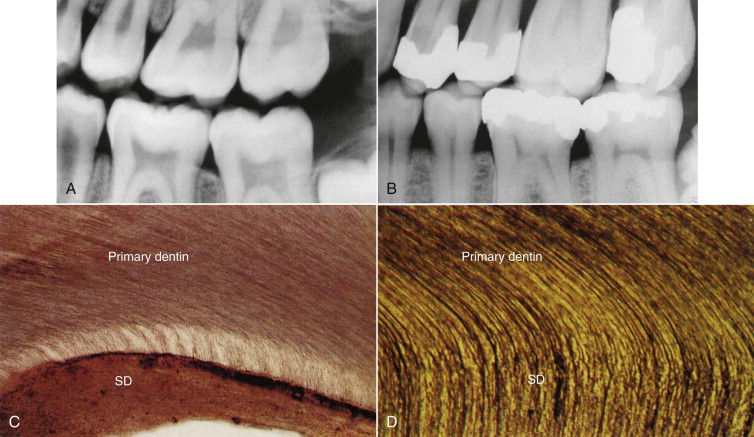
The anatomy of the root canal varies not only between tooth types, but also within tooth types. Although at least one canal must be present in each root, some roots have multiple canals of varying sizes. Understanding and appreciating all aspects of root canal anatomy are essential prerequisites to root canal treatment.
Variation in the size and location of the apical foramen influences the degree to which blood flow to the pulp may be compromised after a traumatic event . Young, partially developed teeth have a better prognosis for pulp survival than teeth with mature roots ( Fig. 1.7 ).
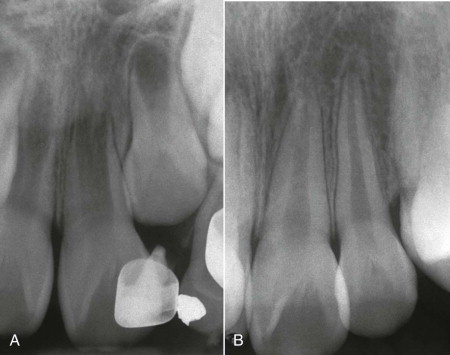
Posteruptive deposition of cementum in the region of the apical foramen creates a disparity between the radiographic apex and the apical foramen. It also creates a funnel-shaped opening to the foramen that is often larger in diameter than the intraradicular portion of the foramen. The narrowest portion of the canal is referred to as the apical constriction. However, a constriction is not clinically evident in all teeth. The constriction coincides with the cementodentinal junction (CDJ). The level of the CDJ varies from root to root. One study estimated the junction to be located 0.5 to 0.75 mm coronal to the apical opening. Theoretically, that is the point where the pulp terminates and the PDL begins, and it would be the ideal point for a procedure aimed at removing the pulp. However, clinically, it is not always possible to locate that point. Cleaning, shaping, and obturation of the root canal should terminate short of the apical foramen and remain confined to the canal to avoid unnecessary injury to the periapical tissues. The determination of root length and the establishment of a working length are essential steps in root canal preparation. Radiographs and electronic apex locators are helpful in establishing the root length.
Pulp Function
The pulp performs five functions, some formative and others supportive.
Induction
Pulp participates in the initiation and development of dentin. When dentin is formed, it leads to the formation of enamel. These events are interdependent, in that enamel epithelium induces the differentiation of odontoblasts, and odontoblasts and dentin induce the formation of enamel. Such epithelial-mesenchymal interactions are the core processes of tooth formation.
Formation
Odontoblasts form dentin. These highly specialized cells participate in dentin formation in three ways: (1) by synthesizing and secreting inorganic matrix, (2) by initially transporting inorganic components to newly formed matrix, and (3) by creating an environment that permits mineralization of the matrix. During early tooth development, primary dentinogenesis is a rapid process. After tooth maturation, when elongation of the root is complete, dentin formation continues at a much slower rate and in a less symmetric pattern (secondary dentinogenesis). Odontoblasts can also form dentin in response to injury, which may occur in association with caries, trauma, or restorative procedures. In general, this dentin is less organized than primary and secondary dentin and mostly localized to the site of injury. This dentin is referred to as tertiary dentin . Tertiary dentin has two forms. Reactionary tertiary dentin is tubular, with the tubules continuous with those of the original dentin. It is formed by the original odontoblasts. Reparative dentin is formed by new odontoblasts differentiated from stem cells after the original odontoblasts have been killed. It is largely atubular ( Fig. 1.8 ).
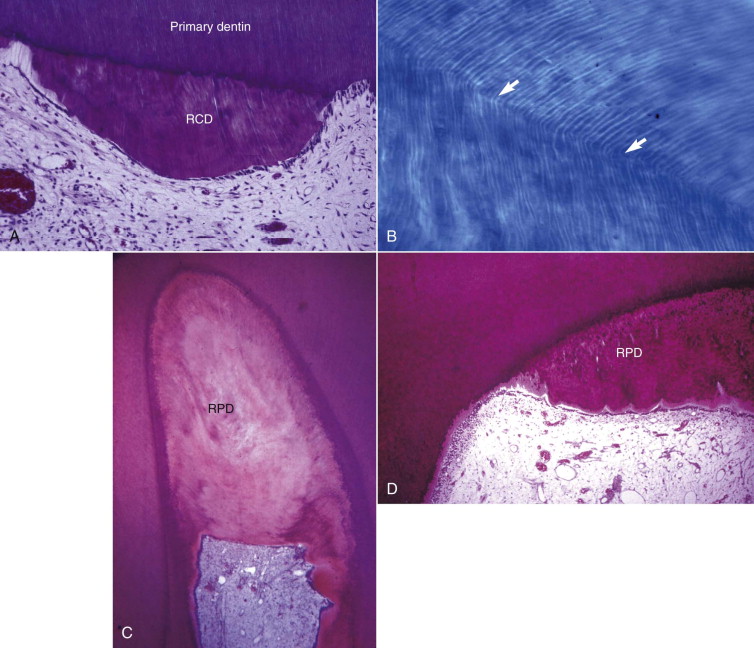
Nutrition
The pulp supplies nutrients that are essential for dentin formation and for maintaining the integrity of the pulp itself.
Defense
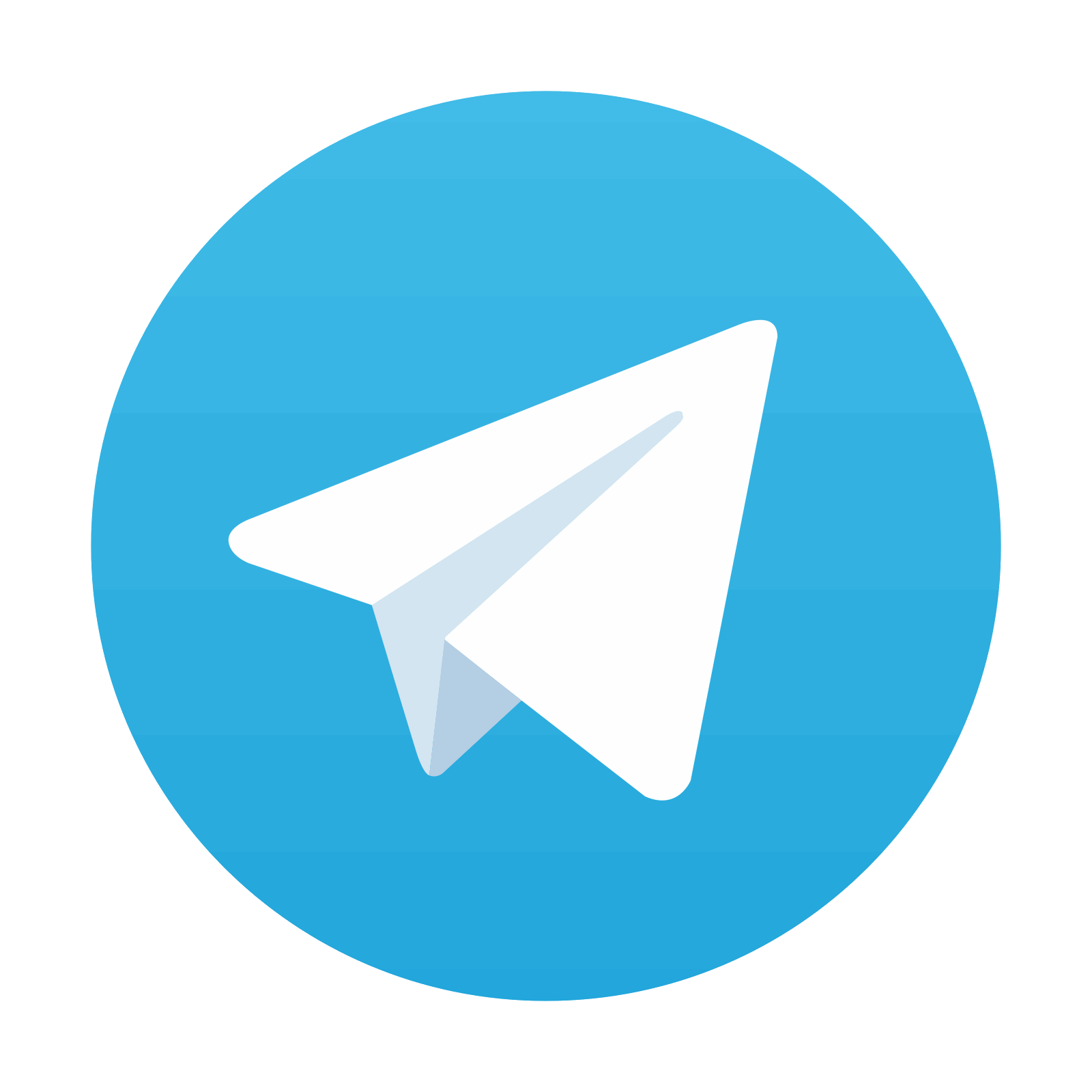
Stay updated, free dental videos. Join our Telegram channel

VIDEdental - Online dental courses
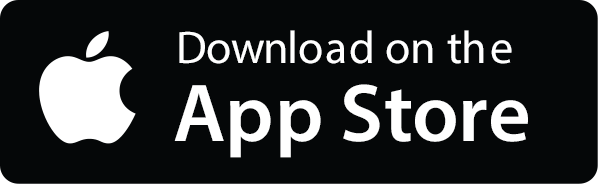
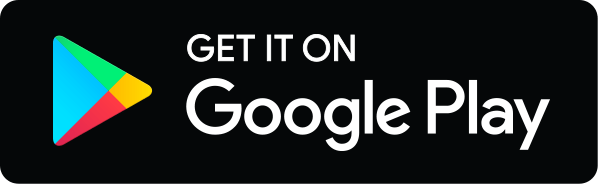