Carbohydrate metabolism
Publisher Summary
This chapter discusses carbohydrate metabolism. Carbohydrates occupy an important place in metabolism because of their roles in energy production and various biosynthetic pathways. Starch is the main source of energy in most populations; before it can be absorbed, it must be broken down. The hydrolysis of starch is catalyzed by enzymes known as amylases, which occur in the saliva and the pancreatic juice. The amylases of animal origin are called α-amylases to distinguish them from the β-amylases of plants, which differ in their point of attack on the starch molecule. The β-amylases are exoamylases that nip off maltose units from the ends of the amylose and amylopectin molecules, whereas the α-amylases are endoamylases and catalyze the breakdown of bonds occurring in the interior of the molecules. The first products of the action of the α-amylases are the dextrins, which are progressively degraded into smaller and smaller units. The final product consists mainly of maltose together with some small branched-chain oligosaccharides. A separate enzyme produced in the intestine is required to hydrolyze the α-1:6 bonds of amylopectin. The dietary carbohydrates also include sucrose and lactose. Specific disaccharides that convert these sugars into their constituent monosaccharides are present in the brush border of the intestinal epithelial cells.
Carbohydrates occupy an important place in metabolism because of their roles in energy production and various biosynthetic pathways. Starch is the main source of energy in most populations (page 122) and before it can be absorbed it must be broken down. The hydrolysis of starch is catalysed by enzymes known as amylases which occur in the saliva and the pancreatic juice. The amylases of animal origin are called α-amylases in order to distinguish them from the β-amylases of plants, which differ in their point of attack on the starch molecule. The β-amylases are exoamylases which nip off maltose units from the ends of the amylose and amylopectin molecules, whereas the α-amylases are endoamylases and catalyse the breakdown of bonds occurring in the interior of the molecules. The first products of the action of the α-amylases are the dextrins (page 98) which are progressively degraded into smaller and smaller units. The final product consists mainly of maltose together with some small branched-chain oligosaccharides. A separate enzyme produced in the intestine is required to hydrolyse the α-1:6 bonds of amylopectin.
< ?xml:namespace prefix = "mml" />
are absorbed even against a concentration gradient. The active transport of such sugars into the mucosal cells is linked with the transport of Na+ in the same direction. Other monosaccharides including fructose and the pentoses are absorbed by passive means. The monosaccharides pass into the portal system and are transported to the liver, where they are converted into compounds that are normal intermediates of glucose metabolism.
Glucose breakdown
The glycolytic or Embden-Meyerhof pathway
Reaction 3
The fructose 6-phosphate is then converted into fructose 1,6-bisphosphate by a second phosphorylation with ATP which is catalysed by phosphofructokinase. Phosphofructokinase is an allosteric enzyme and the reaction, which is irreversible, is an important control point, i.e. rate-limiting step (page 337), for the glycolytic pathway.
Reaction 4
Because of the presence of triose phosphate isomerase the glyceraldehyde 3-phosphate and dihydroxyacetone phosphate are interconvertible. Glyceraldehyde 3-phosphate is on the direct pathway of glycolysis (Figure 17.1) and is continuously removed with the result that dihydroxyacetone phosphate is continuously converted into glyceraldehyde 3-phosphate. Not all the dihydroxyacetone phosphate is used in this way; some is converted to α-glycerophosphate which takes place in the α-glycerophosphate shuttle (page 230) and some may be used in the synthesis of triglycerides. However, when considering the oxidation of glucose it may be assumed that each molecule will give rise to two molecules of glyceraldehyde 3-phosphate which will both participate in the subsequent reactions.
Energy production and the importance of glycolysis

Pyruvate is an important compound. After entering the mitochondria it may be converted to acetyl-CoA (page 232) which is then oxidized to carbon dioxide and water by the joint activities of the citrate cycle and electron-transport chain with the production of much greater quantities of ATP than are produced by glycolysis alone. It also has a role in amino acid synthesis as it may be transaminated to form alanine (page 281). Pyruvate is also an important intermediate in gluconeogenesis the process whereby glucose is synthesized from non-carbohydrate sources (page 237). In bacteria a variety of end products may be formed from pyruvate depending both on the nature of the bacterium and the environmental conditions. When oxygen is in short supply, muscle tissue converts pyruvate to lactate while yeasts ferment it to ethanol.
Aerobic and anaerobic glycolysis
During the oxidation of glyceraldehyde 3-phosphate to 1,3-diphosphoglycerate, which is the only oxidative step in the glycolytic pathway, NAD+ is converted into its reduced form (NADH) and, unless there is a continuous supply of NAD+, the oxidation of glyceraldehyde 3-phosphate will cease. Glycolysis takes place in the cytoplasm and it is here that NADH must be oxidized to NAD+. However, the enzymes specifically responsible for this reaction are constituents of the electron-transport chain and are localized in the mitochondria. A simple solution would appear to be that NADH should pass into the mitochondria and, after oxidation to NAD+ should return to the cytoplasm. The mitochondrial membrane is not, however, readily permeable to NADH and NAD+; this complication is overcome by oxidizing NADH to NAD+ at the expense of dihydroxyacetone phosphate which is reduced to glycerol 3-phosphate. The enzyme catalysing the reaction is glycerol 3-phosphate dehydrogenase. The glycerol 3-phosphate then passes into the mitochondria where it is reoxidized to dihydroxyacetone phosphate. The dehydrogenase which catalyses the conversion of glycerol 3-phosphate to dihydroxyacetone phosphate in the mitochondria is different from the NAD+-linked glycerol 3-phosphate dehydrogenase present in the cytosol. It is linked to FAD instead of NAD+ and transfers electrons to the respiratory chain at the level of coenzyme Q (page 216). Consequently only two molecules of ATP, instead of three, are formed when cytoplasmic NADH is oxidized in this way. The dihydroxyacetone phosphate returns from the mitochondria to the cytoplasm where it is once again available to participate in the oxidation of NADH, thus permitting the oxidative step in aerobic glycolysis to proceed. This is known as the glycerophosphate shuttle.
Anaerobic glycolysis in muscle

Quite large amounts of lactate may be produced in muscle as a result of anaerobic glycolysis and its fate is considered later (page 239). It is mainly derived from muscle glycogen since, although when resting the muscles obtain their energy by metabolizing glucose and fatty acids obtained directly from the blood, during exercise the stored muscle glycogen is called upon. This has the advantage that, at a time when ATP is in short supply, one molecule is ‘saved’ for every glucosyl residue of glycogen used instead of glucose itself. This is because the glucosyl residues are in a higher energy state than free glucose and are phosphorylated by a low-energy phosphoryl donor, i.e. inorganic phosphate (page 234) rather than ATP, which is needed for the phosphorylation of glucose.
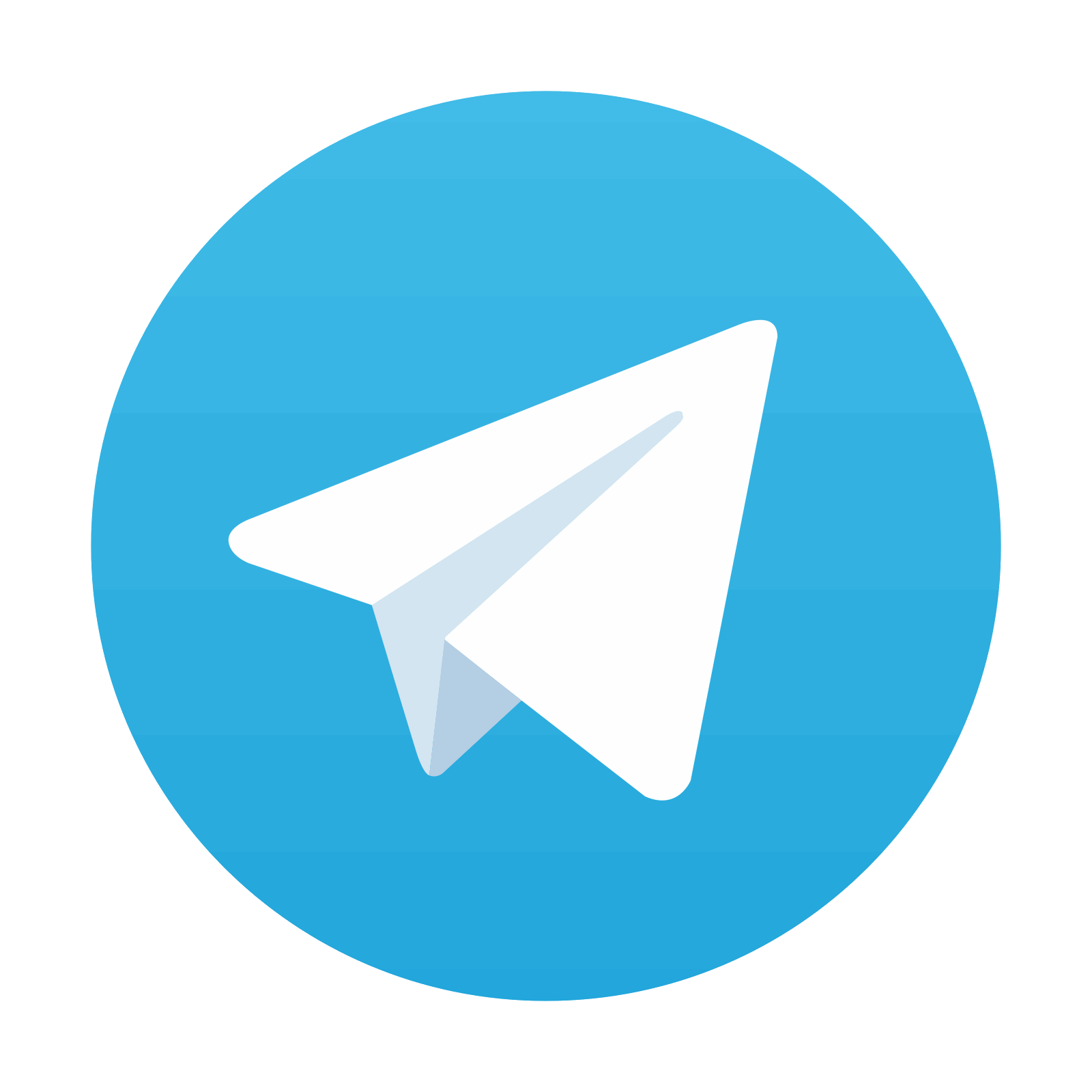
Stay updated, free dental videos. Join our Telegram channel

VIDEdental - Online dental courses
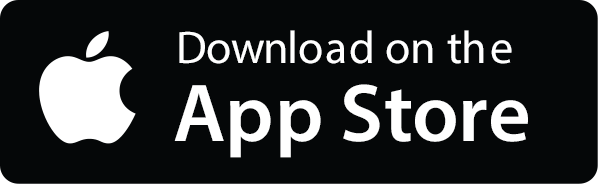
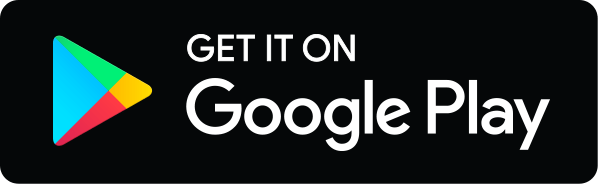